Figure 1.1:Stereoisomeric Forms of Stilbenes
Naturally occurring stilbenes (Table 1.1) exist overwhelmingly in the trans form. It has been observed that the trans and cis forms of stilbenes elicit different pharmacological activities. For example, trans-resveratrol was found to be ten times more potent in its ability to induce apoptosis in the HL 60 leukaemia cell line compared to cis-resveratrol (Roberti et al., 2003). Additional research has shown that trans-stilbene compounds to be significantly more potent in their ability to inhibit cyclooxygenase I (COX-I) activity compared to cis-stilbene compounds (Waffo-Teguo et al., 2001).
Table 1.1: Naturally Occurring Stilbenes
Name of the stilbeneand structure | Str.No. | Source | Activity | Reference |
1 | Polygonum cuspidatum; Vitis spp.; Vaccinum spp.; Morus spp.; Veratrum spp.; Arachis hypogaea; etc., | Anti-oxidant; Anti-cancer; Cardioprotectant; Anti-inflammatory; Anti-aging; Anti-diabetic. | Bagchi 2000 | |
2 | Dioscorea spp.; Bulbophyllum triste | Murine tyrosinase activity | Kittisak 2008 | |
3 | Melaleuca leucadendron; Cassia garretitana Vaccinium berries | Anti-leukaemic; tyrosine kinase inhibitor; anti-inflammatory anti-proliferative; anti-cancer and anti- Epstein-Barr virus drug | Potter et al.,., 2002; Wung et al.,., 2006; Swanson-Mungerson, et al.,., 2003. | |
4 | Cassia garretitana | — | Cunningham et al.,., 1963; Erdtman and Ronlán, 1969 | |
5 | Gnetum spp. | Potent tyrosinase inhibitor | Ohguchi et al.,., 2003. | |
6 | Morus spp.; Maclura pomifera; Artocarpus gomezianus | Neuroprotective,dopa oxidase activity of tyrosinase | Andrabi et al.,., 2004 Shin et al.,., 1998 | |
7 | Polygonum cuspidatum | — | Sovak, 2001 | |
8 | Eucalyptus wandoo | — | Castro et al.,., 1986 | |
9 | Gnetum cleistostachyum; Polygonum nodosum | Antifungal, Antibacterial | Lee et al.,., 2005. | |
10 | Dioscorea batatas | Antifungal; anti-bacterial | Harborne et al.,., 1999 | |
11 | Rheum spp. (incl. R. rhaponticum, R. undulatum); Scilla nervosa; | Antioxidant | Zhang et al.,., 2007 | |
12 | Gnetum spp.; Belamcanda chinensis; | Anti-oxidant | Wang et al.,., 2001 | |
13 | Gnetum cleistostachyum; Rheum undulatum Knema austrosiamensis; Rumex bucephalophorus | Potent inhibitor of CYP1A1 catalytic activity | Harborne et al.,., 1999 | |
14 | Rumex bucephalophorus; Pinus koraiensis | Potent inhibitor of CYP1A1 catalytic activity | Mikstacka et al.,., 2007 | |
15 | Knema austrosiamensis | Antifungal; anti-bacterial | Harborne et al.,., 1999 | |
16 | Pterolobium hexapetallum | Anti-Allergic | Matsuda et al.,., 2004 | |
17 | Rumex bucephalophorus | Anti-oxidant | Kerem et al.,., 2003 | |
18 | Dracena cochinchinensis; Pterocarpus spp. (incl. P. santalinus, P marsupium); Vitis vinifera; Pterolobium hexapetallum; | Anti-oxidant, anti-cancer, anti-hypercholesterolemia, anti-hypertriglyceridemia, anti-diabetic; antifungal, lowers cholesterol | Amorati et al.,., 2004 Rimando et al.,., 2002 | |
19 | Crotalaria madurensis | — | Bhakuni and Chaturvedi, 1984 | |
20 | Yucca periculosa, Y. schidigera; Cassia pudibunda | Anti-oxidant | Torres et al.,., 2003 | |
21 | Yucca periculosa | — | Torres et al.,., 2003 | |
22 | Cryptocarya idenburgensis | — | Juliawaty et al.,., 2000 | |
23 | Dracaena loureiri | — | Kittisak et al.,., 2002 | |
24 | Polygonum cuspidatum; Rheum rhaponticum Picea spp.; Lentils (Lens culinaris) | — | Romero-Pérez et al.,., 1999 | |
