B. With just blood gas and common serum biochemistry data, we can manage the majority of clinical acid–base disorders.
C. The central focus of treating acid–base disturbances is the understanding of the biochemistry of the hydrogen ion.
1. Deviations in hydrogen ion concentrations from the normal range can cause marked alterations in protein structure and function, enzyme activity, and cellular function.
2. The largest contribution of metabolic acids arises from the oxidation of carbohydrates, principally glucose, to produce carbon dioxide (volatile acid, approximately 24,000 mEq/day).
3. The hydrogen ion concentration is regulated to maintain the arterial blood pH between 7.35 and 7.45.
a. The expression of the hydrogen ion concentration as pH masks large variations in hydrogen ion concentration despite small changes in pH.
b. For example, a pH range of 7.0 to 7.7 is associated with a fivefold change (100 nmol/L to 20 nmol/L) in hydrogen ion concentration. The pH of venous blood and interstitial fluid is lower than that of arterial blood (approximately 7.35).
II. Mechanisms for Regulation of Hydrogen Ion Concentration. Regulation of pH over a narrow range depends on (a) buffer systems, (b) ventilatory responses, and (c) renal responses. The buffer system mechanism is local and immediate but incomplete. Ventilatory responses are slower (minutes) and usually incomplete. Renal responses develop very slowly (hours) but can produce nearly complete pH correction.
A. Buffer Systems (Fig. 26-1). Body fluids contain acid–base buffer systems that immediately combine with acid or alkali to prevent excessive changes in the hydrogen ion concentration.
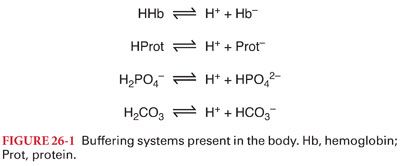
1. Bicarbonate buffering system consists of carbonic acid (H2CO3) and sodium bicarbonate (NaHCO3). Bicarbonate buffer is primarily a product of the approximately 200 mL of carbon dioxide produced per minute (Figs. 26-2 and 26-3). The bicarbonate buffer system accounts for >50% of the total buffering capacity of blood and approximately one-third of the bicarbonate buffering capacity of blood occurs within erythrocytes.
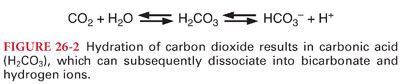
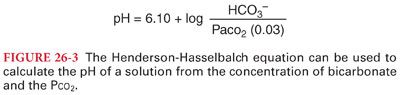
2. Hemoglobin Buffering System. Dissociation of oxyhemoglobin to deoxyhemoglobin facilitates the binding of hydrogen ions produced by the dissociation of carbonic acid. This situation is reversed in the pulmonary circulation where the conversion of deoxyhemoglobin to oxyhemoglobin facilitates the release of hydrogen ions.
B. Protein Buffering System. Like hemoglobin, other histidine-containing proteins are important intracellular buffers. Although the relatively low concentration of plasma proteins limits their role as extracellular buffers, hypoproteinemia will further reduce buffering capacity, especially in the critically ill patient.
C. Phosphate buffering system is important in most fluid compartments but is especially important in renal tubules, where phosphate is concentrated. Phosphate is a very important intracellular buffer because it is the most abundant intracellular anion.
III. Intracellular pH regulation. Although blood pH is commonly measured clinically, it is the intracellular pH (pHi) that is of functional importance (Table 26-2).
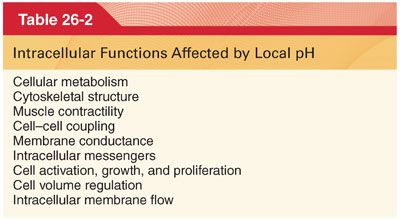
A. Ventilatory Responses
1. Ventilation is quantitatively the most important mechanism of acid removal, given the enormous daily production of volatile acid compared to nonvolatile acid.
2. Ventilatory responses cannot return pH to 7.4 when a metabolic abnormality is responsible for the acid–base disturbance (intensity of the stimulus responsible for increases or decreases in alveolar ventilation will begin to diminish as pH returns toward 7.4).
3. Most patients cannot hyperventilate to below 20 mm Hg.
4. It is likely that the insult causing severe metabolic acidosis will also adversely affect respiratory muscle function, thus compromising the respiratory response.
B. Renal Responses
1. The day-to-day renal contribution to acid–base regulation is directed toward the conservation of bicarbonate and the excretion of hydrogen ions.
2. Almost all filtered bicarbonate must be reabsorbed from the glomerular filtrate to maintain the normal plasma bicarbonate concentration (25 mEq/L) and plasma pH.
a. Carbonic anhydrase facilitates the dissociation of carbonic acid into water and carbon dioxide that both enter the renal tubular cell. Inhibition of carbonic anhydrase by acetazolamide interferes with the reabsorption of bicarbonate ions from renal tubular fluid. As a result, excess bicarbonate ions are lost in the urine and the plasma bicarbonate concentration is decreased (Fig. 26-4).
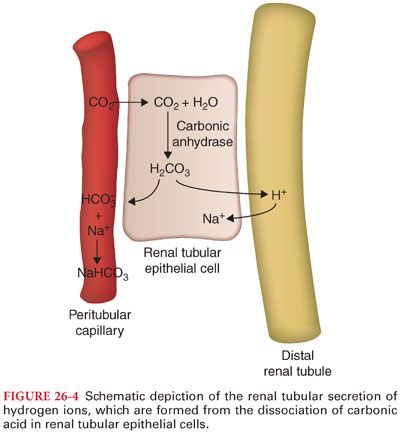
b. Hydrogen ions are secreted into renal tubules by epithelial cells lining proximal renal tubules, distal renal tubules, and collecting ducts (facilitated by aldosterone).
c. Active hydrogen ion transport is inhibited when the urinary pH drops below 4.0. Thus, hydrogen ions must combine with ammonia and phosphate buffers in the renal tubular lumen to prevent the pH from decreasing below this critical level (Fig. 26-5).
