Fig. 9.1
Amniotic fluid cell-based engineered heart valve after 28 days in vitro conditioning in a diastolic pulse duplicator system (from [38])

Fig. 9.2
Human amniotic fluid cells (AFCs) sorted with CD133 magnetic beads resulted in endothelial-like CD133+ cells (a–e) and mesenchymal-like CD133− cells (f–j). While CD133+ cells showed positive staining for Vimentin, eNOS and vWF, CD133− cells stained positive for αSMA and Vimentin (from [61])
In the light of a careful preclinical evaluation of AFCs for cardiovascular tissue engineering applications, the characterization of amniotic fluid harvested from an ovine preclinical model—representing the standard model in cardiovascular medicine—has recently been reported [61]. Several biochemical parameters, like total protein amount or electrolytes, have been suggested as possible indicators for a specific isolation of amniotic fluid in sheep. Moreover, isolated cells showed a stable karyotype with expression of common mesenchymal stem cell surface markers as well as of some stem cell factors like NANOG and STAT-3. In contrast to the successful sorting of human amniotic fluid-derived cells based on AC133.1 [38], isolation of sheep endothelial cells was not possible using this technique. Nevertheless functional in vitro fabrication of tissue engineered vascular grafts and cardiovascular patches could be shown using amniotic fluid-derived cells (Fig. 9.3). However, more in vivo experiments in preclinical models are indispensable to evaluate this cell source for a possible human clinical translation. The exact in vivo fate of the cells needs to be defined in order to guarantee safety and exclude adverse effects for a potential prenatal therapy. In conclusion, amniotic fluid-derived cells represent an effective cell source for the treatment of congenital defects. In particular their early availability via low-risk procedures as well as their wide differentiation capacities hold great potential for autologous tissue engineering applications.


