The type 2 secretion and type 4 pilus systems of Escherichia coli
Leon G. De Masi, Courntey D. Sturey, Joshua A. Lieberman and Michael S. Donnenberg, University of Maryland School of Medicine, Baltimore, MD, USA
Introduction
Definition
The type 2 secretion (T2S) and the type 4 pilus (T4P) systems are two broadly conserved systems that play important roles in the virulence of pathogenic Escherichia coli and many other bacteria. These systems have been identified in enteropathogenic E. coli (EPEC), enterotoxigenic E. coli (ETEC), enterohemorrhagic E. coli (EHEC), and non-pathogenic E. coli strains. The T2S system exports exotoxins and important cell-surface anchored lipoprotein enzymes that digest components of the host cell, including proteins, lipids, and sugars (Economou et al., 2006). The T4P system produces pili that are involved in adherence, motility, and aggregation. Both systems consist of multicomponent machines that are broadly distributed but play distinct roles in bacterial pathogenesis. Components of the T2S and T4P machines are homologous in sequence, structure, and function. Only a few key differences account for the ability of T4P systems to anchor polymerized substrates in the inner membrane (IM) as opposed to the secretion of individual, folded substrates across the outer membrane (OM) by T2S systems. Under artificial conditions, T4P systems can assemble T2S pseudopilins into pili (Sauvonnet et al., 2000).
T2S represents one of at least six secretion systems in Gram-negative bacteria that function in the export of proteins to the extracellular environment (Economou et al., 2006). T2S has been referred to as the main terminal branch of the general secretory pathway (Gsp) and the second part of a two-step process. The first step is transport of the exoprotein across the IM by the Sec or Tat pathway (Cianciotto, 2005). The substrate precursor contains an N-terminal signal peptide that targets the protein to be transported through the IM. The signal peptide is cleaved off and the protein is released into the periplasm where it folds into its functional confirmation. The protein is then transported by the T2S across the OM into the extracellular environment through a pore formed by the secretin protein (Genin and Boucher, 1994).
The T2S system in E. coli consists of 12 core proteins found in all T2S systems and two accessory proteins (Sandkvist, 2001). Mutations in genes encoding most of these proteins result in defects in the secretion process and accumulation of exoproteins in the periplasm (Filloux, 2004). There are chromosomal genes in the non-pathogenic E. coli K-12 and most other strains that encode a T2S system; however, under standard conditions secretion does not occur. The operon encoding this system is silenced by the nucleoid-structuring protein H-NS, and mutants deficient for H-NS can express the T2S system (Francetic et al., 2000). In E. coli pathogens, proteins secreted by accessory T2S systems play multiple roles, including adherence to eukaryotic cells and damage to host tissues. Examples include the EHEC StcE metalloprotease, which promotes adherence to host eukaryotic cells (Grys et al., 2005) and the ETEC heat-labile toxin (LT), a multimeric AB5 enterotoxin functionally and structurally similar to cholera toxin of Vibrio cholerae (Sixma et al., 1993) that induces fluid secretion and promotes colonization of intestinal epithelia (Tauschek et al., 2002).
T4P are filamentous surface appendages required for adherence, motility, aggregation, and transformation in a wide array of bacterial and archaeal species. T4P were first identified in Pseudomonas aeruginosa and Myxococcus xanthus (Ottow, 1975). Further studies revealed that all T4P have a conserved set of core proteins (Pelicic, 2008). T4P are long, thin, flexible, polymeric, three-start helical filaments approximately 85 Å in diameter (Craig et al., 2004; Ramboarina et al., 2005). The pili are composed primarily of the major pilin protein (Craig et al., 2004), and are assembled by a complex biogenesis machine consisting of 10–18 proteins that spans both bacterial membranes (Sohel et al., 1996; Stone et al., 1996; Hwang et al., 2003; Peabody et al., 2003; Pelicic, 2008). Major pilins are first synthesized as precursor prepilins and then processed by a prepilin peptidase to create mature pilin proteins incorporated into the pilus (Strom et al., 1993).