25 | Rheum spp.; Eucalyptus | Induction of apoptosis | Hibasami et al.,., 2007 | |
26 | Rheum rhaponticum | — | Aaviksaar et al.,., 2003 | |
27 | Pinus sibirica; Picea spp. | Hydrolytic activity of Trichoderma cellobiohydrolase | Shibutani et al.,., 2001 | |
28 | Rheum rhaponticum Polygonum cuspidatum; Spruce | — | Vastano et al.,., 2000. | |
29 | Polygonum cuspidatum; Pinus spp.; Vitis vinifera | Anti-oxidant | Gromova et al.,., 1975 | |
30 | Rhubarb (Rheum undulatum) | Inhibitory activity of NO production in lipopolysaccharide-activated macrophages | Matsuda, 2000 |
Resveratrol
In the group of stilbenes, the celebrity is resveratrol. Resveratrol (3, 4’, 5-trihydroxystilbene) is a natural polyphenol known to occur widely in plant sources (Figure 1.2). Resveratrol is found in at least 72 species of plants distributed among 31 genera and 12 families (Jang et al., 1997). Foods are also known to contain resveratrol and are limited to grapes, grape juice, cranberries, cranberry juice, peanuts and peanut products. In addition, it is also known to be present in various wines and maximum concentration was found in red wine (Table 1.2).
Figure 1.2:Source of Resveratrol
Table 1.2:Concentration of Resveratrol in Wines and Other Beverages (Baur and Sinclair, 2006)
Source | Conc. of trans-Resveratrol |
Red wines | 0.1–14.3 mg/l |
White wines | 0.1–2.1 mg/l |
Grapes | 0.16–3.54 μg/g |
Dry grape skins | 24.06 μg/g |
Red grape juices | 0.50 mg/l |
White grape juices | 0.05 mg/l |
Cranberry raw juice | ~0.2 mg/l |
Blueberries | ~0.32 ng/g |
Peanuts (raw) | 0.01–0.26 mg/146 g |
Roasted peanuts | 0.02–1.92 μg/g |
Boiled peanuts | 5.1 μg/g |
Peanut butters | 0.3–0.4 μg/g |
100% Natural peanut butters | 0.65 μg/g |
Polygonum cuspidatum | 0.524 mg/g |
Natural Source of Resveratrol
Resveratrol is a polyphenolic compound found in plants of many families. It can inhibit fungi pathogens of plants and regulate plants–parasite interaction (Sato et al., 1997). It was first reported in the peel of grape berries for disease resistance and later, in wines as one of the health promoting phenolic components. It was found to be the major polyphenol in the roots of Polygonum cuspidatum. The roots of this plant were traditionally used in Korea, China, and Japan as a folk medicine for the treatment of atherosclerosis and other therapeutic purposes. Resveratrol generated further interest after it was discovered to inhibit the copper-catalyzed oxidation of low density lipoprotein (Frankel et al., 1993), the platelet aggregation, arachidonic acid metabolism, reducing liver injury from peroxidized oil (Jang et al., 1997) and having cancer-chemopreventive activities (Jayatilake et al., 1993). Recent discovery that it acts as calorie restriction mimic and can extend life span of organisms, epitomized the interest in this molecule.
Technical Information on Polygonum cuspidatum
Japanese knotweed (Polygonum cuspidatum) is a perennial herb with spreading rhizomes and numerous reddish-brown, freely branched stems. The plant can reach four to eight feet in height and is often shrubby. The petioled leaves are four to six inches long and generally ovate with an abrupt point. The whitish flowers are borne in open, drooping panicles. The plant is dioecious, so male and female versions of the inconspicuous flowers are produced on separate plants. The approximately 1/8 inch long fruits are brown, shiny, triangular achenes (Hitchcock and Cronquist,1964; Hickman, 1993).
