A Flexible Model for Compound Management Facilities to Stimulate Collaborations in the Life Sciences
Griffith University, Nathan, Queensland, Australia
Global Context
An enormous cache of human biology remains to be explored following completion of the Human Genome Project (HGP). The expectation is that selective modulators for new targets will be discovered that, in some cases, will be translated into novel therapeutics. Indeed, the identification of new structural classes is one of the many drivers en route to understanding biological systems and developing innovative, safer therapeutics with novel modes of action. The colossal number of molecules, estimated to be in excess of an estimated 1062 compounds [1] composed of the atoms that make up current molecular therapies, effectively ensures a vast reserve of structural diversity can be mined to achieve this goal. However, despite this amazing diversity, it would nevertheless be impossible to synthesize even one molecule of each member from this set considering there is estimated to be “only” 1050 atoms on Earth [2]. The real challenge lies in the selection of small molecules that can actually probe or otherwise modulate human biology in a therapeutically useful manner. In this respect, biologically valid small molecules (organic compounds with a molecular weight <500 composed mainly of carbon, hydrogen, and oxygen atoms, but typically also nitrogen, sulfur, and fluorine, and less often chlorine, bromine, and phosphorus) that interact with biology space (e.g., the surface of a protein) have become critical tools for understanding important cellular events and biological pathways involved in health and disease.
Until the completion of the HGP, however, only a few hundred human proteins had been studied in detail via small molecules [3]. Because many of these compounds were found/discovered serendipitously in animal models or cell lines, more modern approaches had to be developed to keep abreast of the massive amount of data emanating from the HGP and millions of compounds that could be produced by combinatorial chemistry. The resulting paradigm shift was driven by advances in molecular biology and robust automation so that it is now possible to undertake high-throughput screening (HTS) of 100,000 compounds per day against an isolated biomolecular target (usually a recombinant protein) or cell line to facilitate the efficient discovery of many more useful compounds. The screening of large and structurally diverse small molecule libraries that impede the function of specific target proteins is now commonplace in industrial drug discovery programs [4]. The same tools (compounds) and techniques (automated screening) are also being used by academia in the emerging interdisciplinary field of chemical biology to identify new proteins and map biochemical pathways in the context of functional assays [5–7].
Current wisdom suggests that the quest to identify new molecules that can ultimately be developed into drugs will be facilitated via a combination of chemical biology and phenotypic screening, typically pursued in a basic research environment, and a concerted drug discovery program which, for the most part, is only possible with the financial backing of industry. Chemical biology employs small molecules to interrogate biological systems and pathways. In contrast to classical genetics where manipulation occurs at the DNA level, small molecules generally modulate protein function, and not the gene locus or mRNA, by inducing conformational changes or competing for naturally occurring protein–ligand or protein–protein interaction sites resulting in altered activity. This allows temporal study of signaling pathways and the ability to wash out probes to study reversible inhibition.
Phenotypic screening enables the discovery of compounds which modulate biological systems in a target- and mechanism-agnostic manner, and is complementary to biochemical HTS where compound libraries are evaluated against an isolated protein target. Phenotypic drug discovery directly interrogates complex biological systems composed of multiple or unknown biochemical components and pathways via imaging of cells using high-content screening (HCS) techniques. The phenotypic approach is reminiscent of drug discovery strategies that predate the advent of molecular biology and HTS. HCS, like HTS, has benefited from advances in imaging technologies, assay miniaturization, robust automation, bioinformatics, and the ready availability of large compound libraries. Additionally, phenotypic screening profits from facile access to physiologically relevant biological systems (model organisms together with primary, differentiated, and stem cells).
Significant time and effort can be dedicated in academia to identifying small molecule modulators for proteins, receptors, DNA, and RNA that further explore the underlying biology of a specific system—a luxury not readily amenable to commercial reality. However, while the activities of academia are broader than those of industry, both sectors are inextricably linked as the industrial pipeline of candidate drugs comes to rely increasingly more upon knowledge generated by academic researchers. This trend is expected to continue given the many and complex issues currently facing the pharmaceutical industry. Hence, academic research that aims to comprehend the underlying biology of a disease state, validate drug targets, or otherwise add value to basic research is becoming progressively more important.
