Anemias
KEY CONCEPTS
Anemia is a group of diseases characterized by a decrease in either hemoglobin (Hb) or the volume of red blood cells (RBCs), which results in decreased oxygen-carrying capacity of the blood. Anemia is defined by the World Health Organization as Hb <13 g/dL (<130 g/L; <8.07 mmol/L) in men and <12 g/dL (<120 g/L; <7.45 mmol/L) in women.
Acute-onset anemias are most likely to present with tachycardia, lightheadedness, and dyspnea. Chronic anemia often presents with weakness, fatigue, headache, vertigo, and pallor.
Iron-deficiency anemia (IDA) is characterized by decreased levels of ferritin (most sensitive marker) and serum iron, as well as decreased transferrin saturation. Hb and hematocrit decrease later. RBC morphology includes hypochromia and microcytosis. Most patients are adequately treated with oral iron therapy, although parenteral iron therapy is necessary in selected patient populations.
Vitamin B12 deficiency, a macrocytic anemia, can be due to inadequate intake, malabsorption syndromes, and inadequate utilization. Anemia caused by lack of intrinsic factor, resulting in decreased vitamin B12 absorption, is called pernicious anemia. Neurologic symptoms can be present and can become irreversible if the vitamin B12 deficiency is not treated promptly. Oral or parenteral therapy can be used for replacement.
Folic acid deficiency, a macrocytic anemia, results from inadequate intake, decreased absorption, and increased folate requirements. Treatment consists of oral administration of folic acid, even for patients with absorption problems. Adequate folic acid intake is essential in women of childbearing age to decrease the risk of neural tube defects in their children.
Anemia of inflammation (AI) is a newer term used to describe both anemia of chronic disease and anemia of critical illness. AI is a diagnosis of exclusion. It results from chronic inflammation, infection, or malignancy and can occur as early as 1 to 2 months after the onset of the disease. The serum iron level usually is decreased, but in contrast to IDA, the serum ferritin concentration is normal or increased. Treatment is aimed at correcting the underlying pathology. Anemia of critical illness occurs within days of acute illness.
Anemia is one of the most prevalent clinical problems in the elderly, although not an inevitable complication of aging. Low Hb concentrations are not “normal” among elders. Anemia is associated with an increased risk of hospitalization and mortality, reduced quality of life, and decreased physical functioning in the elderly.
IDA is a leading cause of infant morbidity and mortality. Age- and sex-adjusted norms must be used in the interpretation of laboratory results for pediatric patients. Primary prevention of IDA is the goal. A therapeutic trial of oral iron is the standard of care.
Anemia affects a large part of the world’s population. According to the World Health Organization, almost 1.6 billion people (25% of the world’s population) are anemic. Anemia is defined by the World Health Organization as hemoglobin (Hb) <13 g/dL (<130 g/L; <8.07 mmol/L) in men or <12 g/dL (<120 g/L; <7.45 mmol/L) in women. In the United States, about 3.5 million Americans have anemia based on self-reported data from the National Center for Health Statistics. It is estimated that millions of people are unaware they have anemia, making it one of the most underdiagnosed conditions in the United States. Iron deficiency is the leading cause of anemia worldwide, accounting for as many as 50% of cases.1 Recent data show that the overall prevalence of anemia has declined in the United States in preschool-aged children and women of childbearing age over the past 20 years, but the prevalence of IDA did not change significantly in these same groups. The reasons for these changes remain unclear.2 Although nutritional deficiencies occur less often in the United States, obesity surgery, which can cause deficiencies, is becoming increasingly common. Gastric bypass may result in folate, vitamin B12, and iron deficiencies. Prevalence data are confounded by the lack of a standardized definition of anemia and lack of screening guidelines for most populations. The United States Preventive Services Task Force (USPSTF) guidelines for pregnant women recommend routine screening for IDA.
Anemia is not an innocent bystander because it can affect both length and quality of life. Retrospective observational studies of hemodialysis patients and heart failure patients suggest that anemia is an independent risk factor for mortality.3 In addition, anemia significantly influences morbidity in patients with end-stage renal disease, chronic kidney disease, and heart failure.4 Anemia is associated with psychomotor and cognitive abnormalities in children. Similarly, anemia is associated with cognitive dysfunction in patients with renal failure or cancer, and among community-dwelling elders.5 Anemia during pregnancy is associated with increased risk for low birth weights, preterm delivery, and perinatal mortality.6 Maternal IDA may be associated with postpartum depression in mothers and poor performance by offspring on mental and psychomotor tests. Global goals of treatment in anemic patients are to alleviate signs and symptoms, correct the underlying etiology, and prevent recurrence of anemia.
Anemia is a group of diseases characterized by a decrease in either Hb or circulating red blood cells (RBCs), resulting in reduced oxygen-carrying capacity of the blood. Anemia can result from inadequate RBC production, increased RBC destruction, or blood loss. It can be a manifestation of a host of systemic disorders, such as infection, chronic renal disease, or malignancy. Because anemia is a sign of underlying pathology, rapid diagnosis of the cause may be essential.
