Figure 48-1. Arterial blood supply to the duodenum.
Following its embryologic origins, the vascular supply to the duodenum arises from branches of the celiac trunk for the foregut portion, whereas the distal (midgut origin) duodenum is supplied by branches of the SMA (Fig. 48-1). Venous drainage includes a series of pancreaticoduodenal veins which drain into the SMV–portal vein system. Lymphatic drainage follows the vascular supply with drainage to the pancreaticoduodenal nodes. From here, lymph drains superiorly to the hepatic nodes or inferiorly to the superior mesenteric nodes.
JEJUNUM AND ILEUM
2 Distal to the ligament of Treitz, the jejunum and ileum form the remainder of the small intestine. The boundary between the two is arbitrarily determined such that 40% of the intraperitoneal small intestine comprises jejunum and 60% comprises ileum. This portion of the bowel is suspended within the peritoneal cavity by a thin, broad-based mesentery that is attached to the posterior abdominal wall. The jejunum and ileum are freely mobile within the peritoneal cavity. The jejunum is the widest portion of the small intestine, whose caliber progressively decreases as it approaches the ileocecal valve. The mucosa of the jejunum has a thick lining and is characterized by prominent plicae circulares that become shorter and less frequent in the ileum. The total length of jejunum and ileum varies, but is usually between 5 and 7 m in length. The small intestine terminates in the right lower quadrant at the ileocecal valve. The ileocecal valve exhibits motor characteristics separate from the terminal ileum and colon, postulated to prevent reflux of fecal material from the colon into the small intestine.2
The arterial blood supply of the jejunum and the ileum arises from the SMA. The main vascular branches form arcades within the mesentery. The vasa recta, intestinal arterial branches, enter into the intestinal wall without anastamosing. The vasa recta of the jejunum are straight and long, in contrast to the vasa recta of the ileum, which are shorter with greater arborization (Fig. 48-2). The venous and lymphatic drainage follow the arterial supply. The main venous outflow is through the SMV which, along with the splenic vein, becomes the portal vein.
Figure 48-2. The superior mesenteric artery supplies bloodflow into the small intestinal arteries (vasa recta) which branch within the mesentery. In the jejunum (A), the vasa recta are straight and long, this is in contrast to the vasa recta within the ileum (B), which are shorter with greater arborization.
MICROSCOPIC ANATOMY
General Considerations
3 The wall of the small intestine is composed of four distinct layers—mucosa, submucosa, muscle, and serosa. The role of the mucosa is absorption and secretion. The luminal mucosal surface forms circular folds known as plica circularis or valvulae conniventes in all segments of the small intestine distal to the first portion of the duodenum. The submucosa contains an elaborate network of blood vessels, lymphatics, and nerves. The muscular portion of the wall includes outer longitudinal and inner circular muscle layers. Between the muscle layers lies the myenteric (Auerbach) plexus. The muscular layers are responsible for coordinating peristaltic movements. The outermost layer, the serosa, is composed of a thin layer of mesothelial cells overlying loose connective tissue. The serosa covers only the anterior surface of the retroperitoneal segments of small bowel, but it completely covers the portions of small bowel that are invested with mesentery.
Mucosa
The mucosa lines the luminal surface of the small intestine. It consists of three layers: epithelial cells, lamina propria, and a narrow layer of smooth muscle, the muscularis mucosae. The basic structural unit of the mucosa is the crypt and villus. Villi are finger-like projections of mucosa 0.5 to 1 mm high extending into the intestinal lumen that have a columnar epithelial surface and a cellular connective tissue core of lamina propria. Each villus contains a central lacteal (lymphatic), and a vascular network consisting of a small artery, vein, and capillaries. Ninety percent of the cells of the villi are columnar epithelial cells responsible for absorption and secretion. These cells are 22 to 25 μm high with basally located nuclei. The apices of these cells have microvilli, produced by numerous folds in the apical membrane, which account for the brush border appearance. Microvilli are approximately 1 μm long and 0.08 μm wide. Their surface is coated by glycoproteins rooted in the cell membrane. These glycoprotein filaments are referred to as the glycocalyx and are essential for digestion and absorption.3 The lateral membranes of neighboring enterocytes are connected by tight junctions, an apparent fusion of adjoining plasma membranes just below the level of the brush border. Movement of ions and water can occur by either a transmembrane or a paracellular route through tight junctions, which behave as selective pores.
