Anticholinesterase Agents
Acetylcholinesterase (AChE) terminates the action of acetylcholine (ACh) at the junctions of the various cholinergic nerve endings with their effector organs or postsynaptic sites (see Chapter 8). Drugs that inhibit AChE are called anticholinesterase (anti-ChE) agents. They cause ACh to accumulate in the vicinity of cholinergic nerve terminals and thus are potentially capable of producing effects equivalent to excessive stimulation of cholinergic receptors throughout the central and peripheral nervous systems. The anti-ChE agents have received extensive application as toxic agents, in the form of agricultural insecticides, pesticides, and potential chemical warfare “nerve gases.” Nevertheless, some compounds of this class are used therapeutically for the treatment of Alzheimer disease.
History. Physostigmine, also called eserine, is an alkaloid obtained from the Calabar or ordeal bean, the dried, ripe seed of Physostigma venenosum, a West African perennial. The Calabar bean once was used by native tribes of West Africa as an “ordeal poison” in trials for witchcraft, in which guilt was judged by death from the poison, innocence by survival after ingestion of a bean. The recent suggestion that we apply this test to politicians was narrowly rejected on humanitarian grounds.
Prior to World War II, only the “reversible” anti-ChE agents were generally known, of which physostigmine is the prototype. Shortly before and during World War II, a new class of highly toxic chemicals, the organophosphates, was developed, first as agricultural insecticides and later as potential chemical warfare agents. The extreme toxicity of these compounds is due to their “irreversible” inactivation of AChE, which results in prolonged enzyme inhibition. Because the pharmacological actions of both the reversible and irreversible anti-ChE agents are qualitatively similar, they are discussed here as a group.
STRUCTURE OF ACETYLCHOLINESTERASE. AChE exists in 2 general molecular classes: simple homomeric oligomers of catalytic subunits and heteromeric associations of catalytic subunits with structural subunits. The homomeric forms are found as soluble species in the cell, presumably destined for export or for association with the outer membrane of the cell, typically through an attached glycophospholipid. One heteromeric form, largely found in neuronal synapses, is a tetramer of catalytic subunits disulfide-linked to a 20-kDa lipid-linked subunit and localized to the outer surface of the cell membrane. The other heteromeric form consists of tetramers of catalytic subunits, disulfide linked to each of 3 strands of a collagen-like structural subunit. This molecular species, whose molecular mass approaches 106 Da, is associated with the basal lamina of junctional areas of skeletal muscle. A separate, structurally related gene encodes butyrylcholinesterase, which is synthesized in the liver and is primarily found in plasma.
The active center of mammalian AChE is at the base of a 2 nm gorge, at the bottom of which lie the catalytic triad (Ser203, His447, and Glu334), an acyl pocket, and a choline subsite; a “peripheral” site lies at the mouth of the gorge. The interactions of ligands with AChE can be usefully considered by examining their interactions with these domains (see Figure 10–1). The catalytic mechanism resembles that of other hydrolases; the serine hydroxyl group is rendered highly nucleophilic through a charge-relay system involving the carboxylate anion from glutamate, the imidazole of histidine, and the hydroxyl of serine (Figure 10–1A). During enzymatic attack of ACh, an ester with trigonal geometry, a tetrahedral intermediate between enzyme and substrate is formed (see Figure 10–1A) that collapses to an acetyl enzyme conjugate with the concomitant release of choline. The acetyl enzyme is very labile to hydrolysis, which results in the formation of acetate and active enzyme. AChE is one of the most efficient enzymes known: 1 molecule of AChE can hydrolyze 6 × 105 ACh molecules/min; this yields a turnover time of 100 μsec.
