Drug
Route
Dosing
Indication
Amphotericin B
Topical
1.5–5 mg/ml
First choice in keratitis by yeasts
Intrastromal
5–10 μg/0.1 ml
Deep keratitis with partial response to topical treatment
Intracameral
5–10 μg/0.1 ml
Keratitis affecting anterior chamber/lens
Intravitreal
1–10 μg/0.1 ml
First choice in fungal endophthalmitis (yeast/filamentous fungi)
Natamycin
Topical
50 mg/ml
First choice in filamentous fungi
Miconazole
Subconjunctival
1.2–10 mg/1 ml
Associated with topical therapy in low adherence to treatment
Econazole
Topical
20 mg/ml
Alternative to NTM for filamentous fungi
Ketoconazole
Oral
100–400 mg every 12 h
Deep keratitis along with topical therapy
Itraconazole
Oral
400 mg/day
Deep keratitis by yeasts
Fluconazole
Topical
2 mg/ml
Alternative to polyenes in Candida keratitis
Subconjunctival
2 mg/1 ml
Associated with topical therapy in low adherence to treatment
Oral
200–400 mg/day
Deep keratitis
Voriconazole
Topical
1 mg/ml
Fungal keratitis resistant to polyenes and first-line triazoles
Intrastromal
50 μg/0.1 ml
Deep keratitis with partial response to topical therapy
Intracameral
50 μg/0.1 ml
Deep keratitis affecting anterior chamber/lens
Intravitreal
50 μg/0.1 ml
Alternative to AMB in fungal endophthalmitis
Oral
200 mg every 12 h
Deep keratitis
Posaconazole
Topical
100 mg/ml
Fungal keratitis resistant to polyenes or first-line triazoles
Oral
200 mg every 6 h or 400 mg every 12 h
Deep keratitis or endophthalmitis
Flucytosine
Topical
10 mg/ml
Association with topical AMB in Candida keratitis
Caspofungin
Topical
1.5–5 mg/ml
Fungal keratitis by yeasts resistant to polyenes or first-line triazoles
Micafungin
Topical
1 mg/ml
Fungal keratitis by yeasts resistant to polyenes or first-line triazoles
11.1.6 Conclusion
To conclude, a variety of antifungal drugs are available in the market for topical and systemic use, the choice of which depends upon the causative organism, the location, and the extent of infection. Standard therapy with polyenes remains the first choice of treatment for fungal keratitis. New-generation triazoles act as an add-on therapy in cases of poor responsiveness to the former. Studies until date lack the demonstration of superiority of the newer-generation triazoles over the conventional therapy with polyenes.
11.2 Antiviral Drugs for Ocular Use
Neelima Aron3, Tushar Agarwal3 and Neelima Sharma3
(3)
Cornea Services, Dr. Rajendra Prasad Center for Ophthalmic Sciences, All India Institute of Medical Sciences, New Delhi, India
Viruses are intracellular microorganisms which replicate inside the host cell. The development of antiviral therapy involves the identification of drugs which only attack the virus without affecting the host cells. The recognition of viral enzymes and proteins that can serve as molecular targets for drugs has revolutionized the treatment of viral infections.
Topical antiviral therapy has been available since the past 50 years, but it is only with the advent of acyclovir that a safe and effective therapy has been established for the treatment of herpetic keratitis.
Table 11.1 enumerates the various topical antiviral drugs that are available in the market as of today.
Drug | Preparation | Dosage |
---|---|---|
Trifluridine | 1 % solution | Initially, 1 drop every 2 h. On healing, treatment should be continued for 7 days at a dose of 1 drop every 4 h during day time |
Vidarabine | 3 % ointment | Applied daily at 4-h intervals. On healing, treatment should be continued for 7 days at a twice daily dose |
Idoxuridine | 0.1 % solution | One drop every 2 h during the day and every 4 h at night. Treatment should be continued for 3–5 days after healing is complete |
0.5 % ointment | Applied every 4 h during daytime. Treatment should be continued for 3–5 days on healing | |
Acyclovir | 3 % ointment | Applied five times daily at 3–4-h intervals and continued for at least 3 days after complete healing |
Ganciclovir | 0.15 % ointment | Applied five times a day at 3–4-h intervals and continued for at least 3 days after epithelial healing |
11.2.1 Idoxuridine
It was the first topical antiviral to be used for the treatment of herpetic epithelial keratitis (Kaufman et al. 1962a, b). However, it was later replaced by its thymidine analogue, trifluridine.
Mechanism of Action
IDU owes its antiviral activity to the conversion into a triphosphate form, which mimics thymidine triphosphate and becomes incorporated into viral DNA which results in faulty transcription of viral proteins and inhibition of viral replication.
Indication
IDU has been used in the treatment of herpetic epithelial keratitis. Owing to its lower intraocular penetration, it is ineffective in the treatment of herpetic stromal keratitis or uveitis. It is formulated as a 0.1 % solution and a 0.5 % ointment. The recommended regimen is one hourly during the day and 2 hourly at night and tapered once healing starts to take place (Kaufman et al. 1962a, b).
IDU has been replaced by trifluridine, and it is no longer commercially available for the treatment of viral keratitis.
Toxicity
IDU gets incorporated into the mammalian DNA along with viral DNA and hence is toxic to replication of normal host cells. This results in a low therapeutic ratio and accounts for systemic toxicity (Boston Inter-Hospital Viral Study Group 1975). Its use has been associated with chronic follicular conjunctivitis, conjunctival scarring, punctate keratopathy, pseudodendrites, corneal edema and opacities, indolent ulceration, punctal and canalicular stenosis, narrowing of meibomian gland orifices, and contact dermatitis of the lids (Wilson 1979).
11.2.2 Trifluridine
Trifluridine, like IDU, is a thymidine analogue which is far more potent and has less ocular and systemic toxicity.
Mechanism of Action
Trifluridine, like IDU, gets converted into a triphosphate form and gets incorporated into the viral DNA leading to inhibition of transcription and viral protein synthesis. Though it also gets incorporated into the host DNA, however, viral DNA polymerase utilizes trifluridine triphosphate more efficiently than does host cell DNA polymerase. Hence, it has a more selective antiviral activity with lower ocular toxicity as compared to IDU (Prusoff et al. 1985).