Between the villi lie the crypts of Lieberkühn. Stem cells within the crypts of Lieberkühn are the source of the four major types of differentiated cells: the absorptive enterocyte, goblet cells, enteroendocrine cells, and Paneth cells. Absorptive enterocytes differentiate as they migrate from the crypt compartment up toward the tip of the intestinal villus. Cells then undergo apoptosis and are shed into the intestinal lumen. Most of the intestinal lining is renewed over a period of approximately 5 days. Despite the rapid rate of cellular turnover, intestinal epithelial cells exhibit complex patterns of gene expression that vary according to their location on the two main spatial axes of the gut, the vertical (crypt-villus) and horizontal (proximal to distal) axes. For example, cells destined to become enterocytes do not begin to express a variety of genes important in digestion and absorption until the cells have migrated out of the crypt and up the villus. In addition, many epithelial cell genes are selectively expressed in the proximal small intestine, whereas other genes are specifically expressed only in the ileum.4
Several other cell types are present in the mucosa. Mucus-secreting goblet cells are present in both the crypts and villi. Goblet cells have a narrow base containing the nucleus and a wide apical membrane with a large number of granules containing mucin. Mucin is secreted in a merocrine fashion by the goblet cell and functions as a lubricant and has a cytoprotective function.5
Paneth cells are pyramidal cells that reside in the crypt base. They contain large eosinophilic secretory granules located at their apical surface. It is thought that Paneth cells play a role in host defense based on their abundant expression of lysozyme and defensins, a family of small peptides that are also found in human neutrophils and exhibit microbicidal activity toward many different bacterial organisms in vitro. However, examination of the role of Paneth cells in the small intestine by lineage ablation in transgenic mice revealed no alteration in host defense mechanisms, thus the actual function of Paneth cells are yet to be delineated.6
Enteroendocrine (also referred to as amine precursor uptake and decarboxylation, or APUD) cells may reside in either the crypts or the villi, depending on the particular neuroendocrine substance they produce. Specific areas of the small intestine have higher concentrations of specific neuroendocrine substances than other areas. These cells do not contact the intestinal lumen, unlike exocrine cells, and their secretory granules are located below the nucleus near the basement membrane. This suggests that these cells secrete their contents into the circulation rather than into the intestinal lumen.
Submucosa
The submucosa is a dense connective tissue layer with a rich network of blood vessels, nerves, and lymphatics. The submucosa contains Meissner plexus and is the strongest layer of the intestinal wall. Brunner glands are found in the submucosa of the duodenum and secrete mucus and bicarbonate into the intestinal lumen. These secretions aid in the neutralization of the gastric acid load which enters the duodenum. Peyer patches are localized collections of lymphoid follicles that are most prominent in the submucosa of the ileum. These are typically 8 to 10 mm in diameter and are most abundant early in life, gradually disappearing with age.
Physiology
4 The small intestine plays important physiologic roles in motility, blood flow, growth, digestion, absorption, immune function, and endocrine secretion. In fact, the small intestine is the largest endocrine organ in the human body.7 The secretion of numerous hormones and neurotransmitters are specific to distinct anatomic zones within the small intestine (Fig. 48-3). There is no specific cell mass which produces these hormones, but rather individual cells scattered along the gastrointestinal tract.
Gastrin is a peptide produced in the gastric antrum and in the duodenum by the G cells and secreted into the circulation in response to gastric distension, vagal stimulation, amino acids, and hypercalcemia. Gastrin exists in three functional forms (G-34, G-17, and G-14). Its release is inhibited by low intraluminal pH, somatostatin, secretin, gastric inhibitory peptide (GIP), vasoactive intestinal peptide (VIP), glucagon, and calcitonin. Gastrin binds to CCK2/gastrin receptors on ECL cells causing a release of histamine which in turn stimulates the parietal cells in a paracrine fashion. Gastrin also causes an increase in the gastric blood flow and the release of pepsinogen by the chief cells and pancreatic enzymes from the pancreatic centroacinar cells.