T4P can be subdivided into two distinct classes, type 4 class A pili (T4aPs) and type 4 class B pili (T4bPs), based on differences in component structure, genetic organization, distribution, and function. T4bPs are characterized by larger major pilins compared to those of T4aPs that begin with an amino acid other than phenylalanine and are encoded in a contiguous operon often found on plasmids (Craig et al., 2004). As with the common T2S system, most strains of E. coli have the genes for a T4aP that has been called, somewhat confusingly, the hemorrhagic coli pilus (HCP) (Xicohtencatl-Cortes et al., 2007). In addition, some E. coli pathotypes express accessory T4bPs that play important roles in infection.
History
The T2S system was initially discovered in Klebsiella oxytoca and Pseudomonas aeruginosa and is widely conserved among Gram-negative bacteria (Filloux et al., 1990). Sequencing of plasmid pO157 from EHEC identified a T2S system conserved among all EHEC O157 and some non-O157 strains (Schmidt et al., 1997). A silenced T2S operon was also found in an E. coli K-12 strain (Blattner et al., 1997). This system can secrete endochitinase ChiA when the silencing protein is inactivated (Francetic et al., 2000). In ETEC a T2S system highly similar to that responsible for cholera toxin secretion in Vibrio cholerae was discovered to secrete LT (Tauschek et al., 2002). This gene cluster is absent from E. coli K12, however the K12 genome appears to have once contained this sequence.
Although discovered years earlier in other bacteria, T4P where first identified in E. coli with the discovery of the EPEC bundle-forming pilus (BFP) (Girón et al., 1991). Subsequent identification of the gene encoding the major structural subunit confirmed its similarity to other T4P pilin genes and suggested the presence of a large operon encoding genes required for pilus processing and biogenesis (Donnenberg et al., 1992). Additional E. coli T4P were discovered subsequently.
Distribution
Both T2S and T4P are produced by a diverse number of Gram-negative bacteria including many plant, animal, and human pathogens. Within E. coli, one T2S system and the HCP are ubiquitous, while additional T2S and T4P systems are found on specific subsets of pathotypes (Girón et al., 1993, 1994; Schmidt et al., 1997; Tauschek et al., 2002).
The components of T4P systems share significant sequence similarity and structural homology with components of T2S systems, DNA uptake systems (Averhoff and Friedrich, 2003; Peabody et al., 2003), and filamentous phage assembly systems (Linderoth et al., 1996; Russel et al., 1997). T4P assembly components also are orthologous to proteins involved in archaeal flagellum assembly (Peabody et al., 2003). The sequence and structural similarities across such a wide range of organisms strongly suggest an ancient and shared evolutionary history (Peabody et al., 2003; Pelicic, 2008). T4Ps have also been found in Gram-positive (Varga et al., 2006; Rodgers et al., 2011) and archaeal species (Herdendorf et al., 2002; Bardy and Jarrell, 2003).
Only a few T4bP systems have been identified in E. coli, where some are virulence factors used for adherence to eukaryotic cells, an essential first step for colonization. This is evident in the decreased virulence of EPEC strains deficient for functional pili (Bieber et al., 1998). The best-characterized system is the BFP of EPEC. Through the remainder of this chapter we will refer to the BFP system as the model of T4bP biogenesis, referring to other systems primarily to highlight differences when they occur. In addition to BFP, two related T4Ps have been identified in ETEC, the colonization factor antigen III (CFA/III) and Longus systems. Another system called the R64 pilus is encoded on a plasmid, and a T4aP called the hemorrhagic coli pilus or HCP has been described in EHEC, although the genes encoding HCP are ubiquitous among E. coli strains (Xicohtencatl-Cortes et al., 2007).