The functional classification of anemia is shown in Figure 80–1. This chapter focuses on the most common causes of anemia—IDA, anemia associated with vitamin B12 or folic acid deficiency, and anemia of inflammation (AI). Some of the other causes of anemia are addressed in other chapters.
FIGURE 80-1 Functional classification of anemia. Each of the major categories of anemia (hypoproliferative, maturation disorders, and hemorrhage/hemolysis) can be further subclassified according to the functional defect in the several components of normal erythropoiesis.
Characteristic changes in the size of RBCs seen in erythrocyte indices can be the first step in the morphologic classification and understanding of the anemia. Anemia can be classified by RBC size as macrocytic, normocytic, or microcytic. Vitamin B12 deficiency and folic acid deficiency both are macrocytic anemias. An example of a microcytic anemia is iron deficiency, whereas a normocytic anemia may be associated with recent blood loss or chronic disease. More than one etiology of anemia can occur concurrently. Inclusion of the underlying cause of the anemia makes diagnostic terminology easier to understand (e.g., microcytic anemia secondary to iron deficiency).
Microcytic anemias are a result of a quantitative deficiency in Hb synthesis, usually due to iron deficiency or impaired iron utilization. As a result, erythrocytes containing insufficient Hb are formed. Microcytosis and hypochromia are the morphologic abnormalities that provide evidence of impaired Hb synthesis.
Macrocytic anemias can be divided into megaloblastic and nonmegaloblastic anemias. The type of macrocytic anemia can be distinguished microscopically by peripheral blood smear examination. Megaloblasts are distinctive cells that express a biochemical abnormality of retarded DNA synthesis, resulting in unbalanced cell growth. Megaloblastic anemias may affect all hematopoietic cell lines. The most common causes of megaloblastic anemia are vitamin B12 and folate deficiency. Nonmegaloblastic macrocytic anemias may arise from liver disease, hypothyroidism, hemolytic processes, and alcoholism. Hemolytic anemias often are macrocytic, reflecting the increased numbers of circulating reticulocytes, which are larger on average than mature red cells.
MATURATION AND DEVELOPMENT OF RED BLOOD CELLS
In adults, RBCs are formed in the marrow of the vertebrae, ribs, sternum, clavicle, pelvic (iliac) crest, and proximal epiphyses of the long bones. In children, most bone marrow space is hematopoietically active to meet increased RBC requirements.
In normal RBC formation, a pluripotent stem cell yields an erythroid burst-forming unit. Erythropoietin (EPO) and cytokines such as interleukin-3 and granulocyte–macrophage colony-stimulating factor stimulate this cell to form an erythroid colony-forming unit in the marrow (Fig. 80–2). During this process, the nucleus becomes smaller with each division, finally disappearing in the normal erythrocyte. Hb and iron are incorporated into the gradually maturing RBC, which eventually is released from the marrow into the circulating blood as a reticulocyte. The maturation process usually takes about 1 week. The reticulocyte loses its nucleus and becomes an erythrocyte within several days. The circulating erythrocyte is a nonnucleated, nondividing cell. More than 90% of the protein content of the erythrocyte consists of the oxygen-carrying molecule Hb. Erythrocytes have a normal survival time of 120 days.7
FIGURE 80-2 Erythrocyte maturation sequence (EPO, erythropoietin; GM-CSF, granulocyte-macrophage colony-stimulating factor; IL-3, interleukin-3).
Stimulation of Erythropoiesis
The hormone EPO, 90% of which is produced by the kidneys, initiates and stimulates the production of RBCs. Erythropoiesis is regulated by a feedback loop. The main mechanism of action of EPO is to prevent apoptosis, or programmed cell death, of erythroid precursor cells and allow their proliferation and subsequent maturation. A decrease in tissue oxygen concentration signals the kidneys to increase the production and release of EPO into the plasma, which increases production and maturation of RBCs. Under normal circumstances, the RBC mass is kept at an almost constant level by EPO matching new erythrocyte production to the natural rate of loss of RBCs. A summary of erythropoiesis is shown in Figure 80–3. Early appearance of large quantities of reticulocytes in the peripheral circulation (reticulocytosis) is an indication of increased RBC production.7
FIGURE 80-3 Physiologic regulation of red cell production by tissue oxygen tension. (Reproduced with permission from Adamson JW, Longo DL. Anemia and polycythemia. In: Longo DL, Fauci AS, Kasper DL, et al., eds. Harrison’s Principles of Internal Medicine. 18th ed. New York: Copyright © McGraw-Hill; 2012: http://www.accessmedicine.com/content.aspx?aID=9113377.)
