OBJECTIVES
After studying this chapter, you should be able to:
Describe the structure of the thyroid gland and how it relates to its function.
Define the chemical nature of the thyroid hormones and how they are synthesized.
Understand the critical role of iodine in the thyroid gland and how its transport is controlled.
Describe the role of protein binding in the transport of thyroid hormones and peripheral metabolism.
Identify the role of the hypothalamus and pituitary in regulating thyroid function.
Define the effects of the thyroid hormones in homeostasis and development.
Understand the basis of conditions where thyroid function is abnormal and how they can be treated.
INTRODUCTION
The thyroid gland is one of the larger endocrine glands of the body. The gland has two primary functions. The first is to secrete the thyroid hormones, which maintain the level of metabolism in the tissues that is optimal for their normal function. Thyroid hormones stimulate O2 consumption by most of the cells in the body, help regulate lipid and carbohydrate metabolism, and thereby influence body mass and mentation. Consequences of thyroid gland dysfunction depend on the life stage at which they occur. The thyroid is not essential for life, but its absence or hypofunction during fetal and neonatal life results in severe mental retardation and dwarfism. In adults, hypothyroidism is accompanied by mental and physical slowing and poor resistance to cold. Conversely, excess thyroid secretion leads to body wasting, nervousness, tachycardia, tremor, and excess heat production. Thyroid function is controlled by the thyroid-stimulating hormone (TSH, thyrotropin) of the anterior pituitary. The secretion of this hormone is in turn increased by thyrotropin-releasing hormone (TRH) from the hypothalamus and is also subject to negative feedback control by high circulating levels of thyroid hormones acting on the anterior pituitary and the hypothalamus.
The second function of the thyroid gland is to secrete calcitonin, a hormone that regulates circulating levels of calcium. This function of the thyroid gland is discussed in Chapter 21 in the broader context of whole body calcium homeostasis.
ANATOMIC CONSIDERATIONS
The thyroid is a butterfly-shaped gland that straddles the trachea in the front of the neck. It develops from an evagination of the floor of the pharynx, and a thyroglossal duct marking the path of the thyroid from the tongue to the neck sometimes persists in the adult. The two lobes of the human thyroid are connected by a bridge of tissue, the thyroid isthmus, and there is sometimes a pyramidal lobe arising from the isthmus in front of the larynx (Figure 19–1). The gland is well vascularized, and the thyroid has one of the highest rates of blood flow per gram of tissue of any organ in the body.
The portion of the thyroid concerned with the production of thyroid hormone consists of multiple acini (follicles). Each spherical follicle is surrounded by a single layer of polarized epithelial cells and filled with pink-staining proteinaceous material called colloid. Colloid consists predominantly of the glycoprotein, thyroglobulin. When the gland is inactive, the colloid is abundant, the follicles are large, and the cells lining them are flat. When the gland is active, the follicles are small, the cells are cuboid or columnar, and areas where the colloid is being actively reabsorbed into the thyrocytes are visible as “reabsorption lacunae” (Figure 19–2).
Microvilli project into the colloid from the apexes of the thyroid cells and canaliculi extend into them. The endoplasmic reticulum is prominent, a feature common to most glandular cells, and secretory granules containing thyroglobulin are seen (Figure 19–3). The individual thyroid cells rest on a basal lamina that separates them from the adjacent capillaries. The capillaries are fenestrated, like those of other endocrine glands (see Chapter 31).
FIGURE 19–3
Thyroid cell. Left: Normal pattern. Right: After TSH stimulation. The arrows on the right show the secretion of thyroglobulin into the colloid. On the right, endocytosis of the colloid and merging of a colloid-containing vacuole with a lysosome are also shown. The cell rests on a capillary with gaps (fenestrations) in the endothelial wall.
FORMATION & SECRETION OF THYROID HORMONES
The primary hormone secreted by the thyroid is thyroxine (T4), along with much lesser amounts of triiodothyronine (T3). T3 has much greater biologic activity than T4 and is specifically generated at its site of action in peripheral tissues by deiodination of T4 (see below). Both hormones are iodine-containing amino acids (Figure 19–4). Small amounts of reverse triiodothyronine (3,3′,5′-triiodothyronine, RT3) and other compounds are also found in thyroid venous blood. Whether RT3 is biologically active remains unclear.