BFP fibers are produced from an operon of 14 genes encoded on the large (∼90 kb) adherence factor plasmid (pEAF) (Nataro et al., 1987; Stone et al., 1996). The BFP is a confirmed virulence factor (Bieber et al., 1998) that mediates the initial stages of adherence to the host intestinal epithelium (Cleary et al., 2004; Hyland et al., 2006a; Zahavi et al., 2011). CFA/III fibers are peritrichous pili that are 5–10 µm long and are often encoded on a large plasmid (Honda et al., 1984; Shinagawa et al., 1993). Some ETEC strains produce the Longus pilus, so named because it can grow over 20 µm in length, which is essentially allelic to CFA/III (Girón et al., 1994). The Longus T4P display a polar distribution on the cell surface (Girón et al., 1994). The N-termini of the major pilin proteins in each system, CofA and LngA, and of all accessory genes are nearly identical (Taniguchi et al., 1995; Gomez-Duarte et al., 2007). Despite their similarity, these pilus systems are expressed under different conditions and by different serogroups of ETEC (McConnell et al., 1989; Girón et al., 1995). The R64 pilus is encoded on a large conjugative plasmid. Unlike its counterparts in EPEC and ETEC, the R64 pilus has not been shown to have a role in adherence but rather is specialized for bacterial conjugation in liquid media (Yoshida et al., 1998). The HCP can grow to over 10 µm in length and is involved in adherence of EHEC to host cells (Xicohtencatl-Cortes et al., 2007).
Genetic organization
The genetic organization of T2S systems is relatively well conserved. For all T2S systems there are 12 conserved genes, termed A–O, although in some cases additional genes may be required for T2S function (Peabody et al., 2003; Filloux, 2004). These genes are most often found in a single operon with slight variations depending on species (Sandkvist, 2001). The majority of T2S systems are located on the chromosome with the exception of a plasmid-encoded T2S system in E. coli O157 (Cianciotto, 2005).
Most T4P expressed in E. coli are T4bP and thus are found in a continuous operon on a large plasmid (Figure 13.1). The exception, the HCP pilus characterized in EHEC, is a T4aP and is thus encoded on several small operons in the E. coli genome, though little is known about the function of each gene in these operons outside of hcpA (also known as ppdD), the main pilus subunit (Xicohtencatl-Cortes et al., 2007). In EPEC, the bfp operon is found on pEAF (Sohel et al., 1996; Stone et al., 1996), and of the 14 genes, all except for bfpH must be expressed for BFP expression and function (Anantha et al., 2000). The operon begins with bfpA, which encodes the main pilus subunit precursor, pre-bundlin, and ends with bfpL. Expression of the bfp operon is controlled by perA, an AraC-family transcriptional activator (Gómez-Duarte and Kaper, 1995; Tobe et al., 1996).
Like the bfp operon, the ETEC T4P systems Longus and CFA/III are encoded by a contiguous operon controlled by a perA-like promoter and found on a very large plasmid (Taniguchi et al., 1995, 2001; Gomez-Duarte et al., 2007). In both cases, the order of the genes differs substantially from that of the bfp operon, bearing more similarity to the tcp operon from Vibrio cholerae (Kirn et al., 2003). Another contiguous operon encodes the R64 conjugative pilus and is found on the plasmid of the same name (Kim and Komano, 1997; Sakai and Komano, 2002). Here, the genes encoding the putative tip adhesins are organized in a shufflon located at the end of the operon and the order of the genes encoding the assembly proteins differs markedly from both the BFP operon and the Longus/CFA/III operons. The shufflon is comprised of four DNA segments flanked by inverted repeat sequences. This allows for site-specific recombination between repeats and the formation of one of seven functional gene products, which have different LPS binding capabilities (Ishiwa and Komano, 2004).
Regulation
T2S systems are regulated through specific quorum sensing and constitutive expression mechanisms depending upon the species. Regulation of the ETEC T2S system is under the control of the yghJ promoter, which is repressed by global regulatory proteins H-NS and StpA (Yang et al., 2007). It is expected that these proteins bind directly to the promoter region inhibiting open complex formation.
Multiple sensory and regulatory systems regulate expression of the BFP. The pst operon responds to inorganic phosphate starvation and positively regulates elaboration of the BFP machinery via the per operon (Ferreira and Spira, 2008). The gene products of the per operon, PerA, PerB, and PerC, directly augment transcription of BFP genes (Tobe et al., 1999) and of a second transcriptional activator, Ler that in turn regulates additional EPEC genes involved in type 3 secretion (T3S) (Elliott et al., 2000) (see Chapter 4). Additionally, BFP receptor binding and retraction induce transient up-regulation of bfp transcription and down-regulates expression of Ler-induced virulence genes (Humphries et al., 2010). Retraction of BFP fibers is also required for efficient translocation of effector molecules secreted by this pathway (Zahavi et al., 2011).