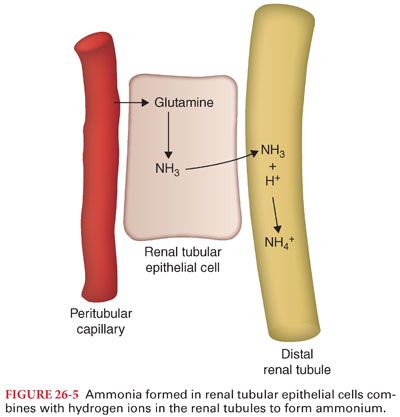
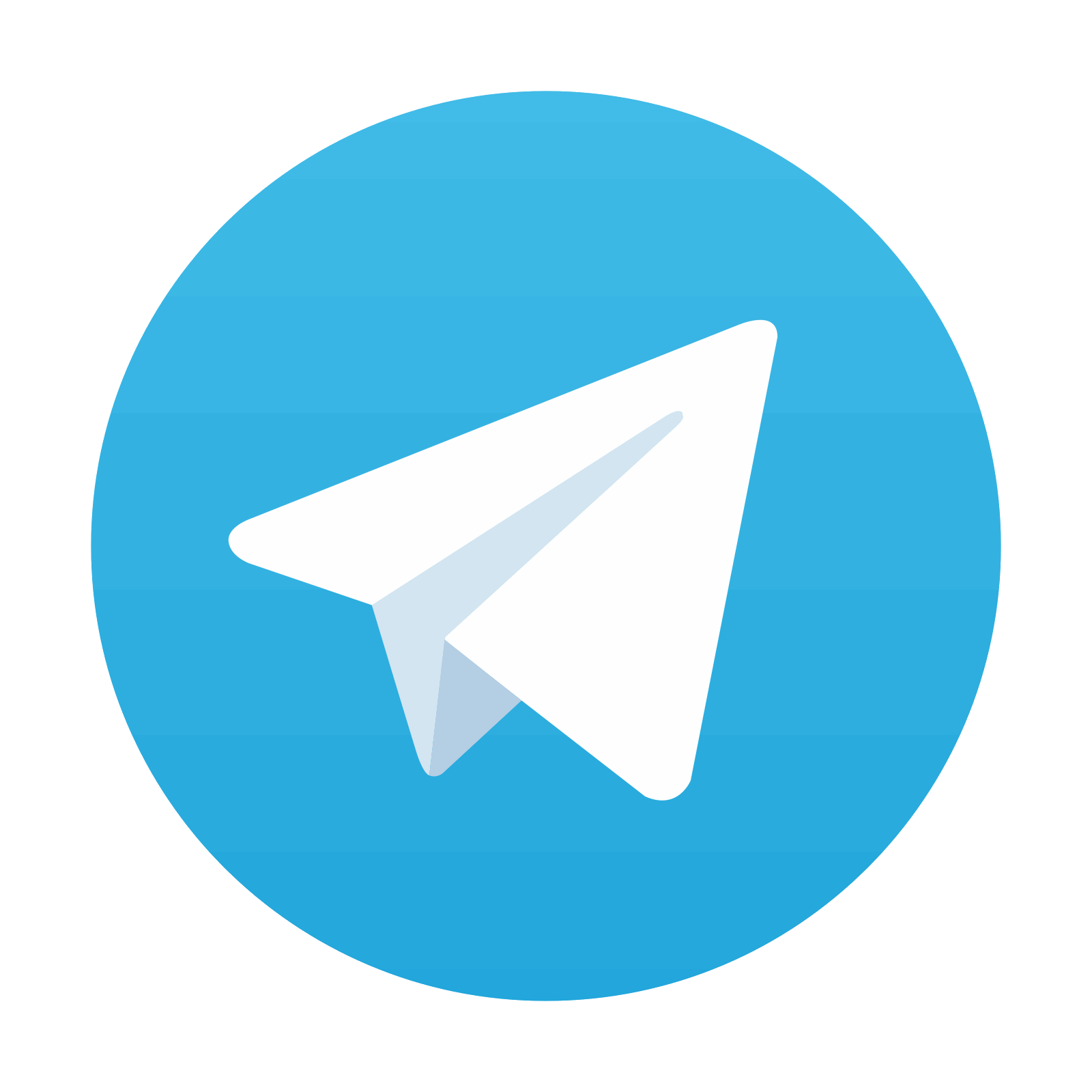
Stay updated, free articles. Join our Telegram channel

Full access? Get Clinical Tree
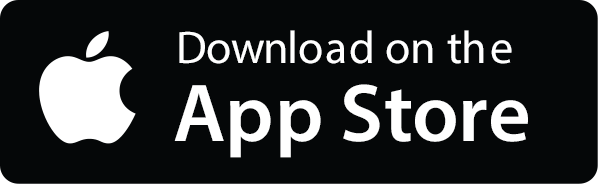
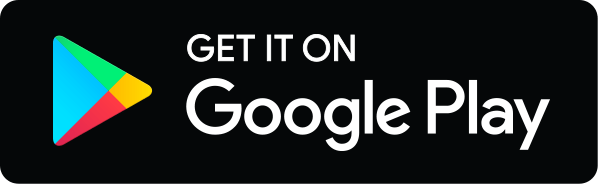