Synthesis of Hemoglobin
Hb contains a protein component with two α-chains and two β-chains. Each chain is linked to a heme group consisting of a porphyrin ring structure with an iron atom chelated at its center, which is capable of binding oxygen. The initial step in the synthesis of heme from the substrate succinyl CoA and glycine requires the presence of pyridoxine phosphate (vitamin B6) as a catalyst. Following its synthesis in the cytoplasmic mitochondria of the RBC, heme diffuses into the extramitochondrial space, where it combines with the completed α– and β-chains and forms Hb. When hemolytic destruction of RBCs exceeds marrow production capacity and anemia develops, the Hb value decreases to a steady-state level at which production is equal to destruction.
Incorporation of Iron into Heme
Iron is an essential part of Hb. The specific plasma transport protein transferrin delivers iron to the bone marrow for incorporation into the Hb molecule. Transferrin enters cells by binding to transferrin receptors, which circulate and then attach to cells needing iron. Fewer transferrin receptors are present on the surface of cells that do not need iron, thus preventing iron-replete cells from receiving excess iron.8
Circulating transferrin normally is about 30% saturated with iron. Transferrin delivers extra iron to other body storage sites, such as the liver, marrow, and spleen, for later use. This iron is stored within macrophages as ferritin or hemosiderin. Ferritin consists of a Fe3+ hydroxyphosphate core surrounded by a protein shell called apoferritin. Hemosiderin can be described as compacted ferritin molecules with an even greater iron-to-protein shell ratio. Physiologically it is a more stable, but less available, form of storage iron. Since total body iron storage is generally reflected by ferritin levels, low serum levels of ferritin provide strong evidence of IDA.9
Normal Destruction of Red Blood Cells
Phagocytic breakdown destroys older blood cells, primarily in the spleen but also in the marrow (Fig. 80–4). Amino acids from the globin chains return to an amino acid pool; heme oxygenase acts on the porphyrin heme structure to form biliverdin and to release its iron. Iron returns to the iron pool to be reused, although biliverdin is further catabolized to bilirubin. The bilirubin is released into the plasma, where it binds to albumin and is transported to the liver for glucuronide conjugation and excretion via bile. If the liver is unable to perform the conjugation, as occurs with intrinsic liver disease or oversaturation of conjugation enzymes by excessive cell hemolysis, the result is an elevated indirect (unconjugated) bilirubin. If the biliary excretion pathway for conjugated bilirubin is obstructed, an elevated direct bilirubin results. Comparison of direct and indirect bilirubin values helps to determine if the defect in bilirubin clearance occurs before or after bilirubin enters the liver. The Hb in RBCs destroyed by intravascular hemolysis becomes attached to haptoglobin and is carried back to the marrow for processing in the normal manner.10
FIGURE 80-4 Destruction of red blood cells (RBCs).
DIAGNOSIS OF ANEMIA
General Presentation
History, physical examination, and laboratory testing are used in the evaluation of the patient with anemia. The workup determines if the patient is bleeding and investigates potential causes of the anemia, such as increased RBC destruction, bone marrow suppression, or iron deficiency. Diet can also be important in identifying causes of anemia. Additionally, information about concurrent nonhematologic disease states and a drug history are essential when evaluating the cause of the anemia (see eChap. 23). History of blood transfusions and exposure to toxic chemicals also should be obtained.
Presenting signs and symptoms of anemia depend on its rate of development and the age and cardiovascular status of the patient. Severity of symptoms does not always correlate with the degree of anemia. Healthy patients may acclimate to very low Hb concentrations if the anemia develops slowly. Mild anemia often is associated with no clinical symptoms and may be found incidentally upon obtaining a complete blood count (CBC) for other reasons. The signs and symptoms in elderly patients with anemia may be attributed to their age or concomitant disease states. The elderly may not tolerate levels of Hb tolerated by younger persons. Similarly, patients with cardiac or pulmonary disease may be less tolerant of mild anemia. Premature infants with anemia may be asymptomatic or have tachycardia, poor weight gain, increased supplemental oxygen needs, or episodes of apnea or bradycardia.
Anemia of rapid onset is most likely to present with cardiorespiratory symptoms such as palpitations, angina, orthostatic lightheadedness, and breathlessness due to decreased oxygen delivery to tissues or hypovolemia in those with acute bleeding. The patient also may have tachycardia and hypotension.
If onset is more chronic, presenting symptoms may include fatigue, weakness, headache, orthopnea, dyspnea on exertion, vertigo, faintness, sensitivity to cold, pallor, and loss of skin tone. Traditional signs of anemia, such as pallor, have limited sensitivity and specificity and may be misinterpreted. With chronic bleeding, there is time for equilibration within the extravascular space, so faintness and lightheadedness are less common.
Possible manifestations of IDA include glossal pain, smooth tongue, reduced salivary flow, pica (compulsive eating of nonfood items), and pagophagia (compulsive eating of ice). These symptoms are not likely to appear unless the anemia is severe.