Bacterial stress response pathways play an important role in regulating the expression of virulence factors in Gram-negative pathogens and the BFP is no exception (Nevesinjac and Raivio, 2005; MacRitchie et al., 2008; Vogt et al., 2010; Lieberman et al., 2012). In the case of the BFP system, full activation of the Cpx envelope stress response pathway represses transcription of BFP genes and inhibits pilus expression (Vogt et al., 2010). However, complete inactivation of the Cpx pathway in EPEC eliminates pilus biogenesis (Nevesinjac and Raivio, 2005) due to insufficient levels of periplasmic chaperone proteins such as DegP, CpxP, and DsbA (Vogt et al., 2010). A previous study revealed DsbA was required for stability of bundlin and BFP biogenesis (Zhang and Donnenberg, 1996). BFP can be expressed in K12 E. coli when the cloned bfp operon is placed under control of a strong promoter (Stone et al., 1996), but sufficient BFP production to allow associated phenotypes requires constitutive activation of Cpx (Price and Raivio, 2009).
Structural components of T2S and T4P machines
Introduction
The T2S and T4P machines are structurally and functionally homologous (Craig et al., 2004; Ayers et al., 2009; Korotkov et al., 2012). These complexes can be conceptualized as consisting of three distinct subcomplexes: the inner membrane (IM) subassembly associated with at least one cytoplasmic ATPase; the OM subassembly which consists of a transmembrane pore; and the translocated substrate. Remarkably few differences between the T2S and T4P machines account for their capacity to process very different substrates: the T2S extrudes fully folded proteins likely driven through the pore by a pseudopilus while the T4P system substrate is itself a pilus. Variations and accessory proteins do exist and are discussed after the conserved components. All components are listed using the General Secretory Pathway (Gsp) nomenclature used for ETEC (Table 13.1).
The IM subassembly
The IM subassemblies of both the T2S and T4P machines consist of a core set of homologous components, including several integral IM proteins and a hexameric ATPase that provides the energy driving secretion (Pelicic, 2008). A polytopic IM protein, GspF, is not only well-conserved in the T4P and T2S machines, but also has homologs in the archaeal flagella system and Gram-positive competence systems (Peabody et al., 2003). A bitopic transmembrane protein, GspL, interacts in the IM with GspM, another bitopic protein that is predominantly periplasmic (Sandkvist et al., 1999, 2000; Abendroth et al., 2004a, 2009a). The cytoplasmic domain of GspL interacts with the hexameric ATPase, GspE (Abendroth et al., 2005; Camberg and Sandkvist, 2005; Camberg et al., 2007). In addition, the T2S IM complex includes a membrane-anchored periplasmic protein, GspC, which interacts with the secretin of the system (Korotkov et al., 2006; Lybarger et al., 2009).
The IM complex of T4P biogenesis machines is very similar. Using the BFP of EPEC as a prototype, there is also a polytopic IM protein, BfpE (Blank and Donnenberg, 2001). The T4P system has a bitopic IM protein BfpC (Milgotina et al., 2011; Yamagata et al., 2012). These two proteins interact with each other as well as with BfpD, an ATPase that powers pilus extension (Milgotina et al., 2011). Remarkably, despite the absence of sequence similarity, the cytoplasmic domain of BfpC is a structural and functional homolog of GspL and of the T4aP component PilM (Yamagata et al., 2012). The Longus and CFA/III pili of ETEC have the same core IM proteins as BFP (Table 13.1). The same core components are also found in R64, however this system has several IM proteins with unknown function (Kim and Komano, 1997). BfpL is a bitopic IM protein essential for BFP biogenesis that interacts with BfpC, but homologs of BfpL have not yet been described in other systems (De Masi et al., 2012).
Although the core transmembrane proteins and ATPase are present in both T2S and T4P systems there are several important differences. At present, no homolog for GspC, which interacts with the secretin and is essential for stability of the IM complex, has been found in an E. coli T4P system. Also, unlike T2S systems, most T4P systems have the capacity to rapidly retract their pilus fibers, and BFP is no exception. This is accomplished through a dedicated retraction ATPase, BfpF (Anantha et al., 1998; Bieber et al., 1998). However, an equivalent in the T4P systems of ETEC has not been found.
