Fig. 8.1
Wounds close partly by contraction and regeneration in the tadpole; however, they close by contraction and scar formation in the adult frog. Photos of healing of full-thickness excisional skin wounds (defects) in tadpoles and frogs are from the study described in Fig. 8.2 and in the text. (Yannas et al. 1996) Top left: Full-thickness skin defect in tadpole, day 1. Top right: Although the tadpole wound contracted significantly, it closed partly by regeneration. Area enclosed with broken line in tadpole: The regenerated skin has spots that are native to the skin of this species (North American bullfrog). Day 97. Bottom left: Full-thickness skin defect in adult frog, day 0. Bottom right: The frog wound closed partly by contraction and partly by scar formation. Area enclosed with broken line in adult frog: Contracted and scarred wound. Day 150. Identification of tissues as regenerated skin or scar was based on histological views (Yannas et al. 1996)

Fig. 8.2
Contraction dominated regeneration as a mode of wound closure with increasing development of the tadpole (larva). The broken line connecting the experimental data divides the wound closure diagram into three regions, each corresponding to a mode of defect closure: regeneration, contraction, scar formation. The percentage of each closure mode is indicated by the length of the ordinate inside the respective region. Regeneration and contraction, without scar formation, combined to close the dermis-free defects in the tadpole at four stages of development. Scar formation (indicated by the cross-hatched region at bottom right) was first observed after metamorphosis, the transition from tadpole to adult frog (Yannas et al. 1996).
Since scar formation was first observed after metamorphosis to the frog, contraction and regeneration were the only mechanisms for wound closure in the tadpole . In this model, contraction and regeneration appeared to be in a directly antagonistic relation in skin defect closure during development; contraction increased continuously at the expense of regeneration (Fig. 8.2) (Yannas et al. 1996).
8.2.2 Anatomical restriction to wound contraction during healing of the rodent ear by regeneration
In the rabbit, a sharp difference in healing outcome of dermis-free defects has been observed between two anatomical sites: the dorsal region and the ear. While the defect in the dorsal region closes by vigorous contraction and synthesis of scar, the defect in the ear (ear hole produced by punch) almost fails to contract. Instead, the skin in the ear regenerates completely, including the synthesis of hair follicles and sebaceous glands; scar has not been observed in the rabbit ear defect (Fig. 8.3) (Joseph and Dyson 1966; Goss 1980, 1992; Goss and Grimes 1972, 1975).

Fig. 8.3
Wound contraction in many rodents depends on the anatomical site of the injury. The skin of the ear in many rodents is firmly attached to the underlying cartilage and does not contract when injured. Regeneration, rather than closure by contraction, is observed following cutting a full-hole, 1 cm in diameter, in the rabbit ear. Top left: 1 day. Bottom left: 2 weeks. Top right: 4 weeks. Bottom right: 6 weeks. (From Goss 1980)
Contraction in the rabbit dorsal wound was estimated at 96 ± 1 % (percent total initial wound area closed by contraction), while the remainder is estimated as being scar (Kennedy and Cliff 1979) (Table 4.3). In contrast, contraction of the ear hole wound in the rabbit ear was estimated at only 3 ± 3 of total wound area while the remainder of the wound area closed by regeneration. The striking lack of contraction in the rabbit ear defect has been attributed to particularly tight binding of the skin to the underlying cartilage (Joseph and Dyson 1966; Goss 1980, 1992; Goss and Grimes 1972, 1975).
Total absence of wound contraction in the surgically injured rabbit ear was also directly observed in a related (but nonidentical) model of a rabbit cartilage wound, the dermal ulcer model (Mustoe et al. 1991). In this study, the full-thickness skin wound, 6 mm in diameter, was placed on one side of the ear only and the dermis was in contact with the avascular base of ear cartilage. Tattoo marks were placed at the wound perimeter on day 0 to measure the extent of contraction during healing. After 7 days, the diameters of wounds were unchanged and wound contraction was excluded as a variable in the experimental study. This finding confirmed that the absence of contraction was due to tight binding of skin on the ear cartilage (Fig. 8.4, Mustoe et al. 1991).