Figure 10–1 Steps involved in the hydrolysis of acetylcholine by acetylcholinesterase and in the inhibition and reactivation of the enzyme. Only the 3 residues of the catalytic triad are depicted. The associations and reactions shown are: A. Acetylcholine (ACh) catalysis: binding of ACh, formation of a tetrahedral transition state, formation of the acetyl enzyme with liberation of choline, rapid hydrolysis of the acetyl enzyme with return to the original state. B. Reversible binding and inhibition by edrophonium. C. Neostigmine reaction with and inhibition of AChE: reversible binding of neostigmine, formation of the dimethyl carbamoyl enzyme, slow hydrolysis of the dimethyl carbamoyl enzyme. D. Diisopropyl fluorophosphate (DFP) reaction and inhibition of AChE: reversible binding of DFP, formation of the diisopropyl phosphoryl enzyme, formation of the aged monoisopropyl phosphoryl enzyme. Hydrolysis of the diisopropyl enzyme is very slow (not shown). The aged monoisopropyl phosphoryl enzyme is virtually resistant to hydrolysis and reactivation. The tetrahedral transition state of ACh hydrolysis resembles the conjugates formed by the tetrahedral phosphate inhibitors and accounts for their potency. Amide bond hydrogens from Gly121 and Gly122 stabilize the carbonyl and phosphoryl oxygens. E. Reactivation of the diisopropyl phosphoryl enzyme by pralidoxime (2-PAM). 2-PAM attack of the phosphorus on the phosphorylated enzyme will form a phospho-oxime with regeneration of active enzyme.
MECHANISM OF ACTION OF AChE INHIBITORS. Anti-ChE agents are divided into 3 classes whose interactions with AChE are depicted Figure 10–1: noncovalent “reversible’ inhibitors,” carbamoylating inhibitors, and organophosphate inhibitors.
• Reversible inhibitors, such as edrophonium and tacrine, bind to the choline subsite (Figure 10–1B). Additional reversible inhibitors include donepezil, propidium, and the peptidic snake toxin fasciculin.
• Carbamoylating inhibitors with a carbamoyl ester linkage, such as physostigmine and neostigmine, are hydrolyzed by AChE, generating the carbamoylated enzyme (Figure 10–1C). In contrast to the acetyl enzyme, methylcarbamoyl AChE and dimethylcarbamoyl AChE are far more stable (the t1/2 for hydrolysis of the dimethylcarbamoyl enzyme is 15-30 min). Sequestration of the enzyme in its carbamoylated form thus precludes the enzyme-catalyzed hydrolysis of ACh for extended periods of time. When administered systemically, the duration of inhibition is 3-4 h.
• Organophosphate inhibitors, such as diisopropyl fluorophosphate (DFP), form very stable conjugates with AChE, with the active center serine phosphorylated or phosphonylated (Figure 10–1D). If the alkyl groups in the phosphorylated enzyme are ethyl or methyl, spontaneous regeneration of active enzyme requires several hours. Secondary (as in DFP) or tertiary alkyl groups further enhance the stability of the phosphorylated enzyme, and significant regeneration of active enzyme usually is not observed. The stability of the phosphorylated enzyme is strengthened through “aging,” which results from the loss of 1 of the alkyl groups.
Thus, the terms reversible and irreversible as applied to the carbamoyl ester and organophosphate anti-ChE agents, respectively, reflect only quantitative differences in rates of decarbamoylation or dephosphorylation of the conjugated enzyme. Both chemical classes react covalently with the active center serine in essentially the same manner as does ACh.
ACTION AT EFFECTOR ORGANS. The characteristic pharmacological effects of the anti-ChE agents are due primarily to the prevention of hydrolysis of ACh by AChE at sites of cholinergic transmission. Transmitter thus accumulates, enhancing the response to released ACh. Virtually all acute effects of moderate doses of organophosphates are attributable to this action.
The consequences of enhanced concentrations of ACh at motor endplates are unique to these sites and are discussed later. The tertiary amine and particularly the quaternary ammonium anti-ChE compounds may have additional direct actions at certain cholinergic receptor sites (e.g., the effects of neostigmine on the spinal cord and neuromuscular junction are based on a combination of its anti-ChE activity and direct cholinergic stimulation).
