Fig. 6.1
The function of seminal fluid in the female reproductive tract following coitus. Seminal fluid exposure at coitus induces female responses that have consequences for conception and pregnancy. In the uterus, seminal fluid mediated effects include the phagocytic clearance of superfluous sperm and microorganisms introduced at mating, sperm selection, preparation of a receptive endometrium, promotion of blastocyst development, and regulation of the maternal immune response towards paternal antigens. In addition to effects in the uterus, seminal fluid has also been demonstrated to have effects in the higher reproductive tract. In the oviduct, components of seminal fluid influence sperm storage as well as promote the development of the embryo through generation of embryotrophic cytokines. Further effects are seen in the ovary, where seminal fluid exposure contributes to the induction of ovulation and the promotion of corpus luteum formation, potentially via a countercurrent mechanism between the uterine vein and ovarian artery. Figure is updated from (O’Leary et al. 2002)
6.6 Clearance of Microorganisms and Superfluous Sperm
A major component of the female immune response to seminal fluid is the recruitment of neutrophils, which are prevalent in the uterine luminal cavity during the initial acute inflammatory phase following mating (De et al. 1991; Pandya and Cohen 1985). These neutrophils are believed to influence the capacity of sperm to reach the oocyte by removing superfluous sperm, microorganisms, and seminal debris (Robertson 2007). At coitus, commensal microorganisms from male or female tissues or sexually transmitted infections have the opportunity to enter the normally sterile higher reproductive tract where they can be detected in the immediate period post-coitus with sterility being recovered within 24 h (Robertson et al. 1999).
In addition to a function for neutrophils and other phagocytes in the rapid clearance of microorganisms introduced at coitus, there is also evidence that these cells may respond to seminal fluid exposure and act to phagocytose non-fertilising sperm. This process was originally postulated to act to filter out morphologically abnormal spermatozoa (Tomlinson et al. 1992). However, both abnormal and apparently viable and morphologically normal spermatozoa can undergo phagocytosis, suggesting that sperm selection may occur on the basis of morphological or antigenic parameters other than the ability to fertilise (Robertson 2005), and conferring female immune cells with the capacity to influence sperm selection through the recognition of surface markers that indicate faulty or damaged sperm (Sutovsky and Lovercamp 2010). The means by which phagocytes could discriminate between gametes remains to be determined, but if a biologically plausible mechanism were identified, this could explain observations of active female tract selection of male gametes (Robertson 2007).
6.7 Endometrial Receptivity and Embryo Implantation
The immune cells recruited into the endometrium in response to seminal fluid contribute to restructuring the endometrial environment to facilitate embryo implantation and support the first phase of placental development (Robertson 2005). Of the leukocytes present in the female reproductive tract following coitus, macrophages are postulated to play a critical role in the tissue remodelling process through the regulation of angiogenesis. Macrophages are a potent source of vascular endothelial growth factor (VEGF) and other key angiogenic factors and vascular permeability agents (Yoshida et al. 1997). The expression of these angiogenic factors is tightly regulated through the estrous cycle and early pregnancy (Ma et al. 2001) with a reduction of VEGF mRNA expression in hamsters after coitus occurring when seminal fluid signalling is perturbed by mating with accessory gland-deficient males (Chow et al. 2003). Evidence of a role for seminal fluid in angiogenesis is also demonstrated by studies in the horse and pig which show an increase in uterine vasodilation and oedema in the days following coitus (O’Leary et al. 2004; Bollwein et al. 2003).
Macrophage secreted products may also target the extracellular matrix of the endometrial stroma, which is remodelled during the decidualisation period (Aplin 2002). In particular, macrophage-secreted matrix metalloproteinases (MMPs) and tissue inhibitors of MMPs (TIMPs) coordinate their expression to contribute to this remodelling process (Robertson 2005). In rodents, MMPs and TIMPs are induced during the pre-implantation period (Das et al. 1997; Feng et al. 1998), with seminal plasma postulated to contribute to their induction as the absence of accessory gland fluid at coitus in the hamster is associated with a reduction in MMP2 at the implantation site (Chow et al. 2003).