Figure 48-3. Distribution of peptide hormones in the gastrointestinal tract.
Cholecystokinin (CCK) is produced in the proximal two-thirds of the small intestine by the I cells and released into the gut lumen. It has 4 main forms (CCK-58, CCK-39, CCK-33, and CCK-8). It shares the final five amino acids adjacent to the C-terminus with gastrin, and this accounts for its hormonal activity. Stimulation of I cells by amino or fatty acids causes release of CCK, this in turn stimulates contraction and emptying of the gallbladder, increases bile flow, causes relaxation of the sphincter of Oddi, and stimulates pancreatic enzyme secretion. CCK also has trophic effects on the small intestinal mucosa and pancreas.
Secretin, discovered as the first gastrointestinal hormone in 1902, is a 27 amino acid peptide produced by the S cells of the duodenum and jejunum. Secretin is released into the circulation and intestinal lumen in response to low intraluminal pH, fatty acids, and bile salts. Water and bicarbonate are secreted by the pancreas in response to secretin. Bicarbonate is also released from the biliary ductal epithelium and Brunner glands in response to secretin. In turn, pancreatic enzymes are released from the pancreas. The increased pH and the presence of pancreatic enzymes aid in the digestion of lipids. The increased pH also provides a negative feedback loop to inhibit further production of secretin. Secretin produces a paradoxical release of gastrin in patients with gastrinomas, but does not produce this effect on normal individuals.
Somatostatin is a peptide hormone consisting of 14 or 28 amino acids and is produced by D cells in the pancreatic islets, gastric antrum, and duodenum. Its primary role is inhibition of other gastrointestinal hormones and inhibition of pancreatic, biliary, and gastric secretion. In addition, somatostatin decreases splanchnic and portal blood flow. Somatostatin is stimulated by the presence of fat, proteins, acid, glucose, amino acids, and CCK. Octreotide, a long-acting synthetic somatostatin analog, is used in the treatment of variceal bleeding, hormone secreting neuroendocrine tumors, carcinoid syndrome, and enterocutaneous and pancreatic fistulas.8
Gastrin-releasing peptide, the mammalian homolog of bombesin, is a 27 amino acid peptide which is produced by the small intestine and is released in response to vagal stimulation. It is a prostimulatory molecule which causes the release of most gastrointestinal hormones, with the exception of secretin. It also has a promotility effect and stimulates endothelial proliferation. Gastric inhibitory peptide or glucose-dependent insulinotropic peptide (GIP) is a 42 amino acid peptide produced by the K cells of the duodenum and jejunum. It is released in response to glucose, fat, protein, and adrenergic stimulation. It stimulates secretion of insulin and inhibits gastric acid and pepsin production. Type 2 diabetics are resistant to the effects of GIP.
Motilin is a 22 amino acid peptide produced by the enteroendocrine cells in the duodenum and jejunum. Release of motilin occurs during the interdigestive and fasting periods. Release may also be related to alkalinization of the duodenum. Motilin’s main function is to stimulate the migrating myoelectric complex. Motilin agonists such as erythromycin, are used clinically as stimulants of gastrointestinal motility.
VIP is a 28 amino acid peptide which is produced by gastrointestinal tract neurons. It serves as a neurotransmitter stimulating pancreatic exocrine and intestinal secretion. Conversely, it has an inhibitory effect on gastric acid secretion. VIP is a potent vasodilator and relaxant of smooth muscle.
Neurotensin is a 13 amino acid peptide that is produced by the N cells primarily in the ileum, but also in the proximal small intestine and colon in response to the presence of intraluminal fat. It stimulates pancreatic bicarbonate secretion and intestinal motility. Neurotensin also serves as a trophic factor on the small intestinal mucosa and inhibits gastric secretion.
Pancreatic glucagon and enteroglucagon are 29 and 37 amino acid peptides produced by the α-islet cells of the pancreas and the L cells of the small intestine, respectively. Pancreatic glucagon is released in response to low serum glucose and subsequently induces glycogenolysis, lipolysis, gluconeogenesis, and ketogenesis. Enteroglucagon is released in response to the presence of a mixed meal and inhibits gastric emptying and small bowel motility.