Fig. 8.4
Contraction was ruled out in this study of excision of skin from one side of the rabbit ear. Original drawing and photos are reproduced here. Left: Schematic of surgical model, showing the circular defect generated with a biopsy instrument in the rabbit ear, leading to excision down to the cartilage layer and monitored with a calibrated lens micrometer. EG epithelial gap, GTG granulation tissue gap, MH maximum height of granulation tissue. Photos a–c: Tattoo marks were placed at the wound periphery on day 0 to detect contraction. Arrows (barely visible in photos) demarcated the original borders of the 6-mm-diameter wound. C cartilage, E new epithelium, G new granulation tissue. a Day 0. b Partially healed, Day 7. No contraction. c Fully healed, day 14. Authors reported wound closure by new epithelium and new granulation tissue. They concluded that, following excision of a circular skin wound, the wound borders did not contract (photos by permission of Dr. Mustoe) (Mustoe et al. 1991)
In conclusion, scar formation was observed in a rabbit dorsal skin wound that closed primarily by contraction of the highly mobile integument at that anatomical site. In contrast, spontaneous regeneration of skin in the same species was observed in the wounded ear, in the absence of significant wound contraction. In these studies, lack of contraction of the dermis was confirmed by direct observation and was associated with restricted skin mobility at the local anatomical site.
8.2.3 Scarless Healing of Wounds in the Oral Mucosa of Adults
The oral (palatal) mucosa is often harvested for use as the equivalent of a skin graft in oral surgical procedures in humans . As with the harvesting of skin autografts, removal of mucosal grafts by excision leads to “donor” sites, i.e., deep wounds at the harvested site (Jung et al. 2013). It has been persistently reported that wounds in the oral mucosa of mice closed with greatly reduced scar or even lack of scar formation (Schrementi et al. 2008; Mak et al. 2009; Wong et al. 2009; Larjava et al. 2011; Glim et al. 2013).
Studies in oral mucosal wounds in the swine showed that the levels of TGFβ1 and TGFβ1 expression were lower than in control skin wounds (Schrementi et al. 2008). Another study of the wounded oral mucosa with the same swine model found evidence that oral mucosal wounds contracted significantly less than skin wounds (Mak et al. 2009) .
In conclusion, oral mucosal wounds contracted significantly less than skin wounds while also being relatively scarless. Such wounds also showed evidence of reduced expression of the cytokine which is required for differentiation of myofibroblasts, the contractile cells considered to be primarily responsible for wound contraction (Hinz et al. 2012) (Myofibroblast differentiation is described further briefly).
8.2.4 Scarless Healing in the Axolotl
Among vertebrates the adult axolotl (Ambystoma mexicanum) is uniquely capable to regenerate amputated limbs and other body parts (Lévesque et al. 2010), including full-thickness excisional skin wounds (Seifert et al. 2012) .
Studies of skin wound healing in the adult axolotl have shown that alpha smooth muscle actin (αSMA), a protein characteristic of myofibroblasts, was absent. TGFβ1, which is required for expression of the αSMA phenotype in fibroblasts, was only transiently expressed during wound healing (Lévesque et al. 2007, 2010).
The observed absence of myofibroblasts, contractile cells that drive contraction in wounds (Hinz et al. 2012), and the reduced presence of TGFβ1 are indirect evidence for reduction or absence of contraction in axolotl skin wounds .
8.3 Cell and Tissue Morphology During Spontaneous Wound Contraction
Contraction can be measured either as force or as deformation, the result of application of a balanced force. Measurements of contractile forces in wounds have appeared very rarely in the literature, a testament to the inherent difficulty in making such measurements. The contraction force required to close a skin wound in the rabbit thorax was measured to be about 0.1 N (Higton and James 1964).
In this section, we consider instead various approaches toward measuring deformation of tissues during wound healing (see Fig. 4.4). We focus, as before, on healing processes in the two organs that have been studied extensively in this context, i.e., skin and peripheral nerves. We begin by reviewing evidence that describes the cells responsible for wound contraction.