Figure 1.3:Leaves and Roots of Polygonum cuspidatum
Isolation of Resveratrol
Typical Process
The dried and crushed roots were extracted with methanol three times under reflux, and the extract was concentrated by reduced pressure to give a dark brown residue. The residue was chromatographed over silica gel column using mixtures of chloroform/methanol (3:1 to 1:1, v/v) for elution or chromatography over polyamide column using methanol for elution to give resveratrol and pieced as individual pure compounds.
Synthetic Approaches to Resveratrol
Many synthetic routes were developed to obtain resveratrol and a summary is given in Figure 1.4.
Figure 1.4:Synthetic Approaches to Resveratrol
Biological Activities of Resveratrol
Resveratrol has acquired lot of importance, because of its diverse biological activity and therapeutic profile. There is ongoing debate about the exact mechanism of action by which the desired effects of resveratrol take place. Important biological activities are listed below.
- A strong free-radical scavenger and antioxidant
- An antiinflammatory agent
- Anticancer agent
- Cardioprotectant
- Antidiabetic
- Antiobese
- Phytoestrogen
- A neuroprotectant
- Calorie Restriction Mimic
Free-radical Scavenger and Antioxidant Activity
Resveratrol is a strong free radical scavenger and potent antioxidant, because of its ability to promote the activities of a variety of antioxidative enzymes. The antioxidant activity of polyphenolic compounds depend on the redox properties due to their phenolic hydroxyl groups and the potential electron delocalization across the chemical structure. Oxidation is the transfer of electrons from one atom to another and represents an essential part of aerobic life and in our metabolism, since oxygen is the ultimate electron acceptor in the electron flow system that produces energy in the form of ATP (Adenosine triphospate). The oxygen molecule is very stable in the ground state, but it is changed into reactive oxygen species (ROS), namely, superoxide ion (O2–1), peroxide radical (?OOH), hydroxyl radical (?OH) and nitric oxide (NO?) by the environmental pollutants, radiolysis, UV and the reduction pathway to H2O in the living tissues. These free radicals play a major role in the initiation and progression of a wide range of pathological diseases like cancer, Alzheimer’s, Parkinson’s and cardiovascular diseases. In view of this, considerable attention has been given to the addition of antioxidants in foods and supplementation of antioxidants to biological systems to scavenge free radicals.
The aging process exemplifies the cumulative result of deterioration of individual cells, tissues and organs, promoted by free radicals. The human body has built-in mechanisms to counteract free radicals. These mechanisms are collectively known as the body’s antioxidant defense mechanism. Unfortunately, in most instances, the antioxidant defense is gradually overwhelmed by the aging process, or a disease, or both. The inflammatory processes associated with microbial or viral infections and the progression of cancer are just a few disease conditions which contribute to depletion of the antioxidant defense system of the body. Therefore, it is important to preserve the body’s defenses against damages by free radicals. Some vitamins, minerals, and natural compounds such as phenolics, flavonoids and carotenoids, have the ability to counteract free radical damage by scavenging or neutralizing the free radicals. These diversified groups of biologically important substances are known as “antioxidants’.
Phenolic compounds exert their antioxidant activity by acting primarily as hydrogen atom donators, thereby inhibiting the propagation of radical chain reactions. Antioxidant potential of the phenolics depends on the number and arrangement of phenolic hydroxyl groups, as well as the nature of the other substituents on the aromatic rings.
Losa, 2003 studied the radical scavenging property of resveratrol using peripheral mononuclear cells from healthy humans which are incubated with the oxidant 2-deoxy-d-ribose and found that resveratrol scavenged O2 generated by 2-deoxy-d-ribose. The research group at the university of California, Davis (Frankel et al., 1993) have observed that an ex vivo system whereby conjugated dienes were generated from native LDL during Cu2+ mediated oxygen, and showed that the phenolic constitutes of red wine prevented these oxidative changes and were several fold more potent than α–tocopherol in this respect and the same team extended their studies using pure quercetine, epicatechin and trans-resveratrol. The later was not as potent as quercetine, epicatechin in blocking Cu2+induced oxidation of human LDL, but it was superior to α-tocopherol on a molar basis.