Motility
5 The coordination of movements of the gastrointestinal tract is necessary for the proper digestion of food. Well-timed contraction and relaxation patterns are initiated in gastrointestinal nervous system causing coordinated electrical activity and muscular movements. This may be influenced by both internal and external factors. The small intestine has motor functions which are distinct from the other parts of the gastrointestinal tract. The intrinsic nerves (myenteric or Auerbach plexus) provide the basis for coordinating the circular and longitudinal smooth muscles of the small intestine. Extrinsic sympathetic (epinephrine) stimulation slows motility while parasympathetic (acetylcholine) stimulation increases motility.
The intrinsic electrical activity of the small intestine is based on the intestinal smooth muscle normal resting potential. This normally is –50 to –70 mV and is maintained by Na+-K+-ATPase activity.9 The resting potential varies by 5 to 15 mV and results in a phasic depolarization which is referred to as slow waves, basic electrical rhythm, or pacemaker potential. These depolarizations occur at regular intervals of approximately 11 to 13 times per minute in the duodenum and decrease to 8 to 10 times in the ileum, but do not directly lead to muscular contractions. The electrical activity is coupled to muscular contraction at the level of gap junctions, which are low resistance cell-to-cell connections. These gap junctions become less regular in the midjejunum and in the distal small intestine. This causes slowing of the frequency of contractions distally, allowing for absorption of more slowly digested intestinal contents, including fats and bile salts.
Spike potentials represent a second mode of electrical stimulation of the small intestine. Spike potentials result in rapid depolarization of the membrane potential. Repeated bursts of spike potentials cause a short area of contraction. In contrast to the always present slow waves, spike potentials occur at discrete time intervals. The coordination of a slow wave and a spike potential leads to the initiation, duration, and frequency of rhythmic migratory small intestinal contractions.
During the interdigestive period between feeding, the small intestine follows a well-defined rhythmic pattern. This pattern consists of muscular contractions that migrate from the stomach or duodenum, continue on to the terminal ileum, and are regulated by the migrating motor complex (MMC). This pattern can be broken down into four distinct phases. Phase I is a period of relative quiescence; phase II is a period of accelerated irregular electrical spiking and muscular activity; phase III is a series of high-amplitude rapid electrical spikes corresponding to rhythmic gut contractions; and phase IV is a period of subsiding activity. This process occurs over a period of 90 to 120 minutes and progresses from the proximal small bowel and terminates in the ileum. Once the MMC reaches the terminal ileum, the process starts over again in the proximal small bowel. The circular muscles provide segmental contraction over a 1-cm length of small intestine. These contractions occur approximately 11 to 13 times per minute in the duodenum and decrease to 8 to 10 times in the ileum. This creates functional compartments where prolonged exposure to the mucosa and mixing of the intestinal chyme occurs, aiding the process of digestion and absorption. The circular muscles are also responsible for the peristaltic waves which propagate regularly at a rate of 1 to 2 cm/sec. These regular waves may be interspersed with rushes of contractions followed by periods of no motor activity. This rate becomes progressively slower in the distal small intestine. These peristaltic movements serve as a method of propelling chyme through the length of the small intestine. The total transit time from the duodenum to the terminal ileum is approximately 220 minutes (+/–53 minutes).10 Serum motilin levels have been found to mirror the activity of the MMC. Exogenous motilin administration has been found to increase MMC activity.
During and immediately following times of feeding, the intestinal movements are not rhythmic, with complexes of peristaltic and antiperistaltic contractions. This is thought to be a disruption of the MMC from bolus feeding. This seemingly random pattern of movements allows for effective mixing of chyme. Hormonal changes are thought to play a role in this process. Physiologic doses of neurotensin, insulin, gastrin, and CCK cause an alteration in the MMC similar to bolus feeding. Visual and olfactory feeding cues can also cause a disruption of the MMC. The MMC is more significantly inhibited by fatty meals as opposed to protein or carbohydrate meals of similar caloric value. The intrinsic nervous plexus or Meissner plexus innervating the submucosa helps to regulate mucosal absorption and secretion, but has no control on motility.