Neurologic findings in vitamin B12 deficiency may precede hematologic changes. Early neurologic findings may include numbness and paraesthesias. Ataxia, spasticity, diminished vibratory sense, decreased proprioception, and imbalance may occur later as demyelination of the dorsal columns and corticospinal tract develop. Vision changes may result from optic nerve involvement. Psychiatric findings include irritability, personality changes, memory impairment, depression, and, infrequently, psychosis.
Anemia associated with folate deficiency is typically macrocytic but, unlike B12 deficiency, occurs without neurological symptoms. Although the symptoms of anemia will improve with folate replacement and a partial hematologic response will occur, the neurologic manifestations of vitamin B12 deficiency will not be reversed with folic acid replacement therapy and consequently may progress or become irreversible if not treated appropriately.
Laboratory Evaluation
The initial evaluation of anemia involves a CBC (including RBC indices), reticulocyte index, and possibly an examination of a stool sample for occult blood. The results of the preliminary evaluation determine the need for other studies, such as examination of a peripheral blood smear. Based on laboratory test results, anemia can be categorized into three functional defects: RBC production failure (hypoproliferative), cell maturation ineffectiveness, or increased RBC destruction or loss (Fig. 80–1).
CLINICAL PRESENTATION Anemia
Figure 80–5 shows a broad, general algorithm for the diagnosis of anemia based on laboratory data. There are many exceptions and additions to this algorithm, but it can serve as a guide to the typical presentation of common types and causes of anemia. The algorithm is less useful in the presence of more than one cause of anemia.
FIGURE 80-5 General algorithm for diagnosis of anemias (↓, decreased; MCV, mean corpuscular volume; TIBC, total iron-binding capacity; WBC, white blood cells).
Hemoglobin
Values given for Hb represent the amount of Hb per volume of whole blood. The higher values seen in males are due to stimulation of RBC production by androgenic steroids, whereas the lower values in females reflect the decrease in Hb as a result of blood loss during menstruation. The Hb level can be used as a very rough estimate of the oxygen-carrying capacity of blood. Hb levels may be diminished because of a decreased quantity of Hb per RBC or because of a decrease in the actual number of RBCs.
Hematocrit
Expressed as a percentage, hematocrit (Hct) is the actual volume of RBCs in a unit volume of whole blood. In general, it is about three times the Hb value (when Hb is expressed in g/dL). An alteration in this ratio may occur with abnormal cell size or shape and often indicates pathology. A low Hct indicates a reduction in either the number or the size of RBCs or an increase in plasma volume.
Red Blood Cell Count
The RBC count is an indirect estimate of the Hb content of the blood; it is an actual count of RBCs per unit of blood.
Red Blood Cell Indices
Wintrobe indices describe the size and Hb content of the RBCs and are calculated from the Hb, Hct, and RBC count. RBC indices, such as mean corpuscular volume (MCV) and mean corpuscular hemoglobin (MCH), are single mean values that do not express the variation that can occur in cells.
Mean Cell Volume (Hct/RBC Count) MCV represents the average volume of RBCs. It may reflect changes in MCH. Cells are considered macrocytic if they are larger than normal, microcytic if they are smaller than normal, and normocytic if their size falls within normal limits. Folic acid and vitamin B12 deficiency anemias yield macrocytic cells, whereas iron deficiency and thalassemia are examples of microcytic anemias. When IDA (decreased MCV) is accompanied by folate deficiency (increased MCV), the overall MCV may be normal. Failure to understand that the MCV represents an average RBC size creates the potential for overlooking some causes of the anemia.
Mean Cell Hemoglobin (Hb/RBC Count) MCH is the amount of Hb in a RBC, and usually increases or decreases with the MCV. Two morphologic changes, microcytosis and hypochromia, can reduce MCH. A microcytic cell contains less Hb because it is a smaller cell, while a hypochromic cell has a low MCH because of the decreased concentration of Hb present in the cell. Cells can be both microcytic and hypochromic, as seen with IDA. The MCH alone cannot distinguish between microcytosis and hypochromia. The most common cause of an elevated MCH is macrocytosis (e.g., vitamin B12 or folate deficiency).
Mean Cell Hemoglobin Concentration (Hb/Hct) The concentration of Hb per volume of cells is the mean cell hemoglobin concentration (MCHC). Because MCHC is independent of cell size, it is more useful than MCH in distinguishing between microcytosis and hypochromia. A low MCHC indicates hypochromia; a microcyte with a normal Hb concentration will have a low MCH but a normal MCHC. A decreased MCHC is seen most often in IDA.
Total Reticulocyte Count
The total reticulocyte count is an indirect assessment of new RBC production. It reflects how quickly immature RBCs (reticulocytes) are produced by bone marrow and released into the blood. Reticulocytes circulate in the blood about 2 days before maturing into RBCs. About 1% of RBCs are normally replaced daily, representing a reticulocyte count of 1%. The reticulocyte count in normocytic anemia can differentiate hypoproliferative marrow from a compensatory marrow response to an anemia. A lack of reticulocytosis in anemia indicates impaired RBC production. Examples include iron deficiency, B12 deficiency, anemia of chronic disease (ACD), malnutrition, renal insufficiency, and malignancy. A high reticulocyte count may be seen in acute blood loss or hemolysis. The reticulocyte index can aid in determining the functional classification of an anemia (Fig. 80–5).