Indication
Trifluridine is active in vitro and in vivo against HSV-1, HSV-2 (Kaufman and Heidelberger 1964), and vaccinia and in vitro against CMV and some strains of adenovirus. It is more potent than IDU against HSV. It is available as a 1 % solution. Though its penetration is better than IDU, however, it is not very efficient in iridocyclitis or stromal keratitis. The recommended dosage is one drop every 2 h until healing is complete. This is followed by one drop every 4 h for 7 days to prevent reactivation of disease (Coster et al. 1976).
In a study, it was found that trifluridine was at least as effective as topical vidarabine ointment in the treatment of superficial herpetic dendritic keratitis with insignificant difference in the efficacy of the two drugs (Travers and Patterson 1978).
Toxicity
Toxic side effects in the eye are similar to those of IDU and include punctate keratopathy, filamentary keratopathy, epithelial and stromal edema, punctal narrowing, and contact blepharodermatitis. It is too toxic for systemic use (Wilson 1979).
11.2.3 Vidarabine
Vidarabine was the second agent approved for the topical treatment of herpetic epithelial keratitis. It was also the first antiviral agent approved for systemic use; however, recently it has been replaced with acyclovir.
Mechanism of Action
Vidarabine is obtained from fermentation cultures of Streptomyces antibioticus (Lee et al. 1960). It is a purine nucleoside analogue that resembles deoxyadenosine. It gets converted into its triphosphate form which gets incorporated into the viral DNA. Unlike IDU, trifluridine, or acyclovir, vidarabine does not require viral thymidine kinase for its phosphorylation. Therefore, it might be expected to have high activity against thymidine kinase-deficient mutants of HSV (Larder and Darby 1986).
Indication
It is highly effective in the treatment of herpetic epithelial keratitis. In a study comparing trifluridine and vidarabine in 66 patients with herpetic dendritic keratitis, no difference was noted in the antiviral activity of the two drugs (Van Bijsterveld and Post 1980). A meta-analysis concluded that vidarabine was superior to IDU and equivalent to topical acyclovir and trifluridine in relative efficacy for dendritic epithelial keratitis (Wilhelmus 2000). Similar to IDU and trifluridine, it is not effective in herpetic stromal keratitis and uveitis. It is available as a 3 % ophthalmic ointment to be applied 5 times daily. Therapy should not be continued for more than 21 days.
Toxicity
It is less toxic than IDU to the regenerating corneal epithelium. Clinically, the ocular toxicity is similar to IDU. The major systemic side effects include gastrointestinal upset, elevation in liver enzymes (Nicholson 1984), and neurotoxicity (Sacks et al. 1982) when administered in patients with renal dysfunction.
11.2.4 Acyclovir
The development of acyclovir revolutionized the treatment of herpetic keratitis with its better efficacy and safety profile.
Mechanism of Action
It is an acyclic analogue of guanosine which is activated by viral thymidine kinase and becomes a potent inhibitor of viral DNA polymerase. It gets converted into the triphosphate form and is found in HSV-infected cells in a concentration which is 40–100 times higher than uninfected cells (Elion 1982). It has a greater affinity of viral DNA polymerase as compared to cellular DNA polymerase.
Indications
The drug inhibits HSV-1 and HSV-2, VZV, Epstein-Barr virus (EBV), human herpesvirus 6 (HHV-6), and CMV (Wagstaff et al. 1994). It can be administered topically, orally, and intravenously. Topical acyclovir 3 % ophthalmic ointment has the best corneal penetration of any topical antiviral drug. It penetrates intact corneal epithelium to achieve aqueous levels well within the therapeutic range for HSV-1 and HSV-2. Hence, it is effective in the treatment of herpetic stromal keratitis and iridocyclitis.
Significant intraocular levels of the drug are also present after oral and intravenous administration. The oral dose is 400 mg five times a day (5 mg/kg body weight) for HSV and 800 mg five times a day (10 mg/kg body weight) for VZV (Biron and Elion 1980).
Prophylactic antiviral therapy is indicated in patients post-keratoplasty to prevent reactivation of the virus while patients are on topical corticosteroids. The drug is given in a dose of 400 mg twice a day for 1 year.
The Herpetic Eye Disease Studies were carried out in order to determine the role of acyclovir in the treatment of herpetic keratitis. HEDS I and HEDS II consisted of three randomized, placebo-controlled trials. It was found that compared with placebo, corticosteroid therapy reduced the risk of persistent or progressive stromal keratouveitis by 68 % with faster resolution of the ulcer. It determined that there is no clinical benefit of adjunctive oral acyclovir for treating HSV stromal keratitis in patients receiving concomitant topical corticosteroids and trifluridine (Barron et al. 1994). For the treatment of HSV iridocyclitis, there was a strong suggestion of clinical benefit from the addition of oral acyclovir 400 mg 5 times daily as an adjunct to topical corticosteroids and trifluridine (50 % treatment failures with acyclovir vs. 68 % with placebo) (the Herpetic Eye Disease Study Group, 1996). The addition of a 3-week course of oral acyclovir to topical trifluridine treatment of acute HSV epithelial keratitis did not prevent the subsequent development of stromal keratitis or iritis over the following year (the Epithelial Keratitis Trial, 1997). There is, however, a clear-cut benefit from long-term suppressive oral acyclovir in preventing recurrent HSV epithelial keratitis and stromal keratitis. The cumulative recurrence rate of any ocular HSV was significantly reduced from 32 to 19 % by acyclovir (Herpetic Eye Disease Study Group, 1998).
Toxicity
It has not been shown to have a detrimental effect on the regenerating corneal epithelium. Superficial punctate keratopathy has been noted; however, the frequency is less than that seen with IDU. Other less common side effects include burning or stinging, tearing, follicular conjunctivitis, palpebral allergy, and punctal stenosis.
Oral acyclovir causes gastrointestinal side effects such as nausea, vomiting, and diarrhea. Liver function tests should be monitored every 2 weeks. Intravenous infusion of acyclovir can cause renal shutdown especially in patients with preexisting renal dysfunction.
11.2.5 Resistance
There are three mechanisms by which the virus can become resistant to acyclovir therapy. The most common mutation is loss of synthesis of viral thymidine kinase so that acyclovir is not phosphorylated to its active form (Wagstaff et al. 1994). A second type of mutation induces thymidine kinase with altered substrate specificity that phosphorylates thymidine but not acyclovir. Finally, a mutation of the viral DNA polymerase gene induces altered DNA polymerase that is not sensitive to inhibition by acyclovir triphosphate.