Iodine is an essential raw material for thyroid hormone synthesis. Dietary iodide is absorbed by the intestine and enters the circulation; its subsequent fate is summarized in Figure 19–5. The minimum daily iodine intake that will maintain normal thyroid function is 150 μg in adults. In most developed countries, supplementation of table salt means that the average dietary intake is approximately 500 μg/day. The principal organs that take up circulating I– are the thyroid, which uses it to make thyroid hormones, and the kidneys, which excrete it in the urine. About 120 μg/day enter the thyroid at normal rates of thyroid hormone synthesis and secretion. The thyroid secretes 80 μg/day in the form of T3 and T4, while 40 μg/day diffuses back into the extracellular fluid (ECF). Circulating T3 and T4 are metabolized in the liver and other tissues, with the release of a further 60 μg of I– per day into the ECF. Some thyroid hormone derivatives are excreted in the bile, and some of the iodine in them is reabsorbed (enterohepatic circulation), but there is a net loss of I– in the stool of approximately 20 μg/day. The total amount of I– entering the ECF is thus 500 + 40 + 60, or 600 μg/day; 20% of this I– enters the thyroid, whereas 80% is excreted in the urine.
The basolateral membranes of thyrocytes facing the capillaries contain a symporter that transports two Na+ ions and one I– ion into the cell with each cycle, against the electrochemical gradient for I–. This Na+/I– symporter (NIS) is capable of producing intracellular I– concentrations that are 20–40 times as great as the concentration in plasma. The process involved is secondary active transport (see Chapter 2), with the energy provided by active transport of Na+ out of thyroid cells by Na, K ATPase. NIS is regulated both by transcriptional means and by active trafficking into and out of the thyrocyte basolateral membrane; in particular, thyroid-stimulating hormone (TSH; see below) induces both NIS expression and the retention of NIS in the basolateral membrane, where it can mediate sustained iodide uptake.
Iodide must also exit the thyrocyte across the apical membrane to access the colloid, where the initial steps of thyroid hormone synthesis occur. This transport step is believed to be mediated, at least in part, by a Cl–/I– exchanger known as pendrin. This protein was first identified as the product of the gene responsible for the Pendred syndrome, which causes thyroid dysfunction and deafness. Pendrin (SLC26A4) is one member of the larger family of SLC26 anion exchangers.
The relation of thyroid function to iodide is unique. As discussed in more detail below, iodide is essential for normal thyroid function, but iodide deficiency and iodide excess both inhibit thyroid function.
The salivary glands, the gastric mucosa, the placenta, the ciliary body of the eye, the choroid plexus, the mammary glands, and certain cancers derived from these tissues also express NIS and can transport iodide against a concentration gradient, but the transporter in these tissues is not affected by TSH. The physiologic significance of all these extrathyroidal iodide-concentrating mechanisms is obscure, but they may provide pathways for radioablation of NIS-expressing cancer cells using iodide radioisotopes. This approach is also useful for the ablation of thyroid cancers.
At the interface between the thyrocyte and the colloid, iodide undergoes a process referred to as organification. First, it is oxidized to iodine, and then incorporated into the carbon 3 position of tyrosine residues that are part of the thyroglobulin molecule in the colloid (Figure 19–6). Thyroglobulin is a glycoprotein made up of two subunits and has a molecular weight of 660 kDa. It contains 10% carbohydrate by weight. It also contains 123 tyrosine residues, but only 4–8 of these are normally incorporated into thyroid hormones. Thyroglobulin is synthesized in the thyroid cells and secreted into the colloid by exocytosis of granules. The oxidation and reaction of iodide with the secreted thyroglobulin is mediated by thyroid peroxidase, a membrane-bound enzyme found in the thyrocyte apical membrane. The thyroid hormones so produced remain part of the thyroglobulin molecule until needed. As such, colloid represents a reservoir of thyroid hormones, and humans can ingest a diet completely devoid of iodide for up to 2 months before a decline in circulating thyroid hormone levels is seen. When there is a need for thyroid hormone secretion, colloid is internalized by the thyrocytes by endocytosis, and directed toward lysosomal degradation. Thus, the peptide bonds of thyroglobulin are hydrolyzed, and free T4 and T3 are discharged into cytosol and thence to the capillaries (see below). Thyrocytes thus have four functions: They collect and transport iodine, they synthesize thyroglobulin and secrete it into the colloid, they fix iodine to the thyroglobulin to generate thyroid hormones, and they remove the thyroid hormones from thyroglobulin and secrete them into the circulation.