Fig. 9.3
(a–c) In vitro fabricated tissue engineered vascular grafts (small and large diameter) with the use of ovine amniotic fluid-derived cells (from [61])
References
1.
2.
3.
4.
Brennan MP, Dardik A, Hibino N, Roh JD, Nelson GN, Papademitris X, et al. Tissue engineered vascular grafts demonstrate evidence of growth and development when implanted in a juvenile animal model. Ann Surg. 2008;248(3):370–7.PubMedCentralPubMed
5.
6.
7.
8.
9.
Dasi LP, Simon HA, Sucosky P, Yoganathan AP. Fluid mechanics of artificial heart valves. Clin Exp Pharmacol Physiol. 2009;36(2): 225–37.PubMedCentralPubMedCrossRef
11.
12.
Emmert MY, Weber B, Behr L, Sammut S, Frauenfelder T, Wolint P, et al. Transcatheter aortic valve implantation using anatomically oriented, marrow stromal cell-based, stented, tissue-engineered heart valves: technical considerations and implications for translational cell-based heart valve concepts. Eur J Cardiothorac Surg. 2014;45(1):61–8.PubMedCrossRef
13.
15.
16.
17.
Hibino N, Yi T, Duncan DR, Rathore A, Dean E, Naito Y, et al. A critical role for macrophages in neovessel formation and the development of stenosis in tissue-engineered vascular grafts. FASEB J. 2011;25(12):4253–63.PubMedCentralPubMedCrossRef
18.
Hoerstrup SP, Cummings Mrcs I, Lachat M, Schoen FJ, Jenni R, Leschka S, et al. Functional growth in tissue-engineered living, vascular grafts: follow-up at 100 weeks in a large animal model. Circulation. 2006;114(1 Suppl):I159–66.PubMed
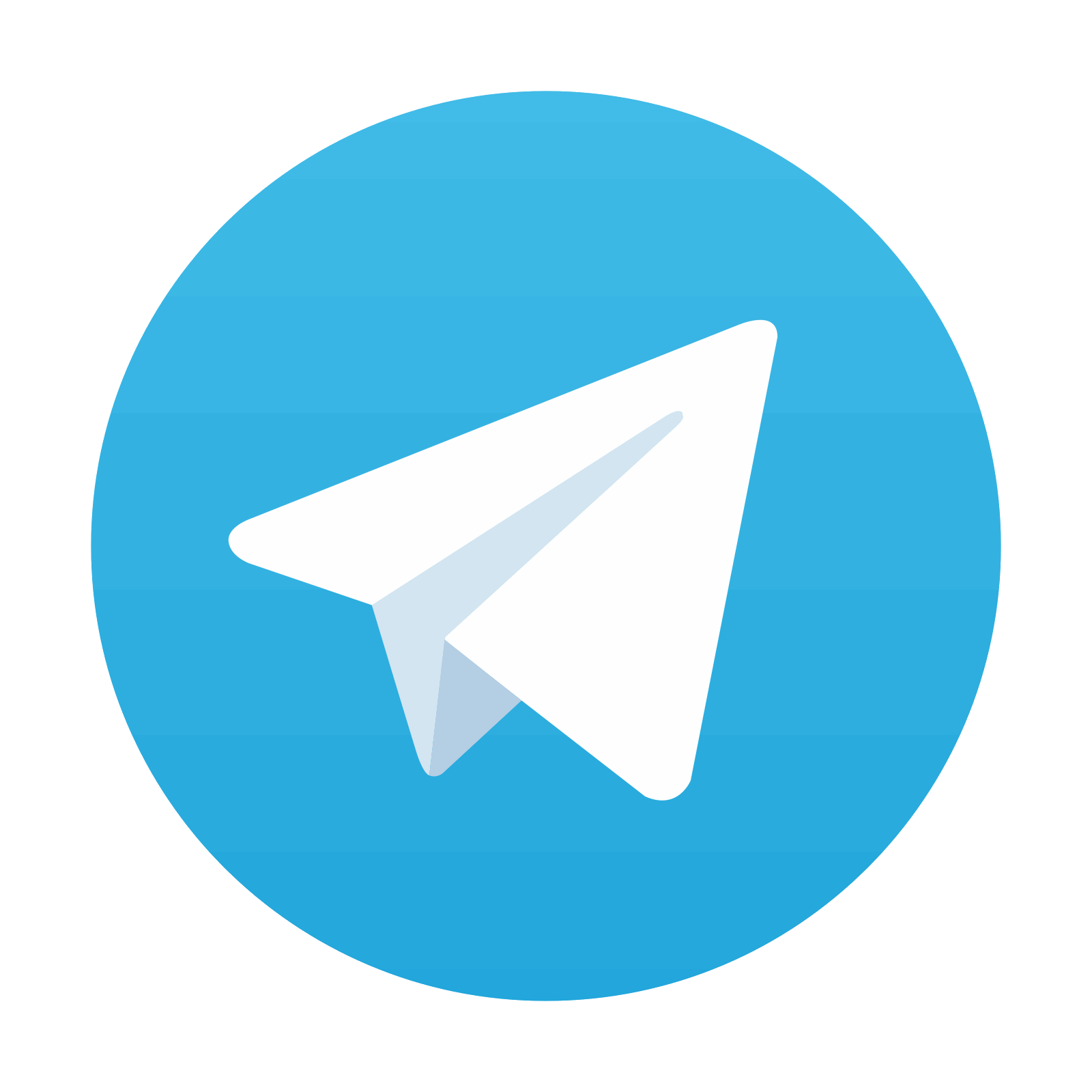
Stay updated, free articles. Join our Telegram channel

Full access? Get Clinical Tree
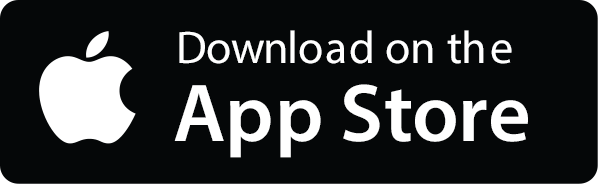
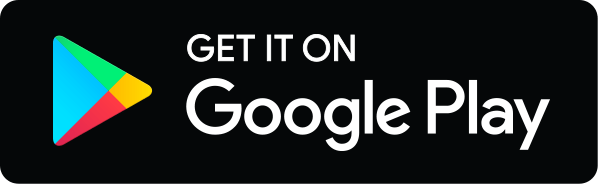