In addition to macrophages, seminal fluid-induced dendritic cells may contribute to tissue remodelling in preparation for implantation. Uterine dendritic cells have been demonstrated to be crucial in decidua formation and maternal receptivity. Depletion of uterine dendritic cells around the period of implantation leads to impaired decidual formation through perturbed angiogenesis. This process was postulated to be regulated by dendritic cell soluble fms-like tyrosine kinase-1 (sFlt1) and transforming growth factor beta (TGFB) which promote coordinated blood vessel maturation (Plaks et al. 2008).
Embryo attachment and implantation is a process requiring specific changes in the expression of integrins and mucins which function to allow close apposition and then adhesion between the blastocyst and implantation site (Aplin 1997). Fucosyltransferases, which modulate the expression of fucosylated structures involved in the attachment and implantation process, are deficient when seminal plasma signalling is perturbed by surgical removal of the seminal vesicle from males (Jasper et al. 2011), suggesting that seminal plasma exposure contributes to this process.
Macrophages are implicated as cellular mediators transmitting the effects of seminal fluid on endometrial receptivity. They are postulated to secrete molecules that target luminal epithelial cells to induce modifications to proteins involved in embryo attachment (Robertson 2005). In support of this, mouse macrophage-derived LIF and IL1B induce fucosyltransferases Fut2 and Fut4 required for embryo attachment and implantation (Jasper et al. 2011), and activity of fucosyltransferases is impaired when females are mated with seminal fluid-deficient males (Jasper et al. 2011). Studies in human tissues indicate a similar mechanism, where leukocytes influence the adhesive properties of uterine epithelial cells in vitro (Kosaka et al. 2003). In the cow, intrauterine administration of leukocytes may operate through a similar pathway to increase embryo implantation rates (Ideta et al. 2010).
6.8 Synthesis of Embryotrophic Cytokines
A key role of the female tract response to seminal fluid is the synthesis of embryotrophic cytokines that control the development of the pre-implantation embryo. Alterations to embryo programming may have a major influence on long-term offspring health as small perturbations in blastomere number and inner cell mass/trophectoderm allocation in the blastocyst are associated with an altered growth trajectory of the fetus and resulting offspring (Thompson et al. 2002). Embryos express cytokine receptors from conception until implantation with seminal fluid-induced cytokines GMCSF, IL6, LIF, and MCSF all exerting direct effects on blastocyst cell number and viability, gene expression, and developmental competence (Robertson 2005). Prominent cytokine receptor pathways demonstrated to be involved in pre-implantation embryo development include the phosphatidylinositol-3 kinase-protein kinase B (PI3K-AKT), Janus kinase/signal transducer and activator of transcription (JAK/STAT), and mitogen-activated protein kinase (MAPK) pathways. Depending on the cytokine, ligation may affect one or more of these signal transduction pathways to modify gene expression, metabolism, and differentiation, alter the epigenome, suppress the cell stress response, and ultimately impact blastomere survival (Robertson et al. 2011). Cytokine effects have profound impact on embryo developmental competence and programming future developmental trajectory, providing a pathway whereby the peri-conception environment affects the future growth of the fetus and phenotype of offspring after birth (Robertson et al. 2011; Sjoblom et al. 2005).
GMCSF, CSF1, IL6, and LIF are well-described examples of key embryotrophic cytokines induced in the uterus and oviduct by seminal fluid contact (Bromfield et al. 2014). In mice, GMCSF targets the pre-implantation embryo to promote blastocyst formation and increase the number of viable blastomeres by inhibiting apoptosis and facilitating glucose uptake (Robertson et al. 2001) and GMCSF deficiency compromises blastocyst development by inducing stress response and apoptotic gene pathways (Robertson et al. 2001; Chin et al. 2009). In cattle embryos, GMCSF regulates genes involved in de novo methylation to influence epigenetic reprogramming (Loureiro et al. 2009). IL6 regulates STAT3-dependent miRNAs that function to protect embryos from apoptosis (Shen et al. 2009) while neutralisation of LIF affects embryo implantation rates and fetoplacental development (Mitchell et al. 2002). Addition of CSF1 to embryo culture media improves embryo development (Pampfer et al. 1991) and reduced fertility in CSF1-deficient mice may be due to adverse effects in blastocysts (Cohen et al. 1997a). The contribution of seminal plasma to embryo development is illustrated by findings that coitus with seminal plasma-deficient males is associated with reduced cytokine synthesis and this is linked with a significant reduction in the rate of cleavage to the 2-cell stage in zygotes and reduction in the development of blastocysts from 2-cell embryos (Bromfield et al. 2014).