Red Blood Cell Distribution Width
The higher the red blood cell distribution width (RDW) is, the more variable is the size of the RBCs. The RDW increases in early IDA because of the release of large, immature, nucleated RBCs to compensate for the anemia, but this change is not specific for IDA. The RDW also can be helpful in the diagnosis of a mixed anemia. A patient can have a normal MCV yet have a wide RDW. This finding indicates the presence of microcytes and macrocytes, which would yield a “normal” average RBC size. Use of RDW to distinguish IDA from ACD is not recommended.
Peripheral Blood Smear
The peripheral blood smear can supplement other clinical data and help establish a diagnosis. Peripheral blood smears provide information on the functional status of the bone marrow and defects in RBC production. Additionally, it provides information on variations in cell size (anisocytosis) and shape (poikilocytosis). Automated blood counters, used for the CBC, can flag specific RBC changes that can be confirmed by a peripheral blood smear. Blood smears are placed on a microscope slide and stained as appropriate. Morphologic examination includes assessment of size, shape, and color. The extent of anisocytosis correlates with increased range of cell sizes. Poikilocytosis can suggest a defect in the maturation of RBC precursors in the bone marrow or the presence of hemolysis.
Serum Iron
The level of serum iron is the concentration of iron bound to transferrin. Transferrin is normally about one-third bound (saturated) to iron. The serum iron level of many patients with IDA may remain within the lower limits of normal because a considerable amount of time is required to deplete iron stores. Serum iron levels show diurnal variation (higher in the morning, lower in the afternoon), but this variation is probably not clinically significant in timing of levels.9 Since serum iron levels are decreased by infection and inflammation, serum iron levels are best interpreted in conjunction with the TIBC. The serum iron level decreases with IDA and ACD and increases with hemolytic anemias and iron overload.
Total Iron-Binding Capacity
An indirect measurement of the iron-binding capacity of serum transferrin, total iron-binding capacity (TIBC) evaluation is performed by adding an excess of iron to plasma to saturate all transferrin with iron. Each transferrin molecule can carry two iron atoms. Normally, about 30% of available iron-binding sites are filled. With this laboratory test, all binding sites are filled to measure TIBC. The excess (unbound) iron is then removed and the serum iron concentration determined. Unlike the serum iron level, the TIBC does not fluctuate over hours or days. TIBC usually is higher than normal when body iron stores are low. The finding of a low serum iron level and a high TIBC suggests IDA. The TIBC is actually a measurement of protein serum transferrin, which can be affected by a variety of factors. Patients with infection, malignancy, inflammation, liver disease, and uremia may have a decreased TIBC and a decreased serum iron level, which are consistent with the diagnosis of ACD.
Percentage Transferrin Saturation
The ratio of serum iron level to TIBC indicates transferrin saturation. It reflects the extent to which iron-binding sites are occupied on transferrin and indicates the amount of iron readily available for erythropoiesis. It is expressed as a percentage, as described in the following formula:
Transferrin normally is 20% to 50% saturated with iron. In IDA, transferrin saturation of 15% or lower is commonly seen.10 Transferrin saturation is a less sensitive and specific marker of iron deficiency than are ferritin levels.
Serum Ferritin
The serum concentration of ferritin (storage iron) is proportional to total iron stores and therefore is the best indicator of iron deficiency or iron overload. Ferritin levels indicate the amount of iron stored in the liver, spleen, and bone marrow cells. Low serum ferritin levels are virtually diagnostic of IDA. In contrast, serum iron levels may decrease in both IDA and ACD. Serum ferritin is an acute phase reactant; so chronic infection or inflammation can increase its concentration independent of iron status, masking depleted tissue stores. This limits the utility of the serum ferritin if the level is normal or high for a chronically ill patient. For these patients, iron, even if present in these tissue stores, may not be available for erythropoiesis.
Soluble Transferrin Receptor
The soluble transferrin receptor (sTfR) assay is a laboratory test considered a sensitive, early, highly quantitative marker of iron depletion. The sTfR concentration is inversely correlated with tissue iron stores, and elevated levels are predictive of iron deficiency. Unlike ferritin, the sTfR is not an acute phase reactant; so its level remains normal for patients with chronic disease. It may be a useful test for distinguishing ACD from IDA.9 The major limitation of this test is that it is not widely available in many laboratories.