11.2.6 Ganciclovir
Ganciclovir is another antiviral agent that has been developed recently for ophthalmic use. It was the first drug to be approved by the Food and Drug Administration (FDA) for use in the treatment of CMV retinitis in immunocompromised patients.
Mechanism of Action
Ganciclovir is a nucleoside analogue that is selectively phosphorylated by virus-encoded thymidine kinase and is subsequently phosphorylated by cellular enzymes. Similar to acyclovir, it is converted into ganciclovir triphosphate which inhibits herpesvirus DNA polymerase and arrests HSV replication (Davies et al. 1987).
Indication
Following topical application of ganciclovir, it has been shown that the drug can penetrate the corneal stroma and can reach the aqueous humor in therapeutic levels. Corneal penetration of ganciclovir was due to the small size of the ganciclovir molecule, its high lipophilicity, and its high cellular affinity. It is available as an ophthalmic gel in a concentration of 0.15 % five times daily. Compared to acyclovir 0.3 % ointment, ganciclovir 0.15 % gel has been shown to be better tolerated and no less effective in several phase II and III trials. Randomized multicenter clinical trials demonstrated that ganciclovir ophthalmic gel 0.15 % is as effective as acyclovir in the treatment of acute epithelial herpetic keratitis. Also, ganciclovir is available in a gel form which induces lower blurring following its ophthalmic administration as compared to acyclovir which is available in an ointment form.
Ganciclovir 0.15 % gel may also help in the prevention of recurrences of herpetic keratitis in patients undergoing corneal transplantation. It is given in a dose of four times a day along with topical corticosteroids.
Toxicity
The most frequent adverse effects clinically have been hematological, primarily neutropenia (40 % of patients) and thrombocytopenia (20 %) after systemic administration of the drug. No ocular toxicity has been noted after ophthalmic use.
11.2.7 Other Drugs
11.2.7.1 Valacyclovir
Valacyclovir, the L-valyl ester of acyclovir, is a prodrug that is rapidly and nearly completely converted to acyclovir after oral administration. Its excellent bioavailability results in serum acyclovir levels comparable to intravenous acyclovir but requiring less frequent dosing than oral acyclovir. Therefore, it has much the same antiviral indications and safety as oral acyclovir with the advantage of simpler dosing. For herpetic epithelial keratitis or iritis, it is used in a dose of 1000 mg twice or thrice a day which has been shown to be as effective as acyclovir 400 mg 5 times a day. For prophylaxis, it is used in a dose of 1000 mg once daily. A large multicentered randomized double-blind trial of herpes zoster was carried out which compared valacyclovir 1000 mg 3 times daily with acyclovir 800 mg 5 times daily. There was no significant difference between the drugs in the resolution of HZO. Valacyclovir, therefore, is a reasonable alternative to oral acyclovir for the treatment of HZO in immunocompetent patients at a dosage of 1000 mg 3 times daily for 7 days.
11.2.7.2 Famciclovir/Penciclovir
Famciclovir is the diacetyl-6-deoxyester prodrug of the acyclic guanosine analogue penciclovir which is similar in efficacy to acyclovir. Similar to valacyclovir, it is as effective as oral acyclovir with a less frequent dosing requirement. It is used in a dose of 250 mg three times daily for HSV and 500 mg three times daily for VZV. Tyring and associates performed a multicenter randomized study comparing famciclovir 500 mg 3 times daily with oral acyclovir 800 mg 5 times daily for 7 days in 454 immunocompetent patients with HZO. The efficacy of the two drugs was similar, with no significant difference in the percentage of patients who experienced ocular manifestations.
11.2.7.3 Valganciclovir
Valganciclovir is a valyl ester prodrug of ganciclovir that is well absorbed orally and rapidly metabolized to ganciclovir. It provides blood levels similar to that seen after intravenous infusion of ganciclovir and has been FDA approved for the induction and maintenance treatment of CMV retinitis. It is used in a dose of 900 mg twice daily for 3 weeks for induction followed by 900 mg once daily for chronic maintenance.
11.2.7.4 Foscarnet
Foscarnet has also been used for the treatment of CMV retinitis in AIDS patients. It is used in patients who are unresponsive to or intolerant to ganciclovir and in the treatment of acyclovir-resistant herpetic infections. Oral absorption is very poor; hence, the drug is administered intravenously. It is also given intravitreally for the treatment of CMV retinitis.
11.2.7.5 Cidofovir
Cidofovir has been approved only for intravenous therapy of CMV retinitis in AIDS patients. It is given in a dosage of 5 mg/kg once a week for 2 weeks induction and then every 2 weeks for maintenance therapy. Intravitreal injections have also been given for the same.
11.2.7.6 Fomivirsen
It belongs to a new class of drugs of antisense nucleotides to be used clinically. It is administered only via intravitreal injections and is a second-line drug in the treatment of CMV retinitis.33
11.2.8 Conclusion
A variety of antiviral drugs have been identified for the treatment of herpetic and other eye infections. The advent of acyclovir and its prodrugs has revolutionized the treatment of herpetic eye infections. The search for newer drugs with better efficacy and an enhanced safety profile is still on.
11.3 Antibacterial Agents in Ocular Infections
Antibiotics are the substances produced by microorganisms which selectively inhibit the growth of microorganisms (bacteriostatic drugs) or kill the microorganisms (bactericidal drugs). When bacteriostatic drugs are used to treat ocular infection, the host defense mechanisms are ultimately responsible for clearing and eradicating the infective organism (Leeming 1999). In bacterial keratitis, the infection develops in the avascular cornea, and in endophthalmitis it develops in the fluid-filled aqueous or vitreous cavity (Ryan and Durand 2011). In either case, the immune system may be unable to control the microorganism fast enough to prevent the sight-threatening sequelae. Within the first 24 h, pathogens may multiply and release toxins and degradative enzymes that destroy the function and integrity of ocular tissues (Snyder and Glasser 1994).
Therefore, the bactericidal drugs are preferred for the treatment of severe ocular infections. Sometime, for severe systemic infections involve ocular components, systemic antibiotics are instituted with adjunctive topical therapy (Andreoli et al. 2004). Systemic antibiotics have poor penetration into the anterior chamber of the eye, and thus, both systemic and topical aminoglycoside antibiotics, and occasionally subconjunctival injections, are required for effective treatment.