In contrast to cytokines and growth factors that exert a positive influence on embryonic development, factors that have profound inhibitory effects on embryo development have also been identified. Increased levels of apoptosis-inducing cytokines TNF, interferon gamma (IFNG), and TNF-related apoptosis-inducing ligand (TRAIL) in the female reproductive tract following coitus are emerging as factors that cause pre-implantation embryonic loss or impair embryo development. TNF acts in vitro to increase the percentage of apoptotic blastomeres in the mouse (Fabian et al. 2007), while IFNG inhibits the embryotrophic factor GMCSF and also exerts direct inhibitory effects in mouse embryo culture (Robertson et al. 1992; Haimovici et al. 1988). TRAIL induces apoptosis in the pre-implantation embryo (Riley et al. 2004). Like the embryotrophic cytokines, these cytokines are regulated by seminal fluid and particularly oviduct TRAIL expression is suppressed in response to seminal fluid factors (Bromfield et al. 2014).
In the human, the embryotrophic cytokines GMCSF, IL6, and LIF are induced in cervical and endometrial epithelial cells in response to seminal plasma exposure (Sharkey et al. 2012a, b; Gutsche et al. 2003), but to date whether this occurs in the higher reproductive tract in vivo has not been confirmed. These cytokines are predicted to have a similar role in the human as in vitro culture of human embryos in GMCSF improves developmental outcomes through an increase in embryos reaching blastocyst stage, with earlier blastulation and increased inner cell mass and trophectoderm cell number (Sjoblom et al. 1999), followed by improved implantation rates and progression to healthy birth after IVF treatment (Ziebe et al. 2013). Due to the challenges in accessing appropriate tissues, it has not been possible to ascertain the extent to which expression of embryotrophic and embryotoxic cytokines responds to coital activity to influence human embryo development and implantation after natural conception.
The capacity for seminal fluid to alter the balance of embryotrophic and embryotoxic cytokines provides a mechanism through which the female reproductive tract can reflect signals from the male, to either support or impair progression of pre-implantation embryo development, and impart longer-term programming. The capacity to liberate embryotoxic cytokines such as TRAIL in the absence of sufficient seminal fluid suppression raises the prospect of maternal tract ‘quality control’. If proven to occur, this would be relevant in couples where altered seminal fluid composition provides insufficient signalling factors (Sharkey et al. 2007) or where sexually transmitted infection alters the balance of seminal fluid signals to induce a proinflammatory phenotype (Rasmussen et al. 1997). These changes may affect progression of these embryos as well as imparting effects on offspring phenotype and health in adult life (Bromfield et al. 2014; Sjoblom et al. 2005).
6.9 Maternal Immune Tolerance
A critical component of the female tract response to seminal fluid which is essential for ongoing pregnancy success is programming of the maternal immune system to respond to the presence of the genetically disparate fetus. Immune tolerance must exist from the very earliest time the embryo contacts the maternal tissues at implantation. A combination of strategies contributes to the activation of maternal immune tolerance, with seminal fluid providing antigens and cytokines that, in the correct immune environment, drive production of regulatory T cells (Trowsdale and Betz 2006; Robertson et al. 2009a).