Early-phase drug discovery is also pursued by many academic screening centers in addition to curiosity-driven research as the underlying infrastructure, skill sets, and procedures required to unearth a new modulator for chemical biology or phenotype screening programs is identical to the identification of a lead molecule for potential development into a pharmaceutical. The possible development a marketable product in the form of a therapeutic following screening of a target that was identified through a university’s own basic research makes the acquisition of infrastructure for screening molecular libraries extremely attractive. In this scenario, a hit is usually taken as far down the value-added chain as possible before partnering with industry.
The inclusion of drug discovery screening programs in academia has no doubt been aided by the HGP, which has provided many potential new drug targets outside the “low-hanging fruit” for which the vast majority of current therapeutics have been developed, and the recent ruling in the case of the University of Rochester versus Pfizer [8]. In the latter, discovery of COX-2 protein by the university was insufficient to file a patent claim on the basis that the target could be inhibited using a compound yet never actually disclosing any compound. The real invention was deemed to be the compound (Celebrix) developed by Searle (later Pfizer), which brought about a reduction in acute pain and inflammation via specific inhibition of COX-2. This ruling effectively means that potentially druggable targets found through basic research emanating from the “-omics” sciences are not patentable per se but require a compound to demonstrate usefulness. HTS and HCS therefore afford a mechanism to validate the discovery of a new target through disclosure of a compound that can inhibit, or otherwise modulate, its action. The benefits to academic and other nonindustry organizations are potentially enormous. Payment of milestones or substantial licensing revenues is possible depending, inter alia, on the size of the intended market for the drug, the novelty of the compound, how far along the value-adding pipeline the compound has progressed, and the manner in which the intellectual property (IP) was negotiated with an industry or product development partner.
It is interesting to note that while many academic organizations have developed a genuine capability for HTS, far less have invested in compound management. This is no doubt due, in part, to the fact that the combined cost to acquire a sizable library of more than 100,000 compounds and the supporting infrastructure can be prohibitive. Put simply, the value proposition for establishing a dedicated compound management capability to complement HTS where the library is less than 100,000 compounds does not necessarily justify the outlay, especially for the academic sector. To obviate this limitation, several governments have used public funds to establish centralized repositories to augment already established screening centers or newly created networks. This allows the screening sites to access small molecule libraries at a far lower cost than if they were purchased from commercial vendors and also obviates the need to initially purchase and then maintain additional infrastructure.
Two of the more high-profile publicly funded schemes include the U.S. National Institutes of Health (NIH) National Center for Advancing Translational Sciences (NCATS) [9], which had its genesis in the NIH Roadmap Molecular Libraries Program (MLP) [10], and the pan-European effort, EU-OPENSCREEN [11]. Both the U.S. and European initiatives aim to extend knowledge of human biology via investing in the identification and validation of new targets implicated in various disease states, and perturbing these targets with small molecule libraries. Ultimately, the objective of this chemical biology-based approach is to catalyze the wealth of information arising out of the HGP into tangible health benefits.
The NIH Roadmap was conceived in 2002 and, following funding to several programs in 2004, became the world’s first truly national chemical biology initiative [12]. The NIH invested heavily in equipment and infrastructure to support basic research and development in order to achieve its goals. This initially included, inter alia, establishment of a Small Molecule Repository (SMR) that collated and supplied compounds to a network of 10 screening centers known collectively as the Molecular Libraries Screening Centers Network (MLSCN) [13]. The MLSCN was launched in mid-2005 as a pilot phase to the MLP. Targets for screening were funded by grants to predominately academic researchers to identify and develop assays for novel targets. The synthesis of chemical libraries that expanded the chemical space occupied by commercial libraries was also supported in the pilot phase. The immediate goals of the MLSCN were to expand the availability and use of chemical probes contained in the SMR to explore the function of genes, cells, and pathways in health and disease. Importantly, annotated information on the biological profiles of compounds was made available through PubChem, the NIH’s publicly accessible database. The Molecular Libraries Probe Production Centers Network (MLPCN) superseded the MLSCN pilot phase in mid-2008. In addition to providing capacity to screen over 300,000 small molecules that had been collated over the previous 3 years using a variety of assay formats, the MLPCN significantly expanded its core capabilities to include medicinal chemistry and informatics related to the development of promising probes and library production. The MLPCN’s combined resource is presently distributed among nine sites, four of which hold “comprehensive” status in that they prosecute both HTS and probe-related chemistry, three that undertake screening only and two that are specialist chemistry sites [10].