The OM subassembly
The T2S and T4P systems encounter a common problem in exporting substrates: crossing the OM. Both machines solve this problem with pores formed by proteins in the secretin superfamily. Secretins form multimeric pores in the OM, most often consisting of 12 monomers, each of which is believed to be a fully folded beta barrel (Korotkov et al., 2011). Many of these proteins require a small lipoprotein, termed a pilotin, for stability and successful transport to the OM. In the T2S system, the OM pore is formed by a dodecamer of the secretin, GspD (Genin and Boucher, 1994; Reichow et al., 2010). This complex is stabilized by the pilotin, GspS (Hardie et al., 1996). The T4P OM complex also includes a secretin, BfpB (Ramer et al., 1996; Schmidt et al., 2001). However, the BFP secretin is itself a lipoprotein (Ramer et al., 1996) and can assemble and reach the OM when expressed alone (Daniel et al., 2006; Lieberman et al., 2012). To date no pilotin has been identified in the BFP system. Four secretins in both T2S and T4P systems have been experimentally validated as lipoproteins and none appear to have or require cognate pilotins (Hu et al., 1995; Ramer et al., 1996; Schmidt et al., 2001; Bose and Taylor, 2005; Viarre et al., 2009). Interestingly, the secretins of CFA/III, Longus, and R64 systems all contain canonical lipobox sequences (LxxC) in their N-termini and are likely liposecretins (Wu and Tokunaga, 1986; Viarre et al., 2009). Additionally, none of the other E. coli T4P operons appears to include pilotin genes, further suggesting that the secretins are all self-sorting lipoproteins.
Beyond the lack of a cognate pilotin, the T4P OM complex is noticeably different from the T2S system by virtue of two unique proteins. Both are soluble, periplasmic proteins that associate with the OM in the presence of the secretin (Daniel et al., 2006). BfpG is the product of the second gene in the BFP operon (Schmidt et al., 2001; Daniel et al., 2006). The second, BfpU, is found in both the cytoplasm and periplasm and has no apparent T2S or T4aP homologs (Schreiber et al., 2002). Both of these proteins are essential for pilus biogenesis and interact with BfpB to form the OM subassembly (Daniel et al., 2006). Interactions between the BfpU and BfpG have not been described.
Pseudopilus and pilus
According to generally accepted theory, both the T2S and T4P build filamentous appendages that move through a central channel formed by the IM and OM subassemblies. The T2S builds a pseudopilus that is of sufficient length to reach the vestibule formed by the secretin (Reichow et al., 2011). The pseudopilus is primarily composed of GspG, the major pseudopilin (Sauvonnet et al., 2000; Durand et al., 2003) and a set of two to four minor pseudopilins depending upon the system. In ETEC the four minor pseudopilins are GspH, I, J, and K (Yanez et al., 2008a,b). All pseudopilins share N-terminal sequence homology and are processed by a prepilin peptidase GspO (Bally et al., 1992; Dupuy et al., 1992).
The T4P forms a structure that protrudes through the secretin and serves as a fimbrial adhesin. The main pilus subunit precursor, pre-bundlin for BFP, is cleaved by the prepilin peptidase, BfpP (Zhang et al., 1994) to form the primary component of the pilus. The solution structure of bundlin lacking the N-terminal stretch of hydrophobic amino acids predicted to be buried in the pilus core has been solved. Core features common to all T4P pilins and T2S pseudopilins, including the N-terminal alpha helix, the variable alpha-beta region, and the antiparallel beta sheet, are apparent (Figure 13.2). However, the nature of the alpha-beta region, the topological arrangement of the beta strands in the sheet, and the enclosure of the sheet in alpha helices is unique. These features contrast, not only with those of T4aP pilin proteins, but even with T4bP pilin proteins such as CofA (Fukakusa et al., 2012; Kolappan et al., 2012), with which it shares only the most conserved pilin features (Figure 13.2).