Seminal fluid contains several antigens specific to individual males including class Ia, Ib, and II major histocompatibility complex (MHC) (Hutter and Dohr 1998) that can be presented by antigen-presenting cells (APCs) such as macrophages and dendritic cells recruited into the endometrium following exposure to seminal fluid (Robertson et al. 1996; McMaster et al. 1992). These dendritic cells and macrophages in the presence of seminal fluid factors prostaglandin E (PGE) and TGFB differentiate into cells that mediate tolerogenic immune responses (Blois et al. 2007; Jaiswal et al. 2012). However, not all APCs are tolerogenic as the immune environment at this stage of pregnancy is highly varied with both tolerogenic and immunogenic cells present, demonstrating differing roles for these cells during peri-conception (Jaiswal et al. 2012). The APCs are thought to take up paternal antigens and either traffic to the uterine draining lymph nodes or interact locally with resident uterine T cells driving activation and expansion of clonal subsets of Treg cells which recognise and appropriately respond to paternal/fetal antigens (Robertson et al. 2009a). An antigen-specific T cell response has been demonstrated using a T cell receptor transgenic model with OVA as a model paternal antigen (Moldenhauer et al. 2009). The concept of seminal fluid-induced tolerance of paternal antigens is supported by experiments demonstrating prolonged survival of skin grafts of paternal origin in mated female mice (Lengerova and Vojtiskova 1966) and studies demonstrating tolerance towards tumor cells expressing the same MHC as the donor male (Robertson et al. 2009b).
Treg cells operate as potent suppressors of inflammation and cell-mediated immunity (Rudensky 2011). These cells act via a variety of mechanisms, generally involving the suppression of cytokine production and effector function in T cells, B cells, natural killer (NK) cells, dendritic cells, and macrophages (Sakaguchi 2000; Shevach 2002). In pregnancy, Treg cells play a critical role in mediating immune tolerance required for embryo implantation, with several studies demonstrating in the mouse that allogenic mating leads to fetal rejection unless sufficient Treg cells are present in the endometrium in the pre- and peri-implantation phase (Aluvihare et al. 2004). In humans, Treg cells play a similar regulatory role, with an increase in pregnant women of circulating CD4+ CD25+ cells highly enriched for the signature Treg transcription factor forkhead box P3 (FOXP3) in early pregnancy. The number of these regulatory cells peaks during the second trimester before declining at term (Somerset et al. 2004).
The presence of TGFB and PGE in seminal fluid confers immune-deviating activity that drives immune cells into tolerogenic phenotypes. Both factors have been linked with the induction of naïve T cells into suppressor T cells expressing Foxp3 (Chen et al. 2003; Baratelli et al. 2005) and exogenous TGFB delivery at conception is shown to boost vaginal Treg cell numbers which acts to reduce fetal loss in the abortion prone CBA/J x DBA/2 J model (Clark et al. 2008). Because of poor availability of suitable reagents for Treg cells, they have not been extensively studied in other species, other than in the cat where there is evidence that an increase in Treg cells in early pregnancy occurs after seminal fluid exposure (Lockett et al. 2010).
6.10 Ovulation and Corpus Luteum Formation
As well as the endometrium, seminal fluid exposure at coitus has effects in the ovary. This effect was first described in camelids, where effects of seminal plasma on induction of ovulation are well known (Li and Zhao 2004). It is challenging to conceive how seminal fluid moieties could reach the ovary via the oviduct. An alternative pathway for seminal fluid effects on ovarian function may be due to a unique countercurrent exchange mechanism through which prostaglandins and other small molecules can be transmitted from the uterine vein to the ovarian artery (Krzymowski et al. 1989). In the presence of a mature follicle, ovulation can be induced by natural coitus, artificial insemination, or intramuscular injection of seminal plasma, but not washed sperm. The ovulation-inducing factor in camelid seminal plasma has been identified as the highly conserved beta-nerve growth factor, which acts via an endocrine mechanism targeting the hypothalamic–pituitary axis to induce LH release and ovulation (Ratto et al. 2012). This so-called ovulation-inducing factor (OIF) is present in other species demonstrating induced ovulation (koalas and rabbits). Recent studies indicate that OIF in seminal plasma is conserved among species, including cattle, horses, pigs, mice, and other species considered to be spontaneous ovulators (Bogle et al. 2011; Ratto et al. 2006). Additional studies showing that OIF can induce ovulation in prepubertal mice (Bogle et al. 2011) and alter ovarian follicular wave dynamics in cows (Tanco et al. 2012) imply this factor may have broader relevance than originally thought (Ratto et al. 2012). Seminal plasma-mediated effects on ovarian function are clearly demonstrated in pigs, where a reduction in the interval between LH surge and ovulation, followed by elevated plasma progesterone concentration over the pre-implantation phase, occurs when seminal plasma is instilled into the uterus just prior to ovulation (Waberski et al. 1997; O’Leary et al. 2001).