IMMUNOLOGY
Principles of Gut Immunology
6 The lumen of the gastrointestinal tract is connected to the outside environment and comes in direct contact with many potentially pathogenic microorganisms. Lymphocytes, macrophages, polymorphic granulocytes and other cells that take part in immune response are distributed throughout the whole gut. The commensal microflora have many benefits to the host by supporting digestion and keeping the appropriate balance among different microbial species.11 The immune system is highly effective at responding selectively to invading pathogens, yet on the other hand tolerating a much larger number of harmless food antigens and commensal organisms. Many bacteria, viruses and parasites are digested and enter the small intestine every day.12 Consequently, the small intestine needs a complex defense mechanism to battle against these exposures in different ways.13
While the immune system in the small intestine is important for host defense, other mechanisms within the small intestine also participate in host defense. Proteolytic and lipolytic enzymes are produced in high concentrations by extraintestinal cells in the pancreas and degrade different pathogenic agents at an early phase of digestion. In addition, mucin is produced by the enterocytes which is cytoprotective and inhibits bacterial growth. The small intestine can actively increase peristalsis functions to mechanically get rid of pathogenic agents, which may be potentially dangerous to the gut. In addition, tight junctions between epithelial cells prevent penetration of bacteria in between cells. Potential pathogens vary greatly in size, from very small viruses that are nanometer in size, to parasites such as helminths that are macroscopically visible and quite large. To put this into the broader picture of evolution, a large range of defense mechanisms is essential for survival of each individual.
The primary cellular barrier in the gut that prevents antigens from encountering the immune system is the single layer of gut epithelium. The total surface area of the small intestine is 400 m2, due not only to intestinal length but also due to the formation of millions of villi in the small bowel which contribute significantly to the overall surface area.14 In the upper small intestine, the bulk of antigen exposure comes from the diet, whereas in the ileum and colon, the additional antigenic load of an abundant and highly complex commensal microflora is prevalent. The epithelial cells of the gut mucosa have developed features that make the intestinal epithelium an active immunologic as well as anatomic barrier. For example, these nonclassical immune cells express major histocompatibility complex (MHC) class I and II molecules, consistent with their ability to participate in adaptive immune recognition of pathogenic bacteria. Small intestinal epithelial cells also express toll-like receptors on their apical surface that enables them to detect bacterial products and to initiate an innate immune response. Antigen-presenting dendritic cells (DCs) also send processes between gut epithelial cells without disturbing tight junction integrity and sample commensal and pathogenic gut bacteria.15,16 The gut epithelial barrier therefore represents a highly flexible structure that limits antigens from entering the systemic body.
The gut-associated immune system represents one of the largest immunologic compartments in the body. Lymphoid tissue in the gastrointestinal tract is a major part of the whole-body immune system and consists of both aggregated (lymphoid follicles, Peyer patches) and nonaggregated (luminal, intraepithelial and in lamina propria) immune cells. Under physiologic circumstances, oral administration of protein antigens induces systemic unresponsiveness when the same antigen is given parenterally (a phenomenon known as oral tolerance). In animal models, oral tolerance appears to be a specific consequence of the immune environment in the gut, which favors the generation of regulatory T cells.
Nonaggregated and Aggregated Lymphoid Tissue
The gut epithelium and its mucous layer form a major barrier to trap pathogens which are then eliminated when the gut epithelium is shed and replaced by new cells deriving from stem cells in the crypts. Several different cell types face antigenic exposure present in the intestinal lumen and include the epithelium, neutrophils, lymphocytes, and macrophages. These luminal cells represent an initial effector mechanism in the first front directed toward an antigenic exposure. In addition, there are also immigrated intraepithelial lymphocytes, which are found in between epithelial cells beneath the tight junctions. Local defense depends in part on these T lymphocytes that are nestled among gut epithelial cells. These T cells modulate homeostasis of the gut epithelium through local production of cytokines and have cytolytic effects. In fact, the number of intraepithelial lymphocytes can increase dramatically in response to inflammation or infection. After exposure to antigens, these cells reenter the circulation to initiate a systemic immune response. The lamina propria contains nonaggregated, diffusely distributed lymphoid tissue, including different types of immune cells (Fig. 48-4). B cells in the lamina propria undergo cytokine-induced differentiation to become active producers of immunoglobulin A (IgA) that is secreted into the gut lumen. T cells in the lamina propria may have a different role in a helper-inducer function for immunoglobulin production rather than a cytolytic function. Mast cells and eosinophils are also present in the lamina propria in small numbers. They exhibit an important role in allergic and hypersensitivity reactions as well as defense against parasites. Parasites including worms are recognized and tagged using the monovalent immunoglobulin E (IgE) which is mostly bound to host cell surfaces. Actual parasite killing is depending on toxic proteins secreted by eosinophils.