The MLP has positioned itself in the precompetitive arena and does not have the remit to embark on a fully-fledged drug discovery program per se. Rather, it aims to understand complex human biology through the use of small molecule modulators and place all data in the public domain. However, the NIH may still carry out early phase drug discovery should a promising probe be identified with limited commercial potential. In this respect, the success of the MLP was underscored with an announcement in early 2011 that the program had attained its first landmark achievement: FDA approval to conduct a Phase 1 clinical safety study for a sphingosine-1-phosphate receptor 1 (S1P1) agonist. The original probe report was submitted to PubChem in 2007 by the Scripps Research Institute and has since undergone further development outside of the MLP by Scripps and Receptos Inc. The clinical trial has been initiated as a potential treatment for multiple sclerosis.
EU-OPENSCREEN is a pan-European network that currently involves partners form 12 countries (Austria, Czech Republic, Denmark, Finland, France, Germany, Italy, the Netherlands, Norway, Poland, Spain, and Sweden). The EU-OPENSCREEN initiative effectively leverages existing national networks like Germany’s ChemBioNet [14] and other specialist technology platforms such as the FIMM High Throughput Center in Finland [15] into a seamless well-equipped entity that permits researchers in academia and SMEs to access resources for the development of bioactive small molecules. Planning is already well under way with the goal that EU-OPENSCREEN will be fully operational from 2015.
Like the MLP, the activities of EU-OPENSCREEN precede commercial development. EU-OPENSCREEN will be open to all European organizations involved in chemical biology and is committed to open access. It is envisaged that a legal entity will be created in an effort to facilitate collaboration among members and interactions with stakeholders and external users. In a departure from the MLP IP model, EU-OPENSCREEN is currently developing a flexible IP framework that attempts to balance rapid knowledge sharing and exploitation activities. Thus, researchers are able to protect knowledge gained following use of EU-OPENSCREENs resources before publishing in the database.
There are many other national initiatives in addition to the NCATS. Some like the Chimiothèque Nationalé (France) [16] are part of EU-OPENSCREEN and have been established to allow academic institutions to prosecute HTS and phenotypic screening of small molecule libraries. They are typically less expansive than the NIH effort and may occur as self-organized consortia that provide a range of chemical, screening, and informatics resources such as the Centre for Drug Research and Development (Canada) [17], CGC (Germany) [18], and the UK Drug Discovery Consortium [19]. Alternatively, they may take the form of an institute that makes resources and infrastructure available to the broader scientific community like the RIKEN Program for Drug Discovery and Medical Technology Platform in Japan [20], the Institut Pasteur Korea [21], Tel Aviv University (Israel) [22], the Dundee Drug Discovery Unit (Scotland) [23], Drug Discovery Centre at Imperial College (England) [24], the Broad Institute of MIT and Harvard (USA) [25], the Kansas University HTS Laboratory (USA) [26], or the Memorial Sloan-Kettering Cancer Center HTS Core Facility (USA) [27] to name a few from a large and expanding list. Additional academic screening centers can be found on the website for the Society for Laboratory Automation and Screening [28].
Finally, although not a publicly funded initiative, and somewhat outside the scope of this chapter, the Eli Lilly Phenotypic Drug Discovery (PD2) Initiative [29] is worth mentioning as it provides another mechanism whereby academic compound collections may be screened. Lilly introduced the PD2 initiative because of the slew of issues facing the pharmaceutical industry today. Lilly believes that the solution to future innovation lies, in part, through collaborative research with universities, institutes, and biotechs using a “neoclassical” approach to drug discovery. It is Lilly’s belief that the phenotype drug discovery paradigm can accelerate the search for high-quality drug candidates and, to this end, they have established a panel of assay “modules” which represent therapeutic areas of long-term strategic interest to the company in oncology, neurological disorders, and metabolic diseases. Each module is composed of a phenotypic lead generation assay which is followed by relevant biochemical and cellular assays designed to quickly delineate a compound’s activity profile and potential for future development.