8.3.1 Assemblies of Oriented Contractile Cells and Tissue Deformation During Healing
Contractile fibroblasts , often referred to as myofibroblasts, have been originally credited with generating most of the contractile forces and tissue deformations in skin wounds (Gabbiani et al. 1971; Rudolph 1979; Rudolph et al. 1992; Gabbiani 1998; Daimon et al. 2013). Although other actin isoforms, such as smooth muscle γ-actin and skeletal muscle α-actin, have been implicated in force generation (Tomasek et al., 2013), the α-smooth muscle actin isoform is currently considered to be the most useful marker of the myofibroblastic phenotype (see Fig. 4.5) (Hinz et al. 2012). These cells are highly elongated and display densely bundled actin microfilaments at their perimeter. Perhaps the most widely used phenotype of myofibroblasts is “stress fibers,” filaments containing α-smooth muscle actin (Gabbiani et al. 1971; Desmoulière et al. 2005). Following wound closure, myofibroblasts disappear by apoptosis (Desmoulière et al. 1995; van Beurden et al. 2005). As we have done in earlier chapters, we will use the term myofibroblast to identify cells that have been shown to stain with an antibody to the α-smooth muscle actin isoform .
Identification of contractile cells in injured peripheral nerves has historically lagged behind the evidence for contraction with skin wounds. In an early study, contractile cells were identified in the uninjured perineurium (Ross and Reith 1969). Although myofibroblasts were individually identified in early studies with injured peripheral nerves, the role of these cells in the healing process was not investigated (Badalamente et al. 1985). Myofibroblasts, in the form of a thick sheet (capsule) surrounding peripheral nerve stumps resulting from transection, appear to have been first observed as late as 2000 (see Fig. 4.6) (Chamberlain et al. 2000a; Soller et al. 2012).
The differentiation of the fibroblast to the contractile fibroblast is regulated by transforming growth factor beta1 (TGFβ1), the ED-A splice variant of cellular fibronectin, and the presence of mechanical tension (Desmoulière et al. 2005). Although myofibroblasts are considered responsible for normal wound closure during healing of acute wounds in dermis, tendon, bone, cartilage, more recently in peripheral nerves, as well as in other injured organs, they are also considered primary actors in disfiguring scar contractures, as well as playing several other roles in pathological conditions (see Fig. 4.7) (Follonier Castella et al. 2010; Van De Water et al. 2013) .
Organized assemblies of myofibroblasts appear in contracting wounds, both in skin (Fig. 4.5) (Hinz and Gabbiani 2003) and (Fig. 4.6) peripheral nerves (Chamberlain et al. 2000a; Soller et al. 2012). Considering that a macroscopic force of about 0.1 N is required for skin wound closure in the rabbit (Higton and James 1964) and that the force generated by an individual cell is about 1–10 nN (Freyman et al. 2001a; Harley et al. 2008), it appears necessary to apply forces from as many as 108–109 cells during wound contraction to close a skin wound in the rodent. The required macroscopic force appears to be generated by assemblies of myofibroblasts, organized to apply cooperative forces and provide for a “mechanically coherent” wound (Yannas 1998).
Assemblies of contractile cells are known to be held together by stress fibers in neighboring myofibroblasts that are joined together at the sites of cadherin-type intercellular adherens junctions (AJs) (Hinz et al. 2004). AJs are dense plaques found under the plasma membrane of fibroblasts in granulation tissue of wounds as well as in scar-like tissues (Welch et al. 1990). Maturation processes take place in AJs during healing; for example, it has been shown that fibroblasts change cadherin expression from N-cadherin in early wounds to OB-cadherin in wounds undergoing contraction (Hinz et al. 2004). Intercellular mechanical coupling of stress fibers via AJs improves contraction of collagen gels by myofibroblasts. There is evidence that local contractile events are mechanically coordinated by AJs, via synchronization of periodic intracellular Ca2+ oscillations between physically contacting myofibroblasts (Follonnier et al. 2008, 2010; Goodbout et al. 2013). It appears very likely that these coordinated associations among myofibroblasts are responsible for generation of macroscopic contraction forces across a closing wound .
Orientation of long axes of contractile cells is a distinctive feature of such assemblies. Axial orientation appears to be mechanically required for generation of a directed macroscopic contractile force. This feature was demonstrated in vitro, with fibroblasts incubated inside a collagen scaffold, applying forces to the struts of the matrix along their long axis, causing them to buckle (Freyman et al. 2001b; Harley et al. 2008). In the guinea pig skin wound, the long axes of myofibroblasts have been shown, based on transmission electron microscopic observation, to be highly oriented in the plane of the epidermis (Murphy et al. 1990). In an earlier study, myofibroblasts showed cytoplasmic microfilaments that were aligned along the direction of their long axes; in this study, contractile cells were aligned parallel to the plane of the epidermis and the applied macroscopic forces appeared to be consistent with the observed reduction in wound area in the plane of the epidermis (Baur et al. 1984). The orientation of long axes of myofibroblasts inside a contracting skin wound in the guinea pig (Fig. 8.5) is consistent with these observations. The simplest mechanical field that corresponds to such an array of cells is the plane stress field.