FIGURE 19–6
Outline of thyroid hormone biosynthesis. Iodide (I–) is transported vectorially from the plasma across the epithelial cells of the thyroid gland by specific transporters. The iodide is converted to iodine, which reacts with tyrosine residues exposed on the surface of thyroglobulin molecules resident in the colloid. Iodination of tyrosine takes place at the apical border of the thyroid cells while the tyrosine moieties remain bound to thyroglobulin via peptide linkages.
Thyroid hormone synthesis is a multistep process. Thyroid peroxidase generates reactive iodine species that can attack thyroglobulin. The first product is monoiodotyrosine (MIT). MIT is next iodinated on the carbon 5 position to form diiodotyrosine (DIT). Two DIT molecules then undergo an oxidative condensation to form T4 with the elimination of the alanine side chain from the molecule that forms the outer ring. There are two theories of how this coupling reaction occurs. One holds that the coupling occurs with both DIT molecules attached to thyroglobulin (intramolecular coupling). The other holds that the DIT that forms the outer ring is first detached from thyroglobulin (intermolecular coupling). In either case, thyroid peroxidase is involved in coupling as well as iodination. T3 is formed by condensation of MIT with DIT. A small amount of RT3 is also formed, probably by condensation of DIT with MIT. In the normal human thyroid, the average distribution of iodinated compounds is 3% MIT, 33% DIT, 35% T4, and 7% T3. Only traces of RT3 and other components are present.
The human thyroid secretes about 80 μg (103 nmol) of T4, 4 μg (7 nmol) of T3, and 2 μg (3.5 nmol) of RT3 per day (Figure 19–7). MIT and DIT are not secreted. These iodinated tyrosines are deiodinated by a microsomal iodotyrosine deiodinase. This represents a mechanism to recover iodine and bound tyrosines and recycle them for additional rounds of hormone synthesis. The iodine liberated by deiodination of MIT and DIT is reutilized in the gland and normally provides about twice as much iodide for hormone synthesis as NIS does. In patients with congenital absence of the iodotyrosine deiodinase, MIT and DIT appear in the urine and there are symptoms of iodine deficiency (see below). Iodinated thyronines are resistant to the activity of iodotyrosine deiodinase, thus allowing T4 and T3 to pass into the circulation.
FIGURE 19–7
Secretion and interconversion of thyroid hormones in normal adult humans. Figures are in micrograms per day. Note that most of the T3 and RT3 are formed from T4 deiodination in the tissues and only small amounts are secreted by the thyroid. T4 is also conjugated for subsequent excretion from the body.
TRANSPORT & METABOLISM OF THYROID HORMONES
The normal total plasma T4 level in adults is approximately 8 μg/dL (103 nmol/L), and the plasma T3 level is approximately 0.15 μg/dL (2.3 nmol/L). T4 and T3 are relatively lipophilic; thus, their free forms in plasma are in equilibrium with a much larger pool of protein-bound thyroid hormones in plasma and in tissues. Free thyroid hormones are added to the circulating pool by the thyroid. It is the free thyroid hormones in plasma that are physiologically active and that feed back to inhibit pituitary secretion of TSH (Figure 19–8). The function of protein-binding appears to be the maintenance of a large pool of hormone that can readily be mobilized as needed. In addition, at least for T3, hormone binding prevents excess uptake by the first cells encountered and promotes uniform tissue distribution. Total T4 and T3 can both be measured by radioimmunoassay. There are also direct assays that specifically measure only the free forms of the hormones. The latter are the more clinically relevant measures given that these are the active forms, and also due to both acquired and congenital variations in the concentrations of binding proteins between individuals.
The plasma proteins that bind thyroid hormones are albumin, a prealbumin called transthyretin (formerly called thyroxine-binding prealbumin), and a globulin known as thyroxine-binding globulin (TBG). Of the three proteins, albumin has the largest capacity to bind T4 (ie, it can bind the most T4 before becoming saturated) and TBG has the smallest capacity. However, the affinities of the proteins for T4 (ie, the avidity with which they bind T4 under physiologic conditions) are such that most of the circulating T4 is bound to TBG (Table 19–1), with over a third of the binding sites on the protein occupied. Smaller amounts of T4 are bound to transthyretin and albumin. The half-life of transthyretin is 2 days, that of TBG is 5 days, and that of albumin is 13 days.