Amorati et al., 2004, investigated recently a series of compounds, cis-resveratrol and trans–resveratrol and their derivatives to understand the effect of the geometry of the olefinic double bond on their antioxidant activity. These authors have evaluated antioxidant activity quantitatively by measuring two parameters, i.e., the rate constant for the reaction with peroxyl radicals and the bond dissociation enthalpy (BDE) of the phenolic O-H bond. The results revealed higher activity for the trans resveratrol compared to cis-isomer, but, such a significant difference was not observed in other pairs of trans and cis isomers (Figure 1.5).
Figure 1.5
Zhou and Liu 2005, have studied antioxidant activity by kinetic and mechanistic studies in micelles, in red blood cells, in low-density lipoprotein (LDL) and in microsomes on resveratrol and related trans-stilbene analogs such as, trans-3, 5-dihydroxystilbene (9), trans-4,4’-dihydroxystilbene (21), trans-4-hydroxystilbene (31),, trans-3,4-dihydroxystilbene (34), trans-3,4,5-trihydroxystilbene (35), and trans-3,4,4’-trihydroxystilbene (36) and found that all of these resveratrol analogs exhibit significant antioxidant activity. It is worth noting that the antioxidative activities of stilbenes bearing ortho-dihydroxyl functionality (34, 35 and 36) are appreciably higher than those of resveratrol. The antioxidant activity was found to correlate with the electrochemical behavior. Molecules with lower oxidation potentials and reversible cyclic voltammograms, that is, compounds, 21, 34, 35 and 36, exhibit higher activity, while molecules with higher oxidation potentials and irreversible cyclic voltammograms, that is, 9 and 31, and resveratrol are less active. The data suggest that electron-transfer antioxidation might take place simultaneously with a direct hydrogen-abstraction reaction.
A team at New York Medical College (Ungvari et al., 2007) found in their study in blood vessels, that resveratrol i) attenuates oxidative stress-induced cell death and ii) exerts antioxidant effects, in part, by up regulating vascular antioxidant systems. However, the mechanisms by which resveratrol exerts its vasculoprotective effects are not completely understood. Because oxidative stress and endothelial cell injury play a critical role in vascular aging and atherogenesis, they evaluated whether resveratrol inhibits oxidative stress-induced endothelial apoptosis and found that oxidized LDL and TNF-α elicited significant increases in caspase-3/7 activity in endothelial cells and cultured rat aortas, which were prevented by resveratrol pretreatment. The protective effect of resveratrol was attenuated by inhibition of glutathione peroxidase and heme oxygenase-1, suggesting a role for antioxidant systems in the antiapoptotic action of resveratrol. Indeed, resveratrol treatment protected cultured aortic segments and/or endothelial cells against increases in intracellular H2O2 levels and H2O2-mediated apoptotic cell death induced by oxidative stressors (exogenous H2O2, paraquat, and UV light). Resveratrol treatment upregulated the expression of glutathione peroxidase, catalase, and heme oxygenase-1 in cultured arteries, whereas it had no significant effect on the expression of superoxide dismutase (SOD) isoforms. Resveratrol also effectively scavenged H2O2 in vitro. Thus, resveratrol seems to increase vascular oxidative stress resistance by scavenging H2O2 and preventing oxidative stress-induced endothelial cell death. The prevention of oxidative stress-induced apoptotic cell death, the dual H2O2-lowering effects of resveratrol (both direct scavenging of H2O2 and up regulation of antioxidant enzymes) are likely to contribute to its inhibitory effect on H2O2-induced endothelial activation and NF-κB induction.