11.3.1 General Classification of Antibacterial Agents
The antibiotics are classified on the basis of the following:
Chemical structure (sulfonamides, diaminopyrimidines, quinolones, β-lactam antibiotics, tetracyclines, nitrobenzene derivatives, macrolides, lincosamides, glycopeptides, oxazolidinones, polypeptides, nitrofuran derivatives, nitroimidazoles, azoles, and nicotinic acid derivatives)
Mechanism of action (inhibit cell wall synthesis, disrupt cell membrane, inhibit protein synthesis, inhibit DNA topoisomerase, interfere with DNA function or synthesis, and interfere with intermediary metabolism)
Spectrum of activity (narrow spectrum and broad spectrum)
Type of action (bacteriostatic and bactericidal), origin (from bacteria, actinomycetes, and fungi)
Type of organism against which they are primarily active (antibacterial, antifungal, antiviral, antiprotozoal, and anthelmintic).
Site of action (usually target against cell wall, cytoplasmic membrane, intermediate metabolites, and DNA synthesis in microorganisms)
11.3.2 Factors Affecting the Choice of Antibacterial Agent
The choice of an antibacterial is based on considerations of pharmacodynamic, pharmacokinetic, and bacteriological characteristics, risk of selecting resistant mutants, and cost. The bioavailability of an antibacterial agent depends on the target bacterial species, the site of infection, and the integrity of the hemato-aqueous barrier (Leeming 1999). The bactericidal agents like Penicillins, cephalosporins, aminoglycosides and fluoroquinolones are used to treat ocular infections. Whereas, bacteriostatic agents like tetracyclines, erythromycin, chloramphenicol and sulfonamides are often used for less severe infections or for specific benefit such as tetracycline in the treatment of ocular rosacea.
Some agents (fusidic acid, quinolones) penetrate the cornea, passing into the anterior chamber of normal eyes at therapeutic concentrations, whereas others (polymyxin B, bacitracin) have no penetrating powers and remain at the surface of the eye. Toxicity is mostly manifested by allergic reactions to excipients or active ingredients in topical antibacterial preparations. A few cases of hematological toxicity have brought suspicion on topical chloramphenicol, but the link has yet to be proven. Erythromycin and polymyxin B are good to use as topical applications in pregnant women and nursing mothers (Robert and Adenis 2001; Leeming 1999).
The ophthalmic antibacterial preparations are frequently used topically either as eye drop or eye ointment in the treatment of patients with superficial ocular infections, including conjunctivitis, blepharitis, and corneal ulcers. In addition, these are also used to augment treatment for intraocular infection administered systemically or by local instillation. Topically administered antibiotics are appropriate for external ocular infections, including the conjunctiva and cornea. The addition of subconjunctival antibiotic administration is indicated for serious corneal as well as anterior segment infections. Intravitreal antibiotic administration is potentially hazardous but indicated for bacterial endophthalmitis. Some cataract surgeons incorporate antibiotics into infusion fluid during phacoemulsification. The knowledge of the pharmacokinetics of topically applied agents is useful in guiding treatment regimens, particularly in serious corneal infections (Robert and Adenis 2001).
Systemic ceftazidime can be used for many Gram-negative bacteria, but intravitreal injection is recommended for better coverage, especially for more-potent organisms. Systemic moxifloxacin can be considered for most Gram-positive and Gram-negative infections due to its excellent intraocular penetration and broad coverage, but the patient’s previous history of its topical use and increasing resistance patterns must be considered (Ahmed et al. 2014). Intracameral cefuroxime is significantly more effective than not using prophylaxis or the use of a topical antibiotic. The economic evaluation comparing different prophylaxis regimens had also shown that intracameral cefuroxime has best cost-effectiveness ratio (Linertová et al. 2014).
Costs of treatment must be evaluated as a whole (regimen, drug associations) as the prices for a bottle of eye drops or the ointments may vary severalfolds. The cheapest drugs include chloramphenicol, polymyxin B, and gentamicin, the most expensive being fusidic acid and the quinolones (Robert and Adenis 2001). Intravitreal antibiotic penetration of systemic antibiotics with or without penetrating ocular injury varies depending on the antibiotic. For prevention or treatment of Gram-positive bacteria-causing endophthalmitis, intravitreal vancomycin is necessary and provides the most reliable coverage.
The widespread use of an antibacterial increases risks of selecting resistance to it. Acquired resistance is well documented for fusidic acid and several cephalosporins and newly described fluoroquinolones. The details of each antibiotics class divided based on mechanism of action used in ocular preparation or ophthalmic usages are described below.
11.3.2.1 Cell Wall Synthesis Inhibitors
Penicillins, cephalosporins, glycopeptide, and polypeptide are the class of antibiotics known to target cell wall synthesis machinery.
Penicillins
The penicillins structurally consist of a thiazolidine ring with a β-lactam ring connected to a side chain. Several of the penicillins are used in ophthalmic preparations. These are as follows: penicillin G, penicillin V (acid-resistant penicillin), methicillin, cloxacillin (penicillinase-resistant penicillins), carbenicillin, mezlocillin, piperacillin, and ticarcillin (extended-spectrum penicillins).
Spectrum of Activity
Penicillins have higher susceptibility against Gram-positive bacteria. Extended-spectrum penicillins have significant activity against Pseudomonas aeruginosa and certain Proteus, Enterobacter, and Acinetobacter spp. that are not susceptible to most other penicillins.
Mechanism of Action
Penicillins act by interfering with synthesis of bacterial cell wall. They inhibit the transpeptidases so that cross-linking (which maintains the close knit structures of the cell wall) does not occur.
Indications
Topical use of penicillins for treatment of eye disease is limited by their narrow spectrum and high incidence of allergic reaction to these drugs. Penicillin G has a narrow spectrum of activity though used for the treatment of patients with keratitis caused by susceptible Streptococcus pneumoniae. A 14-day course of high-dose intravenous penicillin G is first-line therapy for all stages of ocular syphilis and chorioretinitis (Benson et al. 2015). Oral penicillin is used in the treatment of poststreptococcal syndrome uveitis (PSU), a newly recognized immune-mediated response to group A β-hemolytic streptococcus infection (Tinley et al. 2012).