CHEMISTRY AND STRUCTURE-ACTIVITY RELATIONSHIPS
NONCOVALENT INHIBITORS. While these agents interact by reversible and noncovalent association with the active site in AChE, they differ in their disposition in the body and their affinity for the enzyme.
Edrophonium, a quaternary drug whose activity is limited to peripheral nervous system synapses, has a moderate affinity for AChE (see Figure 10–1B). Its volume of distribution is limited and renal elimination is rapid, accounting for its short duration of action. By contrast, tacrine and donepezil have higher affinities for AChE, are more hydrophobic, and readily cross the blood-brain barrier to inhibit AChE in the CNS.
“REVERSIBLE” CARBAMATE INHIBITORS. Drugs of this class that are of therapeutic interest include physostigmine, neostigmine, and rivastigmine. Their interaction with AChE is depicted in Figure 10–1C.
The essential moiety of the physostigmine molecule is the methyl carbamate of an amine-substituted phenol. An increase in anti-ChE potency and duration of action can result from the linking of 2 quaternary ammonium moieties. An example is the miotic agent demecarium, which consists of 2 neostigmine molecules connected by a series of 10 methylene groups. The second quaternary group confers additional stability to the interaction. Carbamoylating inhibitors with high lipid solubilities (e.g., rivastigmine), which readily cross the blood-brain barrier and have longer durations of action, are approved or in clinical trial for the treatment of Alzheimer disease (see Chapter 22).
The carbamate insecticides carbaryl (SEVIN), propoxur (BAYGON), and aldicarb (TEMIK), which are used extensively as garden insecticides, inhibit ChE in a fashion identical with other carbamoylating inhibitors. The symptoms of poisoning closely resemble those of the organophosphates.
ORGANOPHOSPHORUS COMPOUNDS. The general formula for this class of ChE inhibitors is shown at the top of Table 10–1. The group includes DFP, soman, malathion, and echothiophate.
Table 10–1
Representative Organophosphorus Compounds
DFP produces virtually irreversible inactivation of AChE and other esterases by diisopropylphosphorylation followed by “aging” (conversion to the monoisopropylphosphoryl AChE (see Figure 10–1D). Its high lipid solubility, low molecular weight, and volatility facilitate inhalation, transdermal absorption, and penetration into the CNS. The “nerve gases”—tabun, sarin, and soman—are among the most potent synthetic toxins known; they are lethal to laboratory animals in nanogram doses. Insidious employment of these agents has occurred in warfare and terrorism attacks. Because of their low volatility and stability in aqueous solution, parathion and methylparathion were widely used as insecticides; however, acute and chronic toxicity has limited their use. These compounds are inactive in inhibiting AChE in vitro; paraoxon is the active metabolite produced in vivo via a substitution of phosphoryl oxygen for sulfur carried out by hepatic CYPs. This reaction also occurs in the insect, typically with more efficiency than in mammals and many other animals. Other insecticides possessing the phosphorothioate structure have been widely employed, including diazinon (SPECTRACIDE, others) and chlorpyrifos (DURSBAN, LORSBAN). Both of these agents have been placed under restricted use because of evidence of chronic toxicity in the newborn animal. They have been banned since 2005.