Fig. 8.5
Orientation of myofibroblasts on the surface of a contracting skin wound in the guinea pig (day 10). Myofibroblasts (MFB) were stained red-brown with an antibody to alpha smooth muscle actin, their characteristic phenotype. (From Troxel 1994)
Contractile forces in skin wounds are applied not only in the plane of the epidermis but also out of plane. Observations of deformation of subdermal tissues in a closed skin wound in the guinea pig support the existence of mechanical forces that act immediately below the scar (Fig. 8.6; see area at bottom right labeled deformed subdermal tissue). This cross-sectional view of an initially dermis-free defect in the guinea pig skin shows the final stage of defect closure. In this micrograph, closely apposed dermal edges are separated by a small mass of scar. The micrograph obtained with polarized light shows highly deformed birefringent fibrous tissues, almost certainly stretched collagen fibers, connecting the proximal adipose layer at the base of the wound with distal scar mass (Troxel 1994). The photographic evidence of mechanical tension inside the closed-skin defect highlights the importance of subdermal tissues during wound closure (Fig. 8.6). There is a sharp contrast between these compliant subdermal tissues in the guinea pig wound and the dermis in the rabbit ear wound model which adheres tenaciously to the underlying cartilage layer (Joseph and Dyson 1966; Goss 1980, 1992; Goss and Grimes 1972, 1975; Mustoe et al. 1991). This comparison suggests the possibility that the mechanical compliance of subdermal tissues is a significant factor in the magnitude of contraction forces generated in skin wounds.

Fig. 8.6
Deformation of subdermal tissues in a contracting skin defect in the guinea pig. Observed by polarized light microscopy following spontaneous closure of an excisional full-thickness skin defect in the guinea pig. Left, right: Dermal edges are separated by scar. Top center: Scar. Bottom right: Highly deformed birefringent fibers (probably stretched collagen fibers) underneath scar and adipose layer. Bar: 500 µm. (From Troxel 1994)
Mechanical forces that contribute to wound closure in skin are not limited to those that deform by contraction. Observations made with guinea pig skin wounds have shown that other tissue movements, including an initial expansion of the dermal edges, as well as translational perilesional movement of wound edges, also participate in the closure process. However, the overall deformation pattern, as reported by tattooing squares on the skin of the dorsal area of a guinea pig, has clearly shown the presence of a reduction in skin wound area consistent with a principal deformation axis along the dorsal–lateral (DL) axis for this defect. The deformations amounted to strains between − 11 and − 67 % (contractions) along the DL axis, as well as lesser strains of 17 to 36 % (extensions, possibly including translations) along the anterior–posterior axis (Fig. 8.7, Troxel 1994). These observations were nearly identical to the deformation pattern observed earlier in a guinea pig skin wound using tattoo marks (Straile 1959) .

Fig. 8.7
Contraction of a full-thickness skin wound in the guinea pig dorsum. Tattoo marks were placed 1 cm apart both along the anterior–posterior (AP) and dorsal–lateral (DL) directions outside the 3·2 cm2 full-thickness excisional defect. Marks close to wound edges were placed 2–3 mm away from wound edges. Strains were determined as deformed length divided by the unstrained length and were − 11 to − 67% (contractions) along the DL axis while being 17 –36 % (extensions, possibly including translations) along the AP axis. Major deformation occurred by contraction along the DL axis. (From Troxel 1994)
8.3.2 Mechanics of Tissue Deformation During Spontaneous Closure of Wounds
Mathematical models of the contractile force and the deformation during wound closure in skin and peripheral nerve wounds are presented below. The models are not designed to provide accurate representations of data. Instead, they provide a rough overview of the cellular variables that control contraction in skin and nerve wounds and are qualitatively consistent with the evidence presented in this chapter.