There are also large aggregates of lymphoid tissue found in the small intestine. These so-called Peyer patches are localized collections of lymphoid follicles that are most prominent and macroscopically visible in the submucosa of the ileum. Peyer patches are most prominent in children and gradually disappear with age. Along with the nonaggregated immune system, the enormous quantity and diversity of antigens present within the gut are also processed via Peyer patches in the lamina propria and the mucosa-associated lymphoid tissue (MALT), which in total contains about 70% of the body’s lymphoid cells. Another often used term to describe the immune system within the small intestine is gut-associated lymphoid tissue (GALT). Peyer patches do not have any villi, and so-called microfold (M) cells concentrated within the epithelium allow the selective uptake of food antigens and microorganisms (Fig. 48-5). M cells are specific cells in the intestinal epithelium over lymphoid follicles that endocytose antigens. M cells transport them into the underlying tissue, where they are taken up by local DCs and macrophages.17 DCs and macrophages that receive antigens from M cells present them to T cells in the GALT, leading ultimately to appearance of IgA-secreting plasma cells in the mucosa. B-lymphocyte precursors proliferate in germinal centers within the Peyer patches. DCs below the epithelium can also take up luminal antigens by pushing pseudopods up between epithelial cells.
Immunoglobulin Secretion
At an early level of exposure at the gut epithelium, M cells take up antigen and transport it transcellularly using an endocytic mechanism into the underlying lymphoid tissues of Peyer patches. Lymphocyte activation ensues, and these activated lymphocytes migrate into afferent lymphatics, pass through mesenteric lymph nodes, and enter the circulation through the thoracic duct. During this process, the lymphocytes mature into B and T lymphoblasts with an enriched population of IgA-producing B cells.18 Passage of viable bacteria from the intact gastrointestinal tract to the mesenteric lymph node and beyond has been termed bacterial translocation, possibly explaining septic complications and multiple organ failure in peritonitis, burn and trauma patients. The immunogenic integrity of the mucosa is significantly damaged by systemic injury of different kinds. A major protective mechanism of the intestinal immune system is synthesis and secretion of IgA. In the intestine, IgA exists as a dimer that is linked with two additional molecules – the J chain – linking two IgA molecules, and the polymeric immunoglobulin receptor, which transports the IgA complex across the cell and allows release of the complex, termed secretory component, into the intestinal lumen. The transmembranous immunoglobulin receptor is produced by the intestinal epithelial cell. It is thought that the secretory component may prevent proteolytic degradation of the IgA molecule and may stabilize the structure of the polymeric IgA complex in an environment containing numerous proteolytic enzymes and bacteria that would otherwise rapidly degrade it. Unlike IgG and IgM, IgA does not activate complement and does not promote cell-mediated opsonization. The major function of secretory IgA in host defense is protection against bacteria, viruses, and luminal antigens. Secretory IgA inhibits the adherence of bacteria to epithelial cells. It is well known that a breast-feeding mother transfers secretory IgA to her nursing infant, protecting the infant from bacteria and viruses that were originally present in the mother’s gastrointestinal tract.
Figure 48-4. Small intestinal villus with associated immunologic cells.