Folic Acid
The results of folic acid measurements vary depending on the assay method used. Decreased serum folic acid levels indicate a folate deficiency megaloblastic anemia that may coexist with a vitamin B12 deficiency anemia. Erythrocyte folic acid levels are less variable than serum levels because they are slow to decrease in an acute process such as drug-induced folic acid deficiency and slow to increase with oral folic acid replacement. In addition, erythrocyte folic acid levels have the theoretical advantage of less susceptibility to rapid changes in diet and alcohol intake. Limitations with sensitivity and specificity do exist with measurements of erythrocyte folate. It has been proposed that serum folate assay levels be drawn for patients with MCV >110 fL or for patients with a lower MCV and clinical features suggesting a macrocytic anemia. If the serum folate concentration is normal for a patient with suspected folate deficiency, then the erythrocyte folate level should be measured.11
Vitamin B12
Low levels of vitamin B12 (cyanocobalamin or cobalamin) indicate deficiency. However, a deficiency may exist prior to the recognition of low serum levels. Serum values are maintained at the expense of vitamin B12 tissue stores. Vitamin B12 and folate deficiency may overlap, thus serum levels of both vitamins should be determined. Vitamin B12 levels may be falsely low with folate deficiency, pregnancy, and use of oral contraceptives.12
Schilling Test
This test used to be the “gold standard” for assessing vitamin B12 absorption. Due to its cost, unavailable test components, and complexity, the test is rarely used today. Tests to replace it are under investigation.13
Homocysteine
Vitamin B12 and folate both are required for conversion of homocysteine to methionine. Increased serum homocysteine may suggest vitamin B12 or folate deficiency. Homocysteine levels also can be elevated in patients with vitamin B6 deficiency, renal failure, hypothyroidism, or a genetic defect in cystathionine β-synthase.14
Methylmalonic Acid
A vitamin B12 coenzyme is needed to convert methylmalonyl coenzyme A to succinyl coenzyme A. Patients with vitamin B12 deficiency have increased concentrations of serum methylmalonic acid (MMA), which is a more specific marker for vitamin B12 deficiency than homocysteine. MMA levels are not elevated in folate deficiency because folate does not participate in MMA metabolism. Levels of both MMA and homocysteine usually are elevated prior to the development of hematologic abnormalities and reductions in serum vitamin B12 levels.12 MMA levels must be interpreted cautiously for patients with renal disease and hypovolemia because the levels may be elevated due to decreased urinary excretion.
IRON-DEFICIENCY ANEMIA
Epidemiology
Iron deficiency is the most common nutritional deficiency in developing and developed countries. Data from the National Health and Nutrition Examination Survey (NHANES) indicate the prevalence of IDA in young children and women of childbearing age is 1.2% and 4.5%, respectively.2 The normal ranges for Hb and Hct are so wide that a patient may lose up to 15% of RBC mass and still have a Hct within the normal range. Therefore, iron deficiency may precede the appearance of anemia.
Iron Balance
The normal iron content of the body is about 3 to 4 g. Iron is a component of Hb, myoglobin, and cytochromes. About 2 g of the iron exists in the form of Hb, and about 140 mg exists as iron-containing proteins such as myoglobin. About 3 mg of iron is bound to transferrin in plasma, and 1,000 mg of iron exists as storage iron in the form of ferritin or hemosiderin. The rest of the iron is stored in other tissues such as cytochromes.9 Due to the toxicity of inorganic iron, the body has an intricate system for iron absorption, transport, storage, assimilation, and elimination. Hepcidin is a regulator of intestinal iron absorption, iron recycling, and iron mobilization from hepatic stores. It is a peptide made in the liver, distributed in plasma, and excreted in urine. Hepcidin inhibits efflux of iron through ferroportin. Hepcidin synthesis is increased by iron loading and decreased by anemia and hypoxia. Hepcidin is induced during infections and inflammation, which allows iron to sequester in macrophages, hepatocytes, and enterocytes.15 As a result, hepcidin is likely an important mediator of AI. Hepicidin is usually suppressed in IDA.16
Most people lose about 1 mg of iron daily. Menstruating women can lose up to 0.6% to 2.5% more per day. Pregnancy requires an additional 700 mg of iron and a blood donation can result in as much as 250 mg of iron loss;17 these patients are at higher risk for deficiency.
Iron is best absorbed in its ferrous (Fe2+) form. The normal daily Western diet contains mainly the ferric (Fe3+) nonabsorbed form. After iron is ionized by stomach acid and then reduced to the Fe2+ state, it is absorbed primarily in the duodenum, and to a smaller extent in the jejunum, via intestinal mucosal cell uptake. Subsequently, it is transferred across the cell into the plasma. Iron absorption is not directly proportional to iron intake. Rather as physiologic iron levels decrease, GI absorption of iron increases.
The daily recommended dietary allowance for iron is 8 mg in adult males and postmenopausal females and 18 mg in menstruating females. Children require more iron because of growth-related increases in blood volume, and pregnant women have an increased iron demand brought about by fetal development. In the absence of hemochromatosis, iron overload does not occur, because only the amount of iron lost per day is absorbed. The amount of iron absorbed from food depends on the body stores, the rate of RBC production, the type of iron provided in the diet, and the presence of any substances that may enhance or inhibit iron absorption.