FIGURE 13.2 Structures of bundlin and CofA highlight similarities and differences among T4bP pilin proteins. Structures of soluble, N-terminally truncated versions of (A) bundlin (PDB 1zwt) and (B) CofA (PDB 3vor). The hydrophobic amino termini of both proteins, predicted to form contiguous alpha helices buried in the core of the pili, were omitted to facilitate purification and structure determination (Ramboarina et al., 2005; Fukakusa et al., 2012; Kolappan et al., 2012). Conserved features include the N-terminal alpha helix (color) and the central anti-parallel beta sheet (sheet). When overlaid (C), marked differences between bundlin (blue) and CofA (green) are apparent. Figures were drawn using PyMOL (The PyMOL Molecular Graphics System, Version 1.3, Schrödinger, LLC) by Kurt Piepenbrink.
T4P systems also contain analogs of the minor pseudopilins called pilin-like proteins: BfpI, BfpJ, and BfpK. These proteins, like the minor pilins in other T4P systems, contain the same peptidase cleavage site as bundlin and are processed by the prepilin peptidase (Ramer et al., 2002). Like the pseudopilins, the pilin-like proteins may form a complex with one another (Koomey, 1995; Ramer et al., 2002; Helaine et al., 2007). Immunoelectron microscopy has confirmed that BfpI is a true minor pilin.
There are several important differences between the T4P systems in E. coli. Unlike BFP, the CFA/III, Longus and R64 systems contain only one pilin-like protein (Gómez-Duarte et al., 1999; Yoshida et al., 1999; Taniguchi et al., 2001). Each of these is much larger in size than the pilin-like proteins in BFP. Second, the first amino acid after the prepilin peptidase cleavage site of BfpA is leucine and, as is the case with most T4P systems, is N-methylated (Donnenberg, 2012). However, in the R64 major pilin tryptophan is the first amino acid after the peptidase cleavage site and undergoes a distinct, but uncharacterized modification (Yoshida et al., 1998).
Mechanism of action
Structure and function of type 2 secretion systems in E. coli
The T2S pseudopilus is believed to interact with substrate exoproteins in the periplasmic vestibule of the secretin and force the exoprotein into the extracellular space through an as yet undocumented piston mechanism (Reichow et al., 2011). The pseudopilus consists of the major pseudopilin, GspG, and minor pseudopilins GspH, I, J, and K, all of which are translocated across the IM via the Sec-dependent pathway (Francetic et al., 2007). A short, positively charged segment at the N-terminal region of each pseudopilin is cleaved off by the prepilin peptidase GspO (Bally et al., 1992; Dupuy et al., 1992). The peptidase is an aspartic acid protease with eight putative transmembrane helices and its catalytic residues in cytoplasmic loops (LaPointe and Taylor, 2000). The enzyme is bifunctional and also N-methylates the cleavage product (Strom et al., 1993). The pseudopilins GspI, J, and K appear to be incorporated as a trimer at the tip of the pseudopilus (Korotkov and Hol, 2008; Douzi et al., 2009) with GspK at the top and GspI/J forming the base. How monomers of GspG and the pseudopilin GspH are added to the pseudopilus remains unknown. All five pseudopilins have markedly different globular structures but share common features: an N-terminal alpha helix (the first half of which is hydrophobic) followed by a variable region and a conserved beta-sheet that varies in length with the various proteins.
T2S biogenesis requires the insertion of the IM proteins, GspC, F, L, and M, into the IM. These proteins create a complex together and appear to protect each other from proteolysis (Sandkvist et al., 1999). GspC contains a short cytoplasmic segment, a transmembrane helix, and two periplasmic domains: the homology region (HR) and PDZ domains (Bleves et al., 1999) although the PDZ domain is not universal across all T2S systems (Korotkov et al., 2006). The HR domain of GspC interacts with the periplasmic N-terminal regions of the secretin, GspD (Korotkov et al., 2011). The HR domain may also influence formation of the IM complex (Lybarger et al., 2009). GspF is the only polytopic protein in the complex, consisting of two cytoplasmic domains sharing some sequence homology and three transmembrane helices (Abendroth et al., 2009a,b). As discussed above, this polytopic transmembrane protein is one of the most highly conserved members of the T2S system and related machines (Peabody et al., 2003).