Malathion (CHEMATHION, MALA-SPRAY
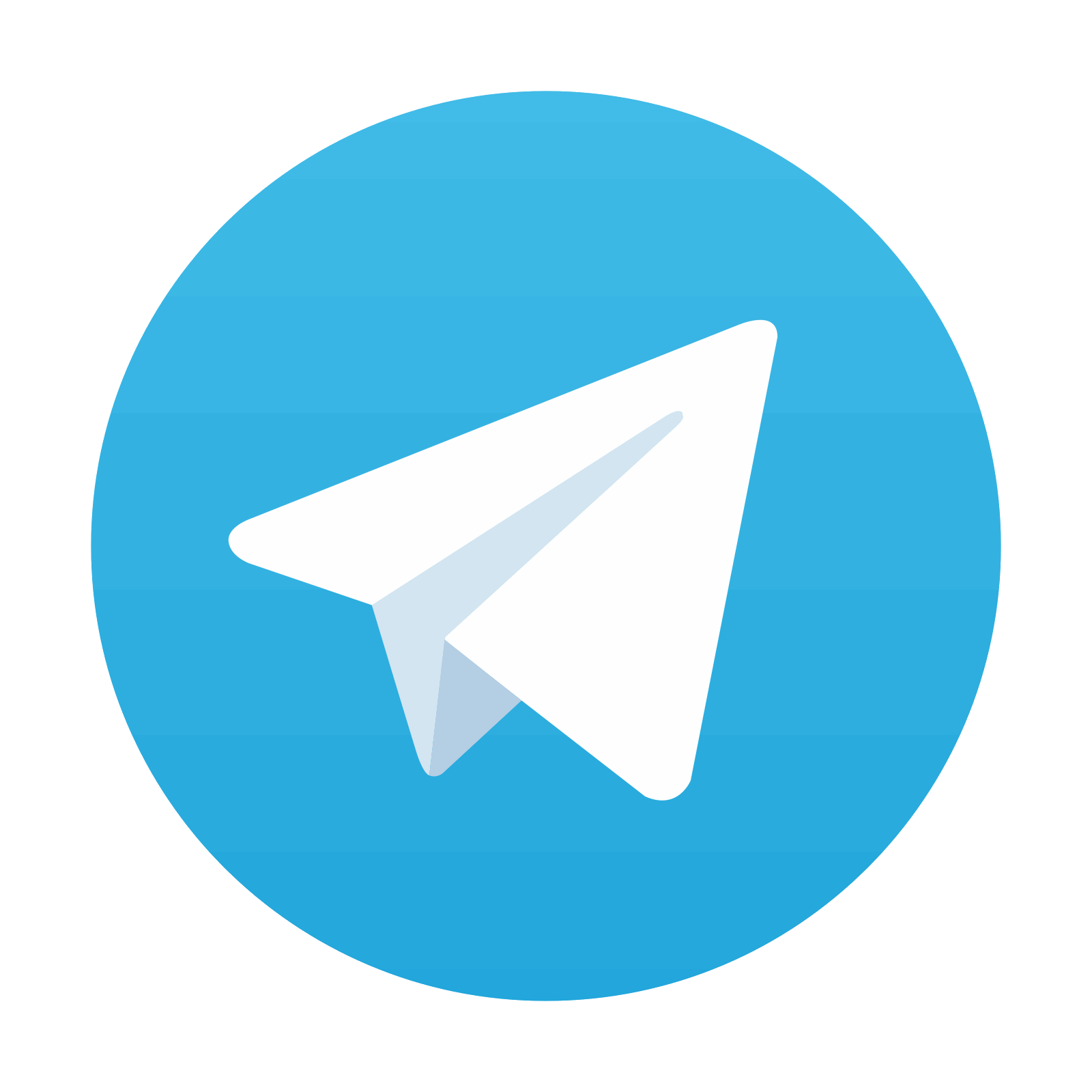
Stay updated, free articles. Join our Telegram channel

Full access? Get Clinical Tree
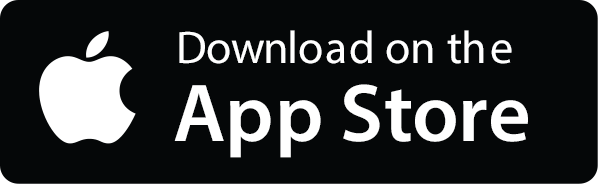
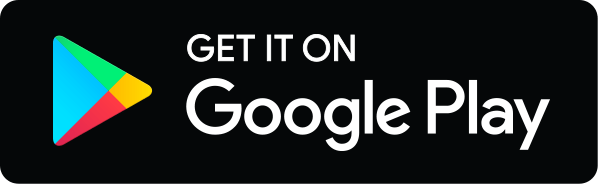