Ticarcillin and piperacillin are used with an aminoglycoside antibiotic topically and subconjunctivally for the treatment of bacterial corneal ulcers caused by Pseudomonas and other Gram-negative rods. Carbenicillin is useful in corneal ulcer caused by the opportunistic organism Achromobacter xylosoxidans which developed during chronic topical steroid treatment of an eye with neovascular glaucoma (Newman et al. 1984). Topical piperacillin/tazobactam is used as an option for the treatment of therapy-resistant P. aeruginosa keratitis (Chew et al. 2010). Also, methicillin is preferred in combination with aminoglycoside for the treatment of keratitis.
Cephalosporins
Cephalosporins are semisynthetic antibiotics derived from a fungus, cephalosporium. It shares its pharmacology with penicillins in structure and mechanism of action. The compounds are commonly classified from first to fifth generation based on their clinical uses and varying spectrum of activity. The cephalosporins that belong to each generation used in ophthalmic preparations are the following: first-generation cephalosporins (cefazolin, cephalexin, and cefadroxil), second-generation cephalosporins (cefamandole, cefaclor, cefprozil, cefoxitin, and cefuroxime), third-generation cephalosporins (ceftriaxone, cefixime, cefoperazone, ceftazidime, ceftibuten, cefdinir, and cefepime), and fourth-generation cephalosporins (cefepime and cefpirome) appear more active against Gram-negative organisms and appear more active against Gram-negative enteric bacteria. All are effective against Gram-positive bacteria, but their activity against Gram-negative bacteria is relatively modest. The activity of cephalosporins increased toward Gram-negative bacteria along with generations.
Spectrum of Activity
Second-generation cephalosporins appear more active against Gram-negative enteric bacteria. Third-generation cephalosporins are more active against Gram-negative organisms.
Mechanism of Action
All cephalosporins are bactericidal and inhibit bacterial cell wall synthesis, similar to the penicillins, but bind to other proteins than penicillin.
Indications
Cefazolin is used to treat bacterial corneal ulcers as part of a broad-spectrum approach in combination with an aminoglycoside or fluoroquinolone such as ciprofloxacin. It is used due to its activity against Gram-positive cocci, including penicillin-resistant Staphylococci. The clinical response is variable in infections caused by the viridans group of Streptococci. Cefazolin is not available as an ophthalmic preparation so it is administered topically as a specially prepared solution or subconjunctivally. It is found to be highly active against Gram-positive bacteria, a common cause of bacterial keratitis (Rautaraya et al. 2014). Cefazolin and cefuroxime are resistant to staphylococcal β-lactamases and used in combination with aminoglycosides in the empirical treatment of keratitis. Cefazolin and cefuroxime intracameral injection are used to decrease the risk of endophthalmitis at the end of cataract surgery (Vazirani and Basu 2013; Kessel et al. 2015).
Second-generation cephalosporins have limited use in ophthalmic practice. Ceftazidime shows excellent activity against Gram-negative bacteria including Pseudomonas aeruginosa and remains the most popular mode of antibiotic prophylaxis in cataract surgery patients for prophylaxis from endophthalmitis (Sridhar et al. 2015). Ceftazidime is also suggested as an alternative for intravitreal amikacin in the treatment of endophthalmitis (Mehta et al. 2011). It possesses high therapeutic index with a lower risk of retinal toxicity than amikacin.
Polypeptide Antibiotic
Bacitracin, gramicidin, and colistin are the polypeptide antibiotics used in ophthalmic topical formulations.
Bacitracin
Spectrum of Activity
It is active against Gram-positive bacteria, including Streptococci and Staphylococci.
Mechanism of Action
It inhibits cell wall synthesis of bacteria by a different mechanism than do the β-lactam antibiotics.
Indications
Because of its systemic renal toxicity, it is used topically alone and in fixed combination products. It is unstable in solution, thus available only as an ointment in combination with neomycin, effective against Gram-negative bacteria and polymyxin B. The inclusion of bacitracin produces broad antibacterial spectra against most common ocular pathogens. Bacitracin appears useful to clear corneal wound infection caused by Gram-positive organisms after phacoemulsification is a serious complication of cataract surgery (Cosar et al. 2001). Bacitracin or trimethoprim-polymyxin B sulfate is found useful for perioperative antibiotic prophylaxis (Ritterband et al. 2003).
Vancomycin
It is a glycopeptide antibiotic first isolated in 1953 from a soil bacterium Amycolatopsis orientalis (formerly known as Nocardia orientalis).
Spectrum of Activity
It possesses broad-spectrum activity against Gram-positive bacteria including methicillin- and cephalosporin-resistant Staphylococci. Also, it shows activity against coagulase-negative Staphylococcus, Gram-positive cocci, including Streptococcus, Staphylococcus, Clostridium, and Corynebacterium (Suemori et al. 2010).
Mechanism of Action
It acts by inhibiting cell wall synthesis by binding to the two D-ala residues on the end of the peptide chain bound to the peptide chains prevents them from interacting properly with the cell wall cross-linking enzyme and cross-links are not formed and the cell wall falls apart.
Indications
It is reserved for serious infections for which less toxic antibiotics are not indicated, not effective, or not tolerated. It is an excellent empiric antibiotic for treating endophthalmitis and an alternative to penicillins or cephalosporins for serious infections (Gentile et al. 2014). It is used as the final choice in serious cases of methicillin-resistant Staphylococcus aureus (MRSA) or methicillin-resistant Staphylococcus epidermidis (MRSE) keratitis. It is recommended as intravitreal, topical, or subconjunctival for treatment of bacterial endophthalmitis (Mehta et al. 2011).
11.3.2.2 Cytoplasmic Membrane Inhibitors
Polymyxin B and gramicidin are the antibiotics known to impair the bacterial cytoplasmic membrane.
Polymyxin B
Spectrum of Activity
It is active against Gram-negative bacteria except Proteus, Serratia, and Neisseria. It is also active against Pseudomonas, Salmonella, and Shigella.
Mechanism of Action
It is surfactant in nature that disrupts the osmotic integrity of bacterial cell membranes.