The GspL component recruits pseudopilins processed by GspO, the prepilin peptidase, to the IM complex (Sandkvist et al., 1995) and also recruits the ATPase, GspE, to the cytoplasmic face of the IM (Sandkvist et al., 2000; Abendroth et al., 2005). GspE is a member of a large ATPase family (Robien et al., 2003) providing the necessary energy for the secretion apparatus to function through ATP hydrolysis and, unlike most other proteins of its type contains zinc (Camberg and Sandkvist, 2005). The protein contains typical Walker boxes A and B (Planet et al., 2001) and is active as a hexamer (Satyshur et al., 2007). GspM contains a short cytoplasmic sequence, a transmembrane helix, and a ferrodoxin fold in its periplasmic domain that could serve as a binding site for another protein (Abendroth et al., 2004b). GspM interacts directly with GspL through contacts in the transmembrane domain and periplasm (Sandkvist et al., 1999; Py et al., 2001).
The secretin GspD is part of a large protein superfamily of multimeric, pore-forming OM proteins (Linderoth et al., 1996; Collins et al., 2004; Reichow et al., 2010). Although variations exist, some common themes of secretin structure have emerged. Like many of the secretin family members, GspD forms a dodecameric ring structure in the OM approximately 200 Å in height and 150–160 Å in width with a central pore through which exoprotein substrates pass (Korotkov et al., 2009; Reichow et al., 2010). The periplasmic side of the pore consists of a chamber that appears to serve as a docking site for secreted exoproteins (Reichow et al., 2011) with an opening of ∼70 Å, which narrows to ∼50 Å at a periplasmic gate (Korotkov et al., 2009; Reichow et al., 2010, 2011). The periplasmic chamber is formed by concentric rings composed of the N-termini of the monomers. How the secretin accommodates the passage of substrate proteins through the gates and narrow center of the channel, and how it prevents secretion of other molecules that are not substrates, remains unknown. The pore opens into a smaller chamber on the extracellular face that is capped. Since GspD is not a liposecretin, the pilotin GspS is required for secretin stability and transport to the OM via an interaction with the distal C-terminus of the secretin (Daefler et al., 1997; Nickerson et al., 2011). GspS is itself a lipoprotein that is acylated and transported to the OM via the Lol pathway (Collin et al., 2011).
Despite accumulating knowledge regarding the structures of the various T2S components, the actual mechanism of secreting a protein like LT of ETEC through the T2S apparatus has not been well established. However, a theoretical process has been proposed. Initially, the target proteins are synthesized in the cytoplasm and translocated via the Sec or Tat export pathways depending on whether the protein is folded in the periplasm or cytoplasm, respectively (Pugsley, 1993; Berks et al., 2005). Molecular modeling strongly suggests an interaction between the correctly folded substrate protein, the secretin, the pseudopilus tip complex of Gsp I/J/K, and GspC (Reichow et al., 2011), although interactions with additional machine components are possible. The binding of the exoprotein then stimulates the ATPase activity of GspE, leading to the addition of GspG monomers to the pseudopilus (Hobbs and Mattick, 1993; Shevchik et al., 1997). It is not known how the system chooses substrates from the plethora of periplasmic proteins available, or how the ATPase is specifically activated by substrate binding. The exoprotein is pushed upward through the secretin pore with the pseudopilus acting like a piston. However, it is not known how the pseudopilus is retracted. The trimer tip of the pseudopilus may interact with the secretin pore, inducing a conformational change destabilizing the GspG monomers below it, causing the pseudopilus to depolymerize (Korotkov et al., 2012). It notable that the degree of expression of the equivalent of GspK in the Pseudomonas aeruginosa T2S is inversely proportional to the length of the pseudopilus and an interaction between the GspK and GspG equivalent subunits destabilizes GspG (Durand et al., 2005).