External investigators can upload and submit structures of their compounds via a secure site where they are evaluated for novelty, lead- and drug-likeness. If a molecule passes this decision gate, investigators are invited to submit a physical sample to Lilly for testing in the PD2 panel. In exchange, the investigator receives a full report of the biological data. Once testing is completed, Lilly evaluates the data and determines whether to initiate discussions leading to a possible collaboration. Importantly, the investigator (or their organization) retains all IP rights in the compound and is free to publish the data if mutual consent is not reached or the molecule does not show any promise.
Australian Context
Australia has a small population by world standards (23M), which can limit the availability of public funds to support the establishment of systemic infrastructure, functional capability (e.g., staff costs and equipment maintenance), and project-related costs (reagents, labware, etc.) across multiple sites like the erstwhile MLSCN or MLPCN.
Highly competitive funding schemes from the federal government have helped cultivate a quality basic medical research environment [30]. This has contributed, in part, to an outstanding scientific output over the past 50 years and allows Australia to continually punch above its weight in science and technology, contributing 3% of global medical research publications each year and establishing 10 times the number of World Health Organization (WHO) “collaborating centers” than would be expected for a population that accounted for 0.3% of the world’s total [31]. Moreover, Australia currently ranks seventh in the world for the number of science research publications per capita [32]. The largest number of research publications originates from the medical and health sciences (which account for approximately one-third of papers published) and the biological sciences (approximately one-fifth of publications).
This international standing in medical research is evident among Australia’s six Nobel laureates for physiology or medicine. Five were born, educated, and performed the work for which they were awarded the Nobel Prize in Australia: Sir Frank Macfarlane Burnett (1960), John Eccles (1963), Peter Doherty (1996), Barry Marshall, and Robin Warren (2005). The sixth, Howard Florey (1945), graduated from the University of Adelaide with an MB, BS in 1921 before being awarded a Rhodes Scholarship to continue his studies in England.
Other notable Australian efforts include the WHO smallpox eradication program led by Frank Fenner from the Australian National University that freed the world from this devastating disease. The program removed smallpox that threatened 60% of the world’s population, killed every fourth victim, and scarred or blinded most survivors. Over 3.5 million patients have been treated with the colony-stimulating factors discovered by Don Metcalf and his colleagues at the Walter and Eliza Hall Institute of Medical Research. These naturally occurring proteins accelerate white blood cell regeneration in patients treated for cancer. More recently, the development of an innovative neuraminadase inhibitor for influenza infections by Peter Colman, Graham Laver, and Mark von Itzstein has become part of the defensive armory against the threat of avian influenza, while a team led by Ian Frazer at the University of Queensland developed a vaccine for cervical cancer that could protect millions of women. Yet, despite these and other success stories, preclinical development is not as strong as its basic research counterpart, and the immediate challenge is to translate this high world standing into internationally competitive industrial niches.
Australia’s 3% contribution to global medical science has not translated into a local industry that accounts for 3% of global drug development [33], in part because of the relatively small number of drug candidates entering preclinical trials every year [34]. Indeed, only four FDA-approved small molecule pharmaceuticals have been largely developed in Australia, that is, Eryc, Doryx, Kapanol, and Relenza, with the first three being formulations rather than de novo developed drugs. In effect, investment in basic research does not realize its full potential, and there is a clear opportunity cost if Australia becomes a country that supplies disease knowledge but fails to supply therapeutics.
As evidenced by Relenza [35], Australia has the necessary skill set to prosecute small molecule drug development but, with the advent of the HTS and HCS, began to suffer from a lack of systemic infrastructure which prevented modernization and the integration of new skills into a truly competitive capability. The region’s chemical and life science communities stand to profit from entry into the HTS and phenotypic screening paradigms. HTS and HCS of appropriately constructed compound libraries can facilitate the discovery of small molecule modulators against validated targets. In turn, these lead or probe molecules may act as the starting points for value adding through existing centers of expertise in structural biology, modeling, synthesis, ADME, toxicology, scale-up, animal models, and clinical trials. Demonstration that a compound has promising pharmacological activity, favorable ADME characteristics, and acceptable toxicity facilitates its progression along the pharmaceutical value chain. The existing capabilities dovetail with HTS and phenotypic screening methodologies and allow a more mature IP position to be obtained before partnering.