Indications
Currently, it used as a last resort antibiotic for the treatment of infections caused by Gram-negative bacteria. Topical neomycin/polymyxin B is found effective in reducing the conjunctival bacterial load given before cataract surgery (Li et al. 2015). The combination of topical trimethoprim/polymyxin B and topical moxifloxacin is found to effectively control the corneal ulcer in keratitis caused by Elizabethkingia meningosepticum (Erdem et al. 2013). Polymyxin B and trimethoprim are found useful in infectious keratitis after photorefractive keratectomy (Donnenfeld et al. 2003). Topical therapy with gentamicin 1.3 %, cefazolin 5 %, chlorhexidine 0.02 %, propamidine 0.1 %, polymyxin B 30,000 IU eye drops, and neosporin (neomycin, bacitracin, polymyxin) eye ointment is used for the treatment of coinfection with Acanthamoeba and Pseudomonas aeruginosa in patients with contact lens-associated keratitis (Sharma et al. 2013a, b). Polymyxin B-trimethoprim continues to be an effective treatment for acute conjunctivitis and bacterial keratitis and may combine with chloramphenicol and gentamicin (Williams et al. 2013). Polymyxin B may be used for multidrug-resistant Pseudomonas spp. It is available in combination with various other agents such as bacitracin (ointment), trimethoprim (eye drops and ointment), and neomycin plus gramicidin (eye drops).
Colistin
Spectrum of Activity
It is active against the multidrug-resistant (MDR) Gram-negative organisms except Proteus, Serratia, and Neisseria. It is also active against Pseudomonas, Salmonella, and Shigella.
Mechanism of Action
Its rapidly acting bactericidal agent exhibits a surfactant-like action on the cell membrane and causes distortion or pseudopor formation.
Indications
Topical colistin 0.19 % found a safe and effective alternative in the management of multidrug-resistant P. aeruginosa bacterial keratitis (Jain et al. 2014).
Gramicidin
Spectrum of Activity
It is active against Gram-positive bacteria, except for the Gram-positive bacilli, and against select Gram-negative organisms, such as Neisseria.
Mechanism of Action
It is bactericidal and causes cell membrane leakage and uncouples oxidative phosphorylation in the microorganisms.
Indications
It replaces bacitracin in some fixed combination formulations used topically for eye infections. It is used in therapeutics in the name of tyrothricin which is a mixture of gramicidin (20 %) and tyrocidine (80 %). Its use is limited to topical application only, as it is very toxic on systemic use.
Fusidic Acid
Spectrum of Activity
It is a narrow-spectrum antibiotic effective against Streptococci, Haemophilus, and methicillin-susceptible Staphylococcus aureus.
Mechanism of Action
It inhibits protein synthesis in bacteria.
Indications
It achieves high concentrations at the surface of the eye. It is available as 1 % viscous drops which liquefy in contact with the eye and results in a relatively better half-life in the tear film, therefore reducing the frequency of application compared to other formulations (Mason et al. 2003).
11.3.2.3 Protein Synthesis Inhibitors
Aminoglycosides, tetracyclines, and macrolide antibiotics as well as the individual drugs clindamycin and chloramphenicol inhibit the protein synthesis in bacteria.
Aminoglycosides
Gentamicin, neomycin, netilmicin, tobramycin, and amikacin are the aminoglycoside antibiotics used in ocular preparations. Gentamicin was discovered in 1963 and was introduced into parenteral usage in 1971. Since then, it has been widely used in medicinal applications including eye diseases (Chen et al. 2014).
Spectrum of Activity
These are effective against most Gram-negative bacteria and Staphylococci with lesser activity against Streptococci. Aminoglycosides showed differences in the type and doses associated with toxic reactions; thereby, the following order of toxicity can be described (from most toxic to least toxic): gentamicin > netilmicin = tobramycin > amikacin = kanamycin (Penha et al. 2010). Aminoglycosides display concentration-dependent bactericidal activity and are the cornerstone of therapy against serious Gram-negative bacterial infections.
Mechanism of Action
These inhibit protein synthesis by binding to 30S bacterial ribosomes and cause inaccurate mRNA translation and so inhibit the biosynthesis of proteins preceded by inhibition of ribosomal translocation, i.e., movement of the peptidyl-tRNA from the A to the P site.
Indications
Gentamicin and tobramycin are more active than neomycin and framycetin, particularly against P. aeruginosa. The rapid bactericidal action of gentamicin and tobramycin and their potential activity against P. aeruginosa make them useful in the treatment of bacterial keratitis. They do not penetrate the cornea well; therefore, they are generally used at fortified concentrations until the condition of the cornea improves. Marked irritation can be experienced its use (McDonald et al. 2014).
Gentamicin is used to treat many bacterial infections such as conjunctivitis, blepharitis, and dacryocystitis. It is also used for the initial treatment of bacterial corneal ulcers or prophylaxis of endophthalmitis after cataract surgery by intracameral antibiotics or subconjunctival injection (Katibeh et al. 2015), though it is considered inadequate for the initial treatment of serious bacterial keratitis. The solutions containing fortified concentrations are prepared from sterile products to be intended for parenteral use. The fortified gentamicin or tobramycin solution is combined with a penicillinase-resistant cephalosporin and considered a useful initial empiric treatment for serious bacterial keratitis. The initial loading dose of fortified aminoglycosides is given to increase the antibiotic concentrations in the cornea, followed by regular applications.
Neomycin is most commonly administered topically in combination with other antibiotics or corticosteroids. Topical application frequently results in sensitization to the drug; therefore, long-term use should be avoided. Netilmicin appears the most effective antibiotic tested against both MRSA and MRSE and may curtail the emergence, spreading, and persistence of antibiotic-resistant bacteria (Blanco et al. 2013). Netilmicin appears a safe and broad-spectrum antibiotic comparable with that of ciprofloxacin, ofloxacin, norfloxacin, and gentamicin that can be used as first-line therapy for the treatment of acute bacterial conjunctivitis (Papa et al. 2002).
Tobramycin is used similar to gentamicin; however, Staphylococci are generally susceptible to tobramycin, whereas Streptococci are not susceptible to tobramycin (Donnenfeld et al. 2003). Cross-resistance between gentamicin and tobramycin is common. Amikacin is usually effective for most strains of Klebsiella, Enterobacter, E. coli, and Serratia. However, for bacterial keratitis, tobramycin is often preferred in combination with ticarcillin. Dual therapy with a β-lactam agent is recommended for empirical therapy to cover streptococcal infection and improve activity against Staphylococci. The aminoglycosides are slowly inactivated in the presence of some β-lactams; thus, they should be administered separately, preferably at least 5 min apart.
Tobramycin 1.4 % topical is found useful in mycobacterial keratitis after laser in situ keratomileusis (LASIK) (Freitas et al. 2003) and infectious keratitis after photorefractive keratectomy (Donnenfeld et al. 2003). Tobramycin also may be useful as prophylactic topical antibiotics for preventing secondary corneal infections or recurrent corneal erosion syndrome (Park et al. 2015).