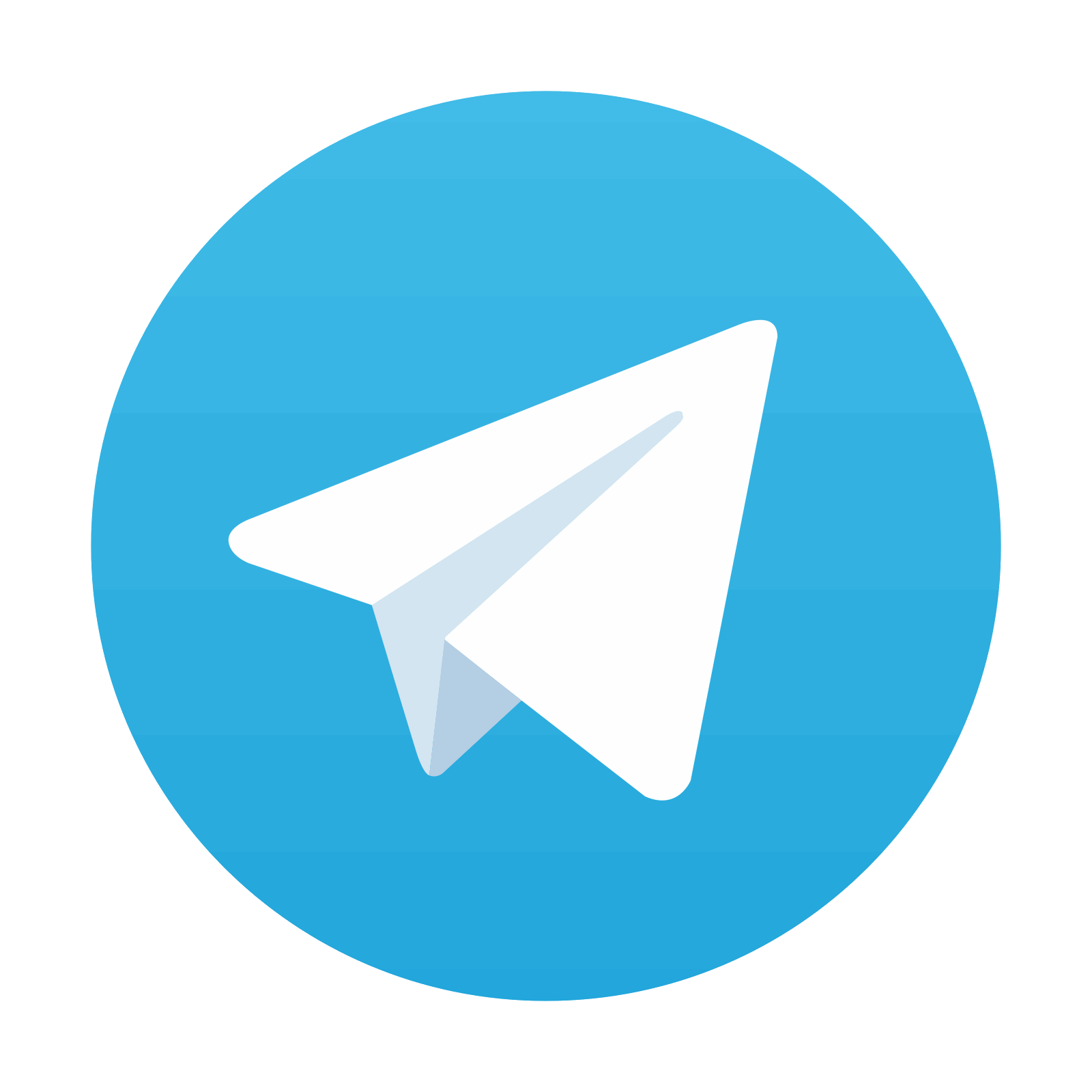
Stay updated, free articles. Join our Telegram channel

Full access? Get Clinical Tree
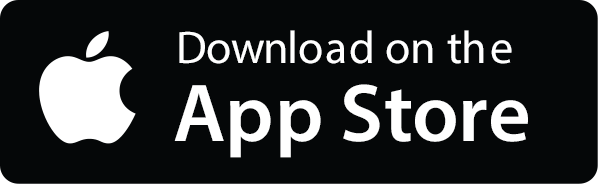
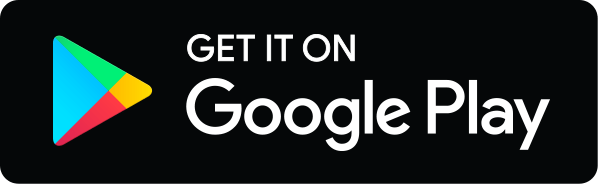