Over the past 10–15 years, a few farsighted Australian research organizations and State governments have invested to create the infrastructure necessary for pharmaceutical discovery; however, the public institutions possessing this infrastructure are under enormous financial pressure and, without the support of funding agencies, are unable to provide the recurrent funding on a scale necessary to build a sustainable Australian preclinical capacity. Consequently, translation of basic research fails to occur or drug discovery IP is sold to overseas interests too early and for too little value.
A paucity of systemic infrastructure to underpin the early discovery phase in the HTS and phenotypic screening paradigms potentially means that Australia would not be in as strong a position to exploit the “-omics” revolution as it relates to small molecule drug discovery and the understanding of biological interactions through new interdisciplinary sciences like chemical biology. The region would benefit enormously if the screening of small molecules was facilitated to unearth probes that allow robust interrogation of biological systems and concomitantly generate leads for drug discovery that act as the starting point for value adding via existing centers of expertise so that a more mature IP position could be obtained before partnering.
Preexisting Conditions in Australia Facilitating Establishment of the QCL
HTS was introduced into Australia during the early 1990s via a collaborative drug discovery program between the pharmaceutical company, AstraZeneca, and Griffith University. Griffith University screened natural product extracts against predominately biochemical assays supplied by AstraZeneca in an effort to provide innovative leads for further development. Although well funded, the exclusive nature of this arrangement did not permit screening of compounds from academia or SMEs against targets that may have impinged on AstraZeneca’s business interests.
By 2001, approximately 45,000 biota samples had been collected from megadiverse countries in accordance with the United Nations Convention on Biological Diversity (UN CBD). Two extracts were taken from 35,000 samples. The ensuing 70,000 extracts along with 2000 natural products and 1000 synthetics were subsequently stored as stock solutions in microtubes equipped with a 2-D barcode. While the introduction of this labware facilitated cradle-to-grave tracking, increased flexibility, and efficiency, it nevertheless suffered from the fact that microtube racks (or occasionally specific tubes) had to be retrieved manually.
It was not until 2003, some 10 years after commencement of HTS at Griffith University, that an open access platform became available through a consortium based in the State of Victoria. This platform was located at the Walter & Eliza Hall Institute (WEHI) of Medical Research in Melbourne and, in addition to automation, incorporated a library of 100,000 compounds in 384-well plate format obtained from commercial vendors. Automated screening began in South Australia a few years later when Bionomics integrated small molecule drug discovery into previously established technology platforms in the genomics of epilepsy, angiogenesis, and breast cancer.
By 2007, the exclusive agreement between AstraZeneca and Griffith University had ceased allowing the contribution of HTS resources, together with the WEHI and Bionomics, into a federal government-sponsored program: the Cooperative Research Centre for Cancer Therapeutics (CRC CTx). The CRC CTx fills a frustrating gap for Australian cancer researchers through provision of funds that permits a promising small molecule lead to progress to the regulatory preclinical stage. Small molecule leads for new targets are typically identified following HTS of an expansive compound library. The strong undercurrent of basic research also prompted other groups with exemplary track records in child-related cancer at the Children’s Cancer Institute of Australia in New South Wales and G-protein-coupled receptors (GPCRs) at Monash University to seek funding via different mechanisms to establish HTS and phenotypic screening capabilities.
The screening of large diverse chemical libraries to identify small molecules capable of modifying the behavior of a target protein or a cellular phenotype effectively means that biology and chemistry are really two sides of the same coin in the HTS and phenotypic screening paradigms. It is as pointless to acquire massive chemical libraries that cannot be evaluated in biochemical, cellular, or organismic assays, as it is to have highly automated and efficient methods to screen a biological system without small molecules. Given the traction among several groups to invest in screening platforms, it therefore became necessary to consider the creation of a compound management and logistics facility from a national perspective. A centralized repository that met the national need would clearly benefit from economies of scale that smaller facilities scattered throughout the country could never achieve.