Amikacin, the first semisynthetic aminoglycoside, has been chemically modified to be protected from aminoglycoside-inactivating enzymes. It is popular as a primary antibiotic for intravitreal injection along with vancomycin for the treatment of bacterial endophthalmitis due to its broad spectrum against resistant Gram-negative organisms and reduced toxicity.
Tetracyclines
Tetracyclines were first acknowledged in 1948 as the natural fermentation product of the soil bacterium Streptomyces aureofaciens and, 6 years later, were chemically purified for the first time. Tetracycline analogues are classified as short-, intermediate-, and long-acting based on duration of action. Analogues in each category have generally similar patterns of bacterial susceptibility and resistance.
Spectrum of Activity
Tetracyclines are the broad-spectrum antibiotics, active against Gram-positive, Gram-negative, aerobic, and anaerobic bacteria as well as Spirochetes, Mycoplasma, Rickettsia, Chlamydophila, Brucella, Bartonella, and a few protozoa.
Mechanism of Action
Primarily these are bacteriostatic in action, inhibit protein synthesis by binding to 30S ribosomes, and interfere with attachment of aminoacyl-tRNA to the mRNA-ribosome complex and inhibition of growth of peptide chain required for protein synthesis.
Indications
Tetracyclines also interact with matrix metalloproteinases (MMP), tissue inhibitors of MMPs, growth factors, and cytokines; therefore, tetracyclines are capable of affecting inflammation, immunomodulation, cell proliferation, and angiogenesis. Slow-release doxycycline 40 mg given daily appears an effective and safe therapy of ocular rosacea (Sobolewska et al. 2014). Oral minocycline or doxycycline can provide clinical benefits in treating moderate and severe meibomian gland dysfunction by reducing inflammatory cytokine levels.
Oral doxycycline is also used with topical amikacin, oral ketoconazole in the treatment of keratitis caused by Nocardia organisms. (TRichet et al. 2011). Oral doxycycline and topical corticosteroid are used in the treatment of recurrent corneal erosion syndrome (Wang et al. 2008). Tetracycline and chlortetracycline both are available in the ophthalmic preparations and indicated for the treatment of chlamydial (TRIC) infections. Several ocular surface infections caused by susceptible organisms respond satisfactorily to topical tetracyclines.
Macrolides
These antibiotics have macrocyclic lactone ring attached with sugar. Erythromycin, clarithromycin, and azithromycin are the popular macrolide antibiotics used in ocular preparations.
Spectrum of Activity
It is narrow spectrum and active mainly against Gram-positive cocci, Streptococcus, Staphylococcus, Gram-positive rods, and a few Gram-negative bacteria. It is also effective against Mycoplasma, Rickettsia, and Chlamydophila.
Mechanism of Action
These are primarily bacteriostatic at low concentration but become bactericidal at high concentration. They act by inhibiting protein synthesis through binding to the bacterial 50S ribosomal subunit and interfering with translocation in protein synthesis.
Indications
The first-generation macrolide, erythromycin, is a widely used macrolide antibiotic for human external ocular infections because of its lack of toxicity and good activity against microorganisms. Erythromycin decreases the risk of gonococcal ophthalmia neonatorum in newborns (Darling and McDonald 2010). The American Academy of Pediatrics recommends a 14-day course of systemic erythromycin (50 mg/kg/day, divided in 4 doses).
The second-generation macrolide, azithromycin, is available as a 1.5 % ophthalmic solution for use in the treatment of bacterial or trachomatous conjunctivitis (Garnock-Jones 2012). Azithromycin 1.5 % ophthalmic solution for 3 days (1 drop twice daily) is found non-inferior to tobramycin 0.3 % ophthalmic solution for 7 days (1 drop every 2 h) in pediatric and adult patients with purulent bacterial conjunctivitis, with regard to clinical cure and bacteriological resolution. Azithromycin administered orally is rapidly absorbed and widely distributed. A single oral dose of azithromycin can eliminate trachoma infection, but cannot be used in infants under 6 months old, and needs to be given every few years in communities with a high prevalence of disease (Baneke 2012).
Azithromycin 1 % ophthalmic solution is also used in the treatment of blepharitis and blepharitis-associated ocular dryness (Veldman and Colby 2011). Azithromycin 1.5 % ophthalmic solution was found effective in bacterial or trachomatous conjunctivitis and appears well tolerated (Garnock-Jones 2012). Topical azithromycin is also used in meibomian gland dysfunction, a common problem associated with evaporative dry eye disease (Foulks et al. 2013).
Mass azithromycin treatments are highly effective for the ocular strains of chlamydia causing trachoma (Keenan et al. 2012). Several extra-label dosage regimens have been anecdotally recommended (e.g., 5 mg/kg once daily for 2 doses and then every third day for 5 doses, 5 mg/kg once daily for 5 doses followed by the same dose every 3 days for 5 doses). A short course of oral azithromycin (20 mg/kg once daily for 3 days) appears an effective treatment alternative for Chlamydia trachomatis.
Macrolides are considered bacteriostatic, but clarithromycin may provide a bactericidal effect against non-tuberculous mycobacteria keratitis if used at a high concentration. Clarithromycin 1 % topical is found useful in mycobacterial keratitis after laser in situ keratomileusis (LASIK) (Freitas et al. 2003).
Chloramphenicol
It was first obtained from Streptomyces venezuelae in 1947.
Spectrum of Activity
It is a broad-spectrum antibiotic and active against most Gram-positive and Gram-negative bacteria, Rickettsia, Chlamydophila, Spirochetes, and Mycoplasma except P. aeruginosa or Chlamydia trachomatis. It is effective against Haemophilus influenzae and Haemophilus parainfluenzae, Legionella pneumophila, Moraxella catarrhalis, Neisseria meningitidis, Pasteurella multocida, and Streptococcus pneumonia (Cagini et al. 2013).
Mechanism of Action
It binds to 30S bacterial ribosomes and hinders the access of aminoacyl-tRNA to the acceptor site for amino acid incorporation and inhibits protein synthesis. Primarily, it is bacteriostatic in action.
Indications
It is one of the oldest and commonest antibiotics, available over the counter and considered least expensive. It is used for the treatment of methicillin-resistant Staphylococcus aureus ocular surface infections and bacterial conjunctivitis and available as 0.5 % drops and 1 % ointments (Fukuda et al. 2002). A high incidence of resistance was reported, and even topical use also may cause bone marrow toxicity and aplastic anemia.