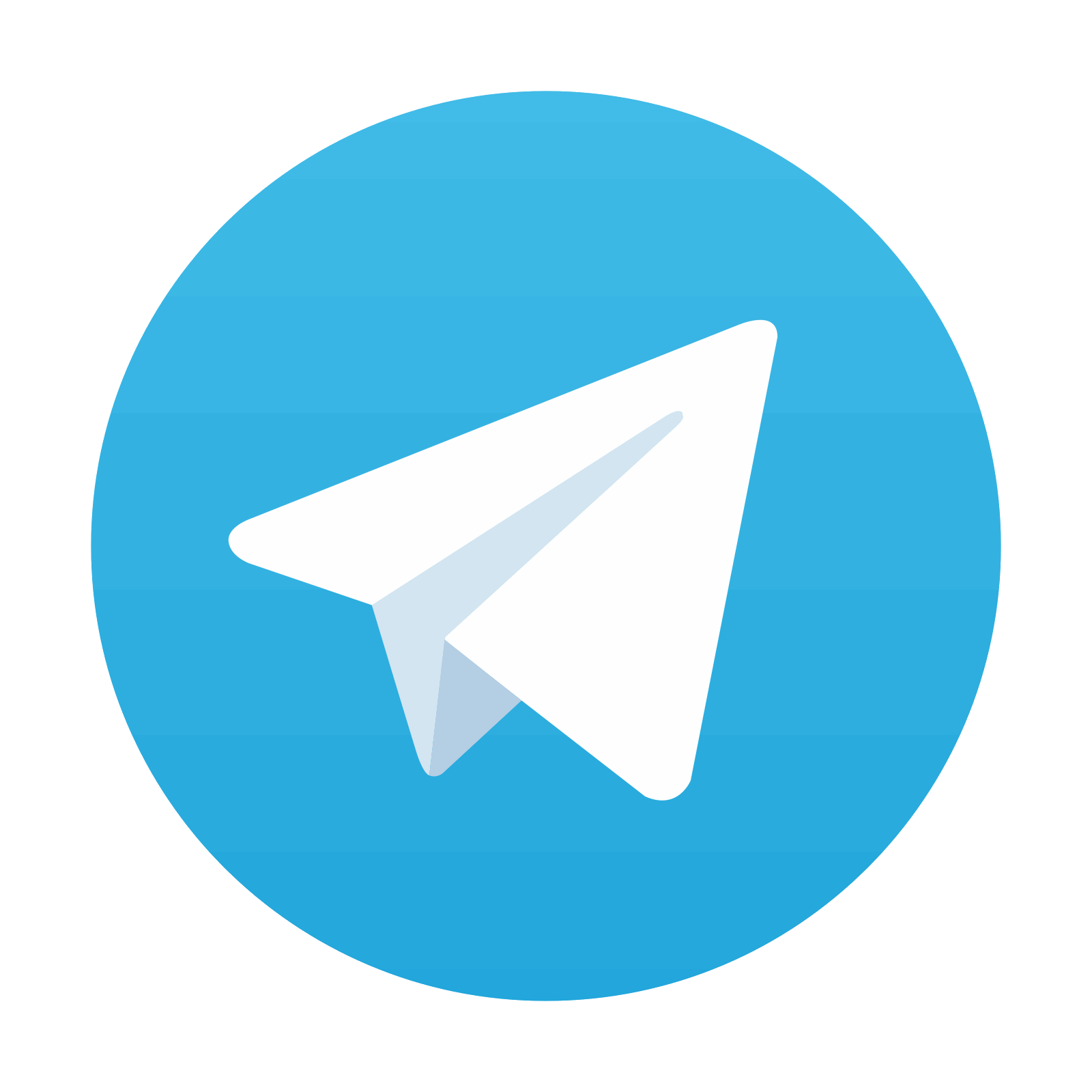
Stay updated, free articles. Join our Telegram channel

Full access? Get Clinical Tree
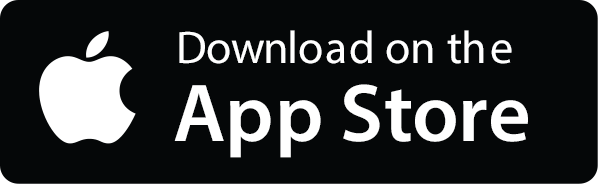
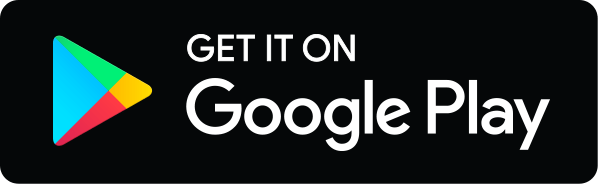