Griffith University took the lead in acquiring this infrastructure in collaboration with other interested stakeholders, partly because there was already a clear need to automate the 70,000 natural product extracts and partly because there was a considerable knowledge base available through AstraZeneca’s Compound Management Network, which was implementing its global, automated compound management facilities. It was fortuitous that planning for the compound management facility coincided with a major national effort in Australia to improve acquisition and access to systemic infrastructure. The scheme, known as the National Collaborative Research Infrastructure Strategy (NCRIS), commenced in 2004 with a broad outline of its scope. Importantly, while the NCRIS scheme aimed to identify and address critical gaps in infrastructure, it also had the remit to increase collaboration among researchers in the same space and reduce the duplication and suboptimal use of resources arising from a lack of coordination. Essentially, the NCRIS program would not provide funds to individual organizations or institutions for the same facilities to be established at multiple sites.
The research community could participate in the process throughout 2005 prior to the release of an Exposure Draft of the Strategic Roadmap. During 2005–2006, grassroots support was received from 14× universities, 6× medical research institutes, 6× publicly funded research agencies [36], and 2× major national resource facilities resulting in a proposal for an integrated compound management and HTS to be included in the priority area of “Translating health discovery into clinical applications” (THD). Although this concept was included in the 2006 Strategic Roadmap [37], the NCRIS Committee noted that responses to the Exposure Draft of the Roadmap indicated not only a strong support for the THD capability area from a large cross-section of the research community but also a diversity of opinions as to appropriate investments. The NCRIS Committee suggested stakeholders continue to work toward clarification of the issues and needs and that this capability be reviewed for possible implementation in 2007.
Concomitantly, the Griffith University group, who was based in Queensland, applied through their State Government’s Smart State Research Facility Fund (SSRFF) in 2005 for the infrastructure. The proposal was successful and ultimately led to the establishment Queensland Compound Library (QCL). This was again fortuitous as the scheme that followed on from NCRIS, the Super Science Initiative (SSI), was delayed until 2008 with in-principle funding earmarked for mid-2012. The postponement was no doubt due, in part, to the global financial crises that afflicted many economies prompting governments to offer stimulus packages and curtail other investments.
Importantly, it should be noted that the SSRFF is not a grant but rather a loan made by the State government to Griffith University. The loan is very generous with repayments commencing after the tenth year and staggered over the next 20 years. Moreover, one-third of the loan is forgiven if the facility reaches agreed Key Performance Indicators (KPIs) so that two-thirds need only be paid in the final 20 years. As part of the loan agreement, the State also owns a percentage of any profits generated by the QCL. This percentage is worked out using a formula that takes into account the up-front investment made by the State and other stakeholders to establish the facility. The SSRFF and Griffith University helped provide resources at a critical time for Australian life science research. Without this scheme, it is doubtful whether a national compound management and logistics facility with an equipment base like that of the QCL would have been established.
It should also be noted that the exchange rate of the AUD to the USD and GBP in 2007, when equipment was being ordered and successively paid, was not particularly strong and did not allow the most comprehensive package to be obtained. The compromises made early on, especially with the sample processing robot, came back to haunt the facility. It was fortuitous then, that the SSI, which had been delayed by 2 years, was reactivated at this critical juncture and the QCL was able to access additional funds. Indeed, the exchange rate from late 2008 onwards improved dramatically and, at the time of writing this chapter, had reached parity, with the USD facilitating the acquisition of an expanded platform.