Heme iron, which is found in meat, fish, and poultry, is about three times more absorbable than the nonheme iron found in vegetables, fruits, dried beans, nuts, grain products, and dietary supplements. Gastric acid and other dietary components such as ascorbic acid increase the absorption of nonheme iron. Dietary components that form insoluble complexes with iron (phytates, tannates, and phosphates) decrease absorption. Phytates, a natural component of grains, brans, and some vegetables, can form poorly absorbed complexes and partially explain the increased prevalence of IDA in poorer countries, where grains and vegetables compose a disproportionate amount of the normal diet. Polyphenols bind iron and decrease nonheme iron absorption when large amounts of tea or coffee are consumed with a meal. Although the mechanism is unknown, calcium inhibits absorption of both heme and nonheme iron. Finally, because gastric acid improves iron absorption, patients who have undergone a gastrectomy or have achlorhydria have decreased iron absorption.18
Etiology
Iron deficiency results from prolonged negative iron balance, which can occur due to increased iron demand or hematopoiesis, increased loss, or decreased intake/absorption. The onset of iron deficiency depends on an individual’s initial iron stores and the imbalance between iron absorption and loss. Multiple etiologic factors usually are involved. Certain groups at higher risk for iron deficiency include children younger than 2 years, adolescent girls, pregnant/lactating females, and those older than 65 years. Patients older than 65 years of age with IDA should be considered for testing for occult GI bleeding.17 Blood loss must initially be considered a cause of IDA in adults. Blood loss may occur as a result of many disorders, including trauma, hemorrhoids, peptic ulcers, gastritis, GI malignancies, arteriovenous malformations, diverticular disease, copious menstrual flow, nosebleeds, and postpartum bleeding. In less industrialized nations, the risk of IDA is largely related to dietary factors.
The USPSTF recommends routine screening for IDA in all pregnant women.19 The USPSTF has concluded that evidence is insufficient to recommend for or against routine iron supplementation for nonanemic pregnant women.17 However, iron deficiency in pregnant women is so common that the Centers for Disease Control and Prevention (CDC) guidelines recommend initiation of low-dose iron supplements or prenatal vitamins with 30 mg/day of iron at each woman’s first prenatal visit.
Medication history, specifically regarding recent or past use of iron, alcohol, corticosteroids, warfarin or other anticoagulants, aspirin, and nonsteroidal antiinflammatory drugs (NSAIDs), is a vital part of the history to assess bleeding risk. Other possible causes of hypochromic microcytic anemia include AI, thalassemia, sideroblastic anemia, and heavy metal (mostly lead) poisoning (Fig. 80–4).
Pathophysiology
Iron is vital to the function of all cells. Without iron, cells lose their capacity for electron transport and energy metabolism. Iron deficiency usually is the result of a long period of negative iron balance. Manifestations of iron deficiency occur in three stages. In the initial stage, iron stores are reduced without reduced serum iron levels and can be assessed with serum ferritin measurement. The stores allow iron to be utilized when there is an increased need for Hb synthesis. Once stores are depleted, there still is adequate iron from daily RBC turnover for Hb synthesis. Further iron losses would make the patient vulnerable to anemia development. In the second stage, iron deficiency occurs when iron stores are depleted, and Hb is above the lower limit of normal for the population but may be reduced for a given patient. This can be determined by serial CBC measurements. Findings include reduced transferrin saturation and increased TIBC. The third stage occurs when the Hb falls to less than normal values.
Laboratory Findings
Abnormal laboratory findings for patients with IDA generally include low serum iron and ferritin levels and high TIBC. In the early stages of IDA, RBC size is not changed. Low ferritin concentration is the earliest and most sensitive indicator of iron deficiency. However, ferritin may not correlate with iron stores in the bone marrow because renal or hepatic disease, malignancies, infection, or inflammatory processes may increase ferritin values.9 Hb, Hct, and RBC indices usually remain normal in early stages.
In the later stages of IDA, Hb and Hct fall below normal values, and a microcytic hypochromic anemia develops. Microcytosis may precede hypochromia, as erythropoiesis is programmed to maintain normal Hb concentration in preference to cell size. As a result, even slightly abnormal Hb and Hct levels may indicate significant depletion of iron stores and should not be ignored. In terms of RBC indices, MCV is reduced earlier in IDA than Hb concentration.
Transferrin saturation (i.e., serum iron level divided by the TIBC) is useful for assessing IDA. Low values may indicate IDA, although low serum transferrin saturation values also may be present in inflammatory disorders. The TIBC may help to differentiate the diagnosis in these patients: TIBC >400 mcg/dL (71.6 mol/L) suggests IDA, while values <200 mcg/dL (35.8 mol/L) usually represent inflammatory disease.