As well as endocrine actions, the effects of seminal fluid on ovarian function in rodents and pigs may be mediated through local mechanisms involving stimulation of macrophage populations. Ovarian macrophages contribute to regulation of follicle development, ovulation, and post-ovulatory tissue remodelling associated with the conversion of the ruptured follicle into the corpus luteum (Cohen et al. 1997b; Care et al. 2013). Ovarian macrophages are present in the theca of developing follicles and their numbers increase at ovulation (Brannstrom et al. 1993). These macrophages support follicle growth, ovulation, and vascular homeostasis (Brannstrom et al. 1993; Wu et al. 2004; Van der Hoek et al. 2000; Turner et al. 2011). In both rodents and humans, macrophages migrate into the developing corpora lutea immediately after ovulation (Cohen et al. 1997b; Hellberg et al. 1991; Brannstrom et al. 1994a, b) where they influence steroidogenic function of luteal cells as well as tissue remodelling after luteal regression (Kirsch et al. 1981; Nelson et al. 1992).
In mice, seminal plasma influences macrophage recruitment into the ovary, as mating with seminal plasma-deficient males results in fewer macrophages in the corpus luteum compared to mating with seminal plasma sufficient males (Gangnuss et al. 2004). Cytokines that are induced by seminal fluid in the uterus such as MCP1, MCSF, and GMCSF (Robertson 2005) also act as chemoattractants to recruit macrophages into the ovary (Cohen et al. 1997b; Jasper et al. 2000; Townson et al. 1996), although whether these cytokines are induced in the ovary by seminal fluid remains to be examined. Serum progesterone levels on days 1, 2, and 4 of pregnancy in mice are not different with seminal plasma deficiency (Gangnuss et al. 2004). However, a threshold level of macrophage activity is essential for normal corpus luteum development as macrophage depletion disrupts the luteal microvascular network and alters expression of VEGFs required to support luteal angiogenesis in the peri-implantation period (Care et al. 2013). The result is increased inflammatory and apoptotic gene expression, decreased steroidogenic gene expression, and infertility which can be rescued by exogenous progesterone administration (Care et al. 2013).
6.11 Active Factors in Seminal Fluid
To further understand the function of seminal fluid at coitus, studies have aimed to identify the components of the ejaculate that induce the female tract response. Early studies in the mouse demonstrated through removal of the male accessory glands that the seminal plasma fraction contains many of the active factors, as its absence resulted in a reduced production of GMCSF and reduced leukocyte infiltration in the endometrium (Robertson et al. 1996). Further analysis of the high molecular weight component of mouse seminal fluid that induced these changes was able to identify TGFB as the principal trigger (Tremellen et al. 1998). These findings have since been confirmed in the human, where high levels of TGFB in seminal plasma contribute to the peri-conception inflammatory response by inducing GMCSF, IL1B, IL6, and LIF in human cervical epithelial and endometrial epithelial cells in vitro (Sharkey et al. 2012a; Gutsche et al. 2003). Secreted TGFB is initially produced in a precursor dimeric form where it is activated in the female tract after insemination as a consequence of the low pH, enzymatic activation with plasmin or interaction of the latent TGFB with thrombospondin-1 or avb6 integrins (Robertson 2005). In the mouse there is approximately 30 ng/ml TGFB1 in the ejaculate (Robertson et al. 2002) while in the human the TGFB concentrations are 219 ng/ml TGFB1, 5 ng/ml TGFB2, and 172 ng/ml TGFB3 (Sharkey et al. 2012a). TGFB is also detected in the seminal plasma of pigs (O’Leary et al. 2011) and sheep (Scott et al. 2006), with the pig carrying similar concentrations [150 ng/ml TGFB1 (O’Leary et al. 2011)] to that of the human and mouse. There is no correlation between TGFB and fertility (Loras et al. 1999), but studies in the seminal plasma of fertile men show that there are substantial differences in TGFB concentrations between individuals (Sharkey et al. 2012a).