Stakeholder Involvement
Despite funding from the Queensland government, a road show was nevertheless organized by the Griffith University team during the latter part of 2006 that included stopovers at Melbourne, Sydney, Brisbane, Adelaide, and Canberra in an effort to provide the NCRIS Committee with additional information to clarify outstanding issues and needs from THD stakeholders. The road show provided invaluable feedback from a national perspective. Although much of the feedback was not directly related to establishment of an integrated compound management and HTS capability per se, it did capture the national zeitgeist of what any facility that was established or supported by NCRIS should adhere to. These included
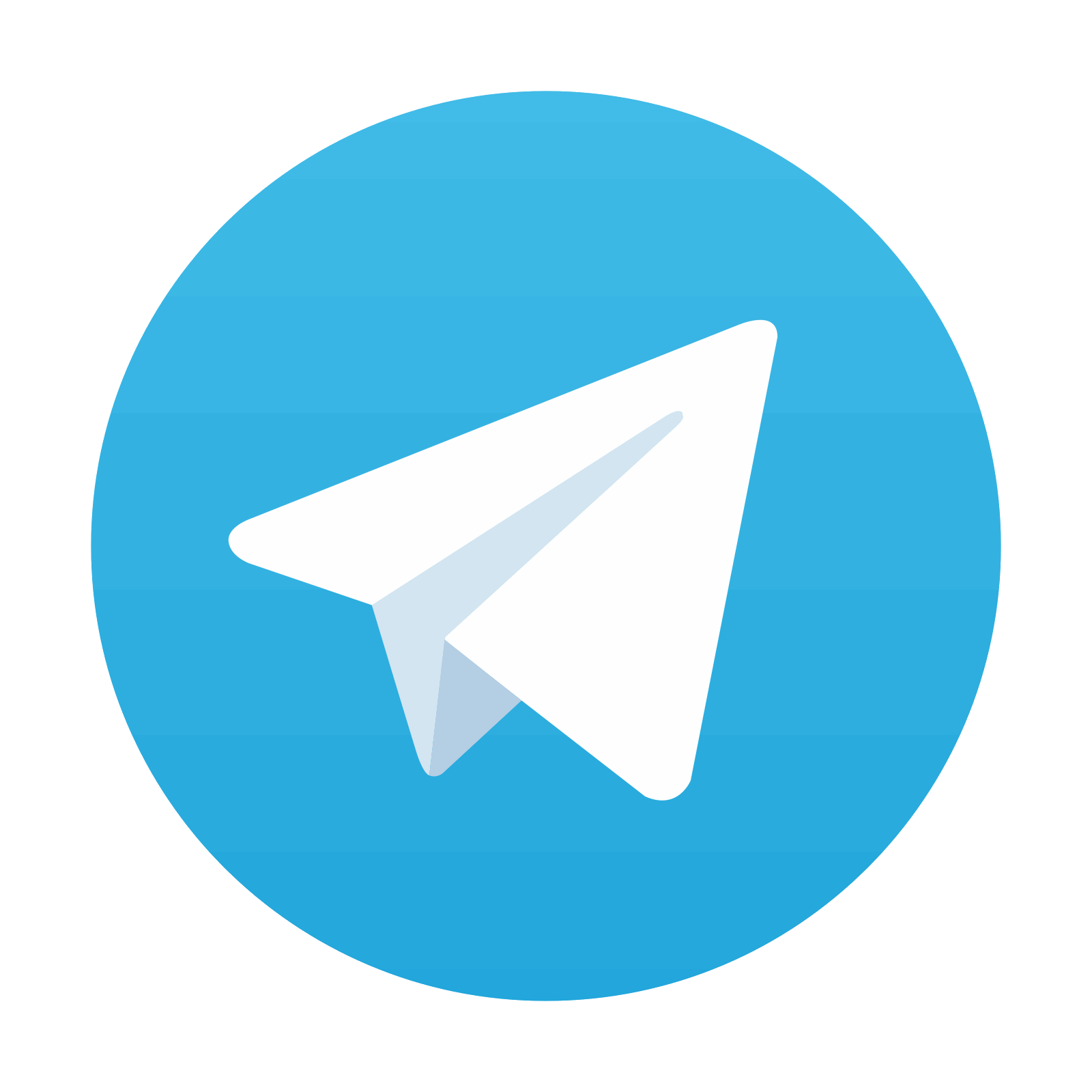
Stay updated, free articles. Join our Telegram channel

Full access? Get Clinical Tree
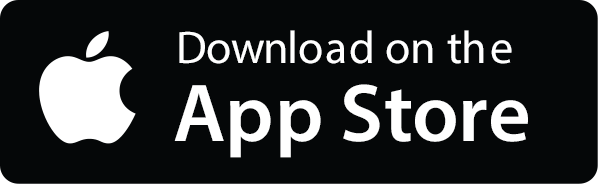
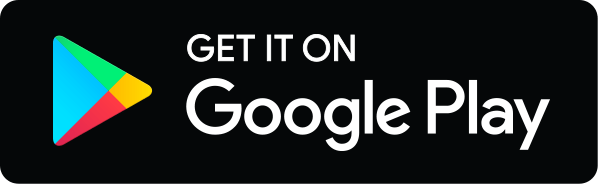