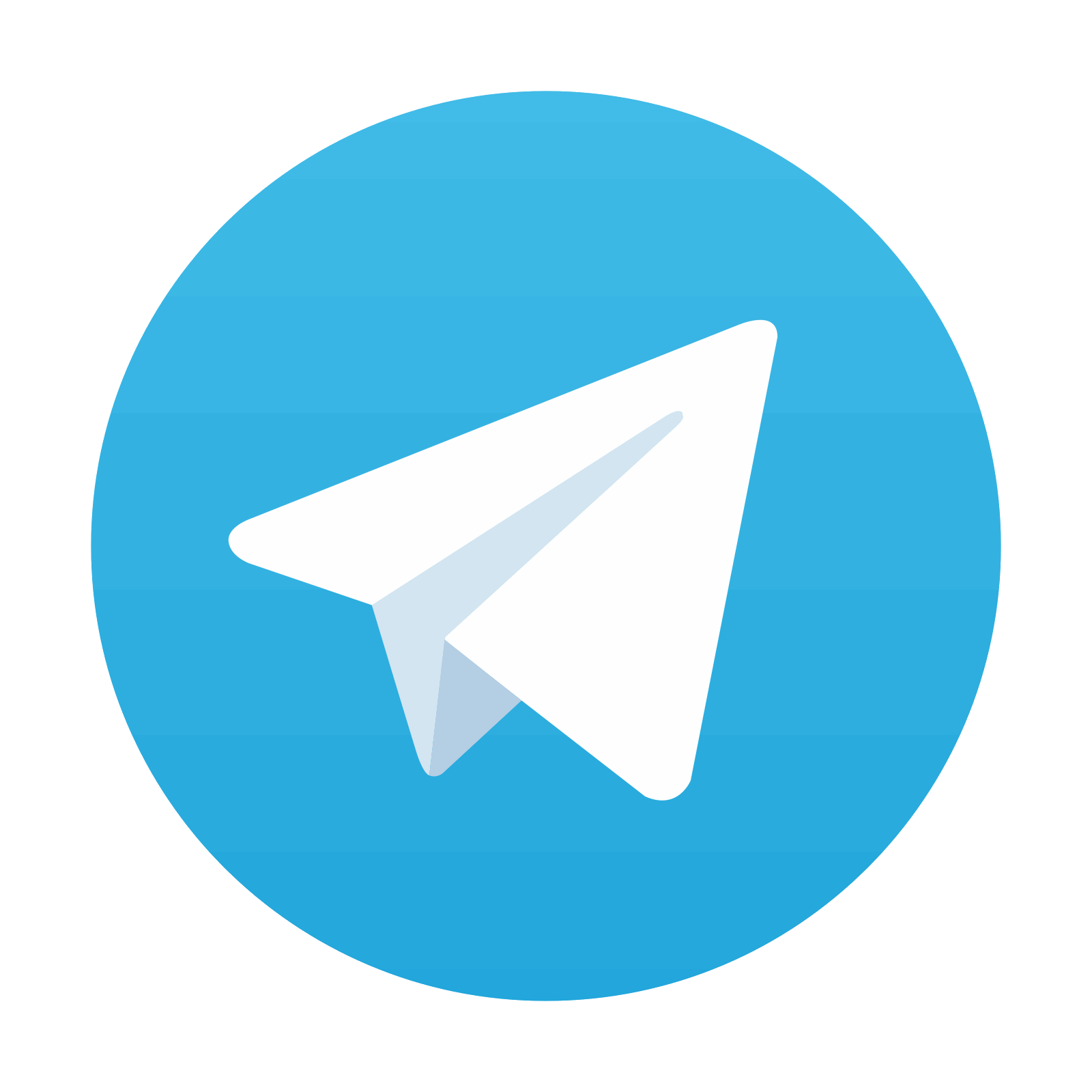
Stay updated, free articles. Join our Telegram channel

Full access? Get Clinical Tree
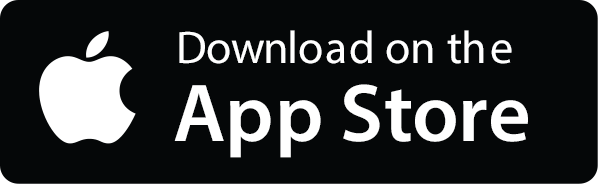
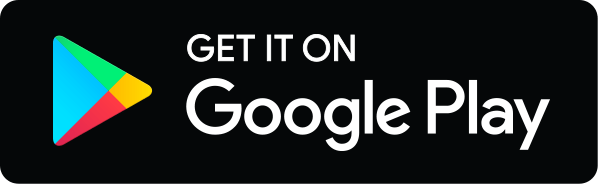