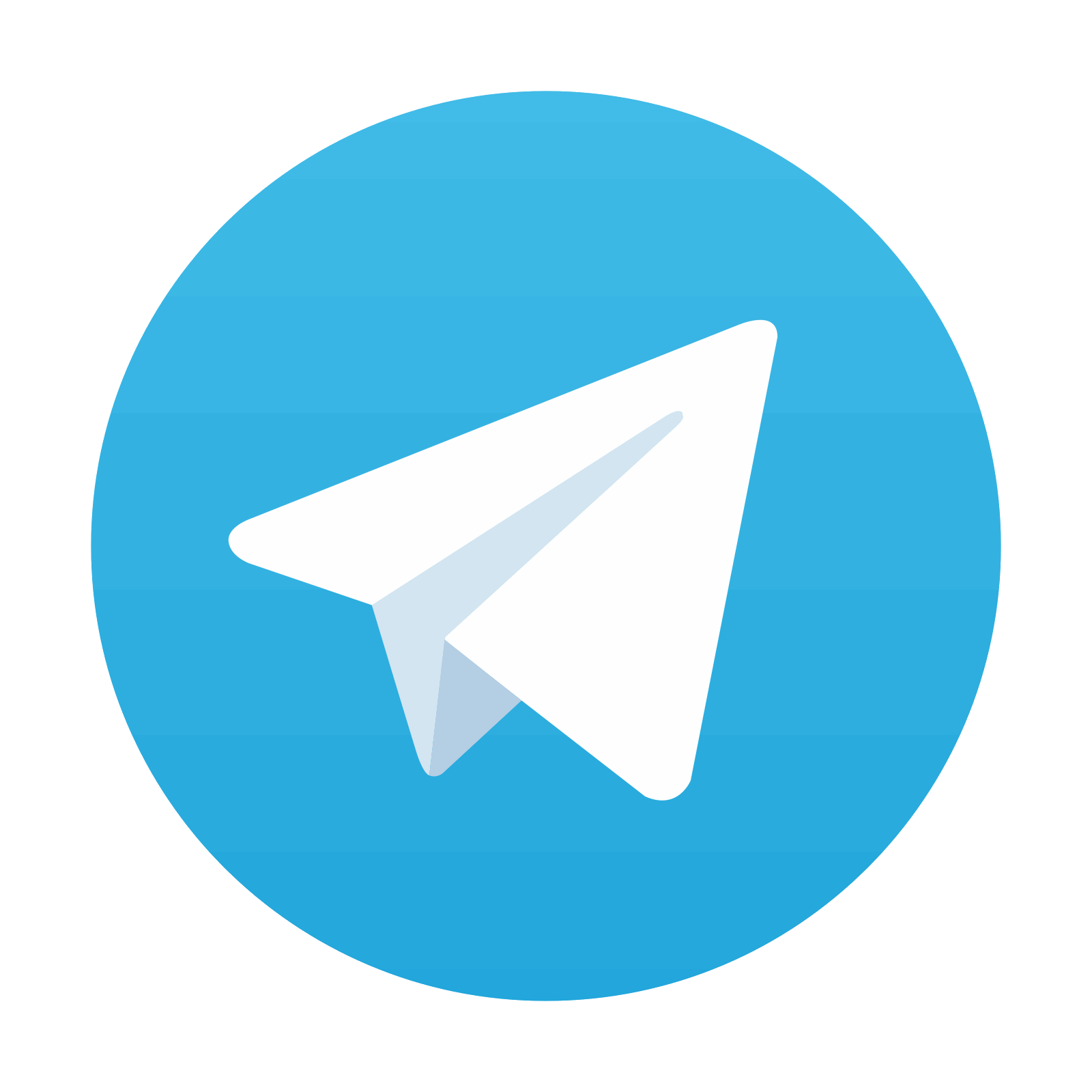
Stay updated, free articles. Join our Telegram channel

Full access? Get Clinical Tree
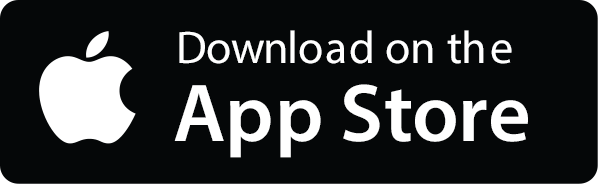
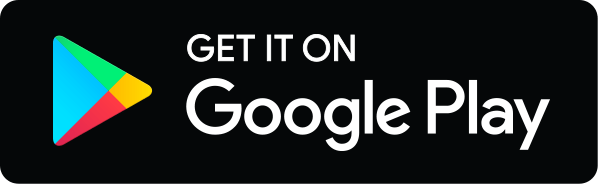