In addition to TGFB, we have recently identified that ligands for TLR4 contribute to the peri-conception inflammatory response in the mouse. In these studies, the induction of colony-stimulating factor 3 (Csf3), Cxcl1, and Cxcl2 was shown to require the presence of TLR4 while the TLR4 signalling pathway may also contribute to the induction of Il1a, Tnf, and Csf2 (Schjenken et al. 2015). While it has been speculated that bacterial products including endotoxin may contribute to the capacity of seminal fluid to interact with the female reproductive tract (Schaefer et al. 2004), the amount of endotoxin detectable in the female reproductive tract of mice following coitus (Schjenken et al. 2015) and in human seminal fluid (Sharkey, in preparation) is insufficient to explain the induction of TLR4 regulated cytokines. This suggests that other TLR4 ligands such as the endogenous danger-associated molecular patterns (DAMPs) contribute to eliciting the female response; however, these studies are yet to be completed. DAMPS have previously been identified in seminal fluid with beta-defensin-2 (Narciandi et al. 2014), fibronectin (Lilja et al. 1987), heat shock proteins (Rego et al. 2014; Pilch and Mann 2006), hyaluronidase (Geipel et al. 1992; Shimada et al. 2008), and S100 proteins (Rego et al. 2014; Donato et al. 2013) all being present.
In line with the functions of seminal fluid, activation of TLR4 signalling may contribute to tolerogenic immune responses (Conroy et al. 2008), as studies have demonstrated that CD45RBlow CD25+ regulatory T cells can express TLR4 and enhance suppressive function following TLR4 ligation (Caramalho et al. 2003), dendritic cells from TLR4-deficient mice have reduced capacity to produce IL10 in response to TLR4 ligation and have impaired expansion of Treg cells (Higgins et al. 2003), and Treg cells cultured in the presence of dendritic cells can induce tryptophan catabolism which enhances tolerogenic dendritic cell production (Fallarino et al. 2015). More detailed studies are required to understand how disruption of TLR4 signalling may affect the female response to seminal fluid in the mouse and other species and how in turn offspring health might be affected.
In humans, PGE in seminal fluid are postulated to act as signalling agents, most notably those in the 19-hydroxy (19-OH) form (Templeton et al. 1978). While PGE is detectable in equine seminal plasma (Claus et al. 1992), it is undetectable in rodent and porcine samples. In the human, extremely high levels of PGE are detected in seminal fluid, with 300 μM of the 19-OH form detectable (Templeton et al. 1978). Due to the immunosuppressive nature of 19-OH PGE, it was postulated that seminal prostaglandins may protect sperm from immunological damage in the male and protect the female against sensitisation to sperm antigens (James and Hargreave 1984; Alexander and Anderson 1987). In vitro experiments using human cervical explants stimulated with 19-OH PGE demonstrated an induction of IL8 and suppression of the anti-inflammatory molecule, secretory leukocyte protease inhibitor (Denison et al. 1999). Seminal plasma PGE2 has also been linked to facilitate tumorigenesis-angiogenesis in reproductive tract cells through activation of fibroblast growth factor 2, COX2, and VEGF expression and E series prostanoid-2 and -4 receptor, EGF receptor, and ERK1/2 signalling pathways (Battersby et al. 2007; Muller et al. 2006). Further, PGE has been demonstrated in the human to induce a regulatory phenotype in naïve CD4+CD25− T cells, enhance the in vitro inhibitory function of Treg cells, and induce expression of Foxp3 (Baratelli et al. 2005) which is consistent with the female tract response to seminal fluid.