11.3.2.4 Intermediary Metabolism Inhibitors
The sulfonamides are the first antimicrobial agents and considered derivative of sulfanilamide. Several sulfonamides were developed and used extensively, but the emergence of resistance and availability of safer and effective agents currently limited the clinical usage. Currently they are used mainly in combination with pyrimethamine and trimethoprim.
Spectrum of Activity
Sulfonamides are broad-spectrum drugs found effective against Gram-positive and Gram-negative bacteria as well as Chlamydophila, Actinomyces, Plasmodia, and Toxoplasma. They generally exert bacteriostatic effect; therefore, cellular and humoral immune mechanisms are potentially more important for eradicating bacterial infections when they are used than for some other antibiotic agents.
Mechanism of Action
Mechanistically, sulfonamides inhibit synthesis of folic acid which is required for synthesis of nucleic acid and protein in bacteria. This inhibition can be reversed by several antagonists, i.e., para-aminobenzoic acid (PABA). Antibacterial action of sulfonamides is also inhibited by tissue breakdown products, blood and pus. Thus, sulfonamide treatment is contraindicated for infections with marked suppuration. Pyrimethamine and trimethoprim are chemically 4-diaminapyrimidine derivatives which inhibit folic acid synthesis. They appear synergistic when used in combination with the sulfonamides. The sulfonamides inhibit an early step in the synthesis of folic acid, and pyrimethamine or trimethoprim inhibits a later step in the pathway.
< div class='tao-gold-member'>
Only gold members can continue reading. Log In or Register a > to continue
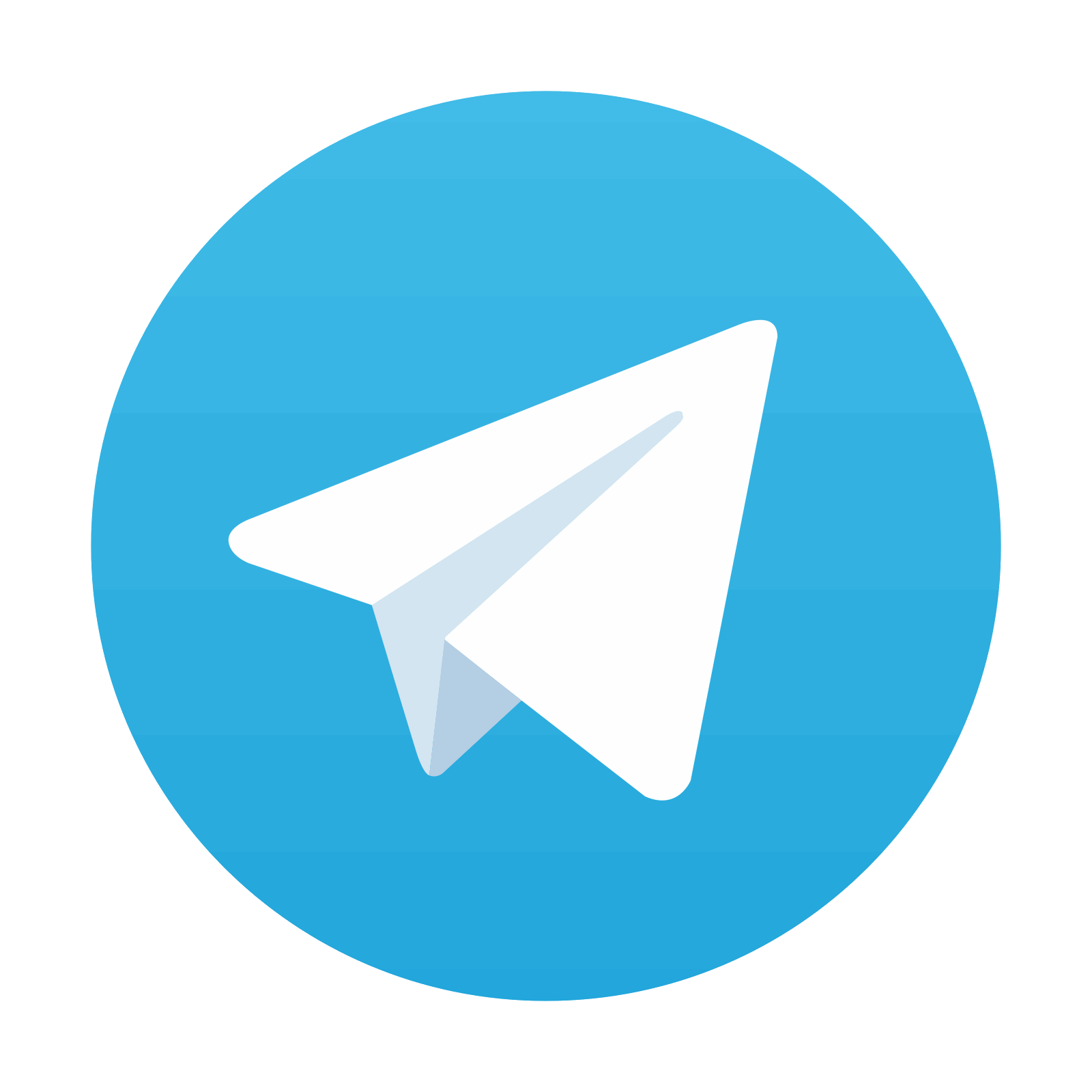
Stay updated, free articles. Join our Telegram channel

Full access? Get Clinical Tree
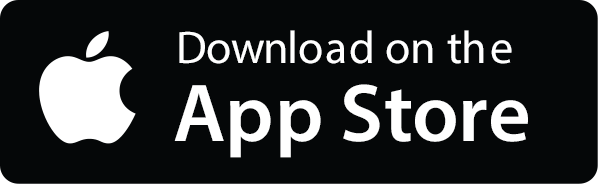
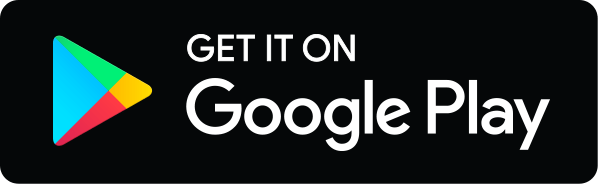