A team from Rutgers University (Wang et al., 1999) have synthesized resveratrol (1) and five other polyhydroxystilbenes, namely, trans-3,3’,4,5’-tetrahydroxystilbene (3); trans-3,4,4’,5-tetrahydroxystilbene (7), trans
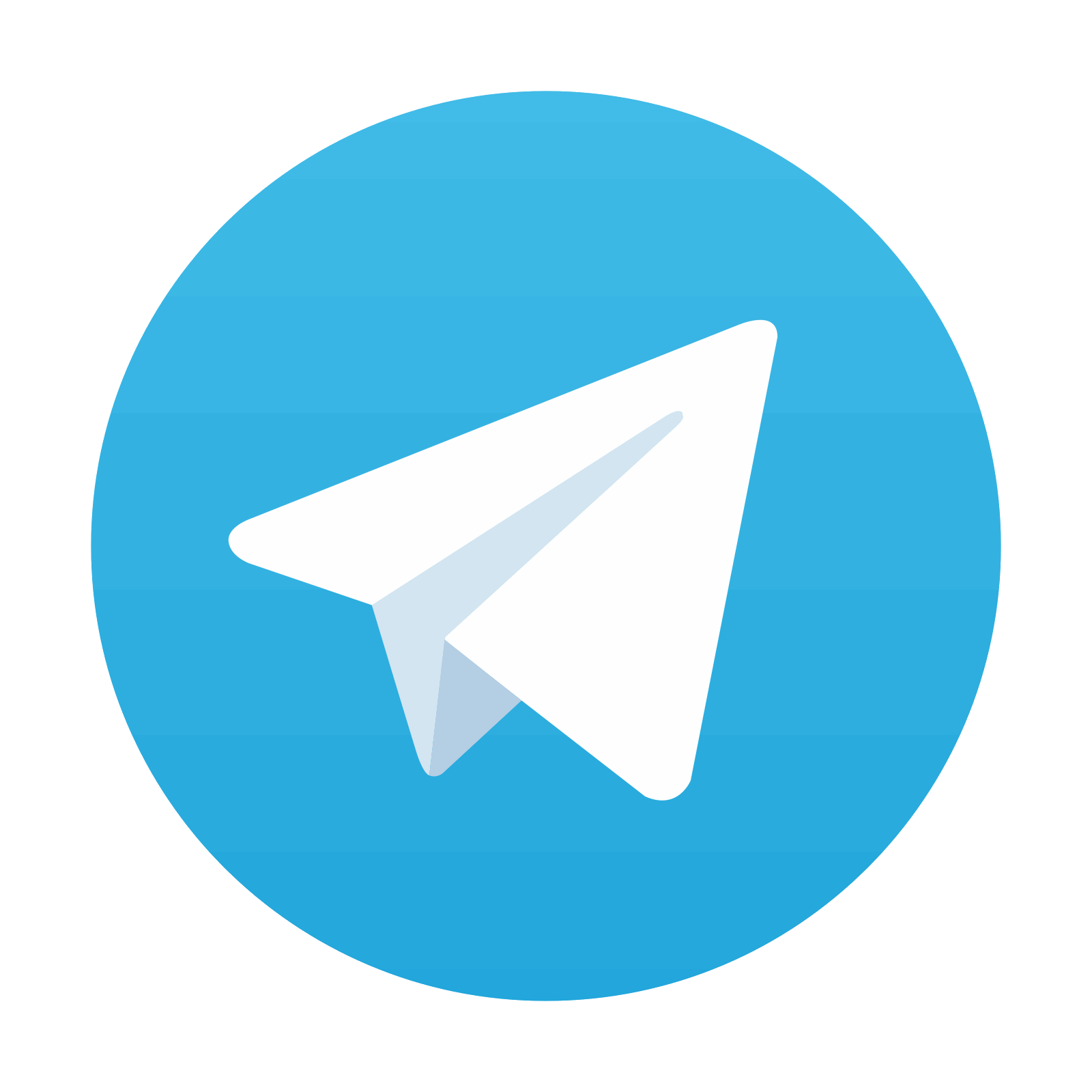
Stay updated, free articles. Join our Telegram channel

Full access? Get Clinical Tree
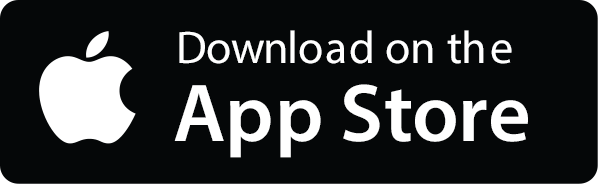
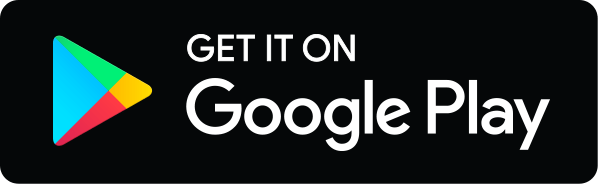