TREATMENT
Iron Deficiency Anemia
Desired Outcomes
The outcomes for all types of anemia in this chapter include: reversal of hematologic parameters to normal, return of normal function and quality of life, and prevention or reversal of long-term complications such as neurologic complications of vitamin B12 deficiency.
Dietary Supplementation and Oral Iron Preparations
The severity and cause of IDA determine the approach to treatment. Treatment is focused on replenishing iron stores. Because iron deficiency can be an early sign of other illnesses, treatment of the underlying disease may aid in the correction of iron deficiency.
Treatment of IDA usually consists of dietary supplementation and administration of oral iron preparations. Foods high in iron are listed in Table 80–1. Iron is best absorbed from meat, fish, and poultry. These foods as well as certain iron-fortified cereals can help treat IDA. Orange juice and other ascorbic acid-rich foods can be included with meals to increase absorption. Milk and tea reduce absorption and should be consumed in moderation. In most cases of IDA, oral administration of iron therapy with soluble Fe2+ iron salts is appropriate.
TABLE 80-1 Good Sources of Iron
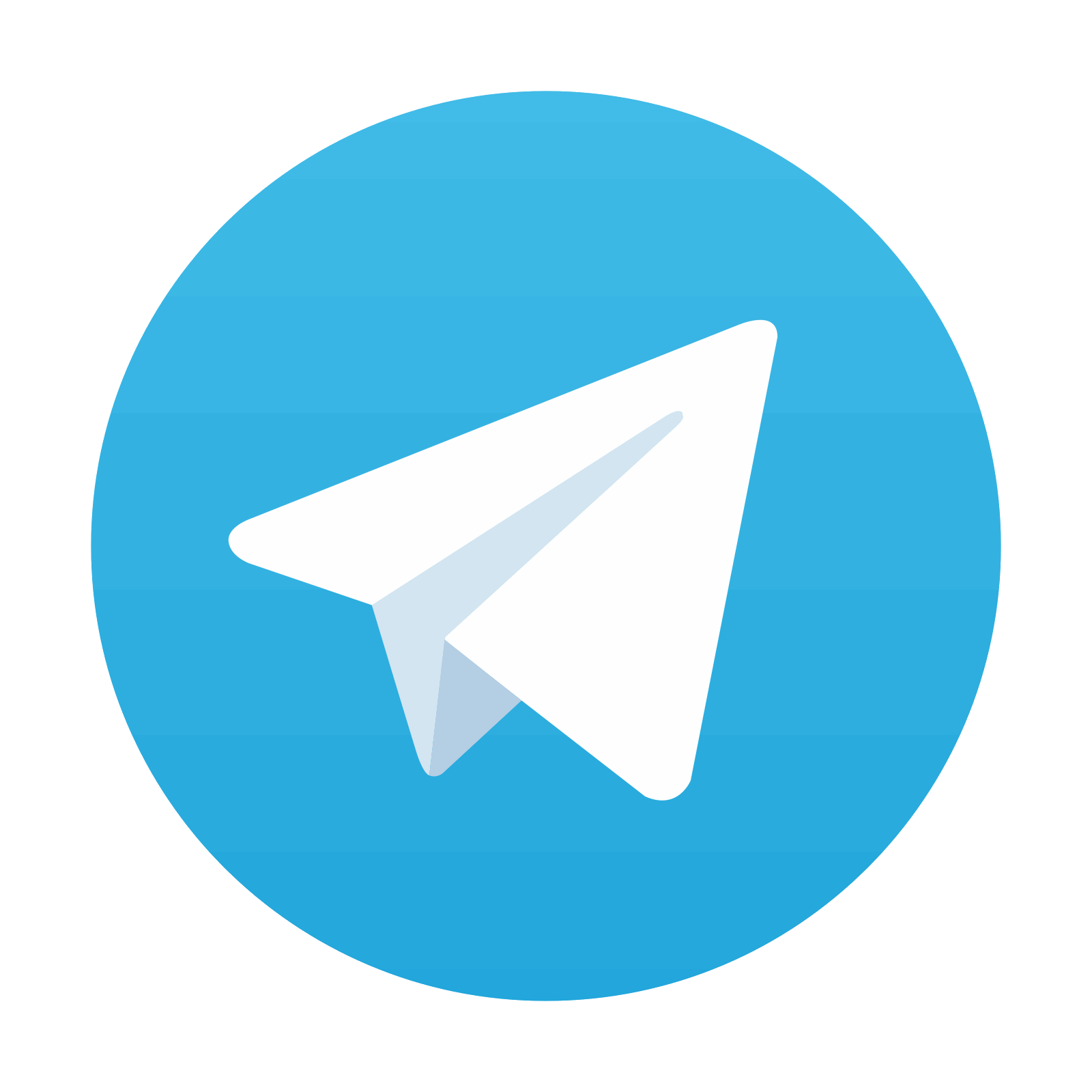