Other factors postulated to contribute to seminal fluid signalling include IL8, which is present in seminal plasma of healthy human donors at 1456 pg/ml. Treatment of human endometrial epithelial cells in vitro with recombinant IL8 at physiological levels can induce IL1B, IL6, and LIF (Gutsche et al. 2003). Outside of mice and humans, other novel seminal fluid signalling molecules that have been examined are porcine sperm adhesion proteins (PSP)1 and PSP2, which contribute in part to the influx of neutrophils in pigs and can preserve sperm viability, motility, and mitochondrial activity (Rodriguez-Martinez et al. 2010; Caballero et al. 2006), and cysteine-rich secretory protein-3 (CRISP3) in horses, which mediates the interaction between sperm and neutrophils (Doty et al. 2011). Positive correlations between specific seminal plasma proteins and fertility have been observed in the horse (Brandon et al. 1999) and bull (Killian et al. 1993).
Despite the evidence showing an integral role for seminal plasma in the signalling process, it is becoming apparent that not all seminal fluid effects can be accounted for by the soluble fraction, raising the prospect that sperm also communicate with female reproductive tract cells. In domestic animal species, there is extensive evidence that sperm is critical to the inflammatory response and may be required to interact with seminal plasma to regulate the influx and function of immune cells (Schjenken and Robertson 2014). In the human and mouse, while the literature has focused on seminal plasma, there is some evidence for a sperm signalling component. In the mouse, sperm has been shown to contribute to the peri-conception immune environment as Foxp3 expression requires the presence of both sperm and seminal plasma for its complete induction (Guerin et al. 2011) while in the human, AI of human sperm into the cervix results in an influx of leukocytes (Pandya and Cohen 1985; Thompson et al. 1992). A function for sperm in the signalling process is further supported by studies in the mouse showing that sperm can form intimate associations with cells of the female reproductive tract following coitus (Reid 1965). Non-fertilising sperm can be taken up by female tissues, potentially by phagocytosis, where sperm-associated transcripts can be identified in the uterus and other distal sites, including the uterine draining lymph nodes for several days post-coitus (Watson et al. 1983). Similar interactions between sperm and the female reproductive tract can be seen in other species, including human, pigs, cow, dog, cats, bats, lizards, and even some marsupials (Suarez and Pacey 2006; Murakami et al. 1985; Rasweiler 1987; Nogueira et al. 2011; Pacey et al. 1995; Taylor et al. 2008; Rijsselaere et al. 2004; Ignotz et al. 2001; Breed et al. 1989). Seminal plasma proteins contribute to these interactions as bovine seminal plasma (BSP) proteins coat bull sperm and mediate interactions between sperm and oviductal epithelial cells to assist in the formation of sperm storage reservoirs, which function to maintain fertility of sperm until ovulation (Suarez 2008). In addition to the formation of storage reservoirs, these interactions assist in the clearance of sperm at coitus. Additionally, these interactions may potentially be a mechanism by which sperm communicates with the female reproductive tract to transmit antigen and facilitate pregnancy success; however, this remains to be determined.
In contrast to signalling molecules that promote successful pregnancy, there is also evidence that seminal fluid may contain factors that inhibit seminal fluid signalling. The type 1 cytokine IFNG can be detected in the seminal fluid of both mouse (~50 pg/ml) (Gopichandran et al. 2006) and human (range: 0–130 pg/ml) (Politch et al. 2007) and in the mouse has been shown to inhibit the tolerance inducing properties of TGFB during early pregnancy (Glynn et al. 2004). Whether IFNG levels are altered in the seminal fluid of infertile or subfertile men remains to be determined.
While several active factors that contribute to seminal fluid signalling have been identified, future studies will be required to understand in more detail how these factors interact to regulate the female response. In addition, if sperm contain signalling factors as has been postulated, studies will be required to elucidate these factors and their function. Additional factors beyond those discussed here no doubt exist. Exciting candidates include CD52, transglutaminase 4, and seminal vesicle secretion 2. CD52 is present in soluble form in seminal plasma and on the sperm surface (Koyama et al. 2009), which is recently reported to be a potent immune-regulatory signal driving induction of antigen-activated suppressor T cells, distinct from FOXP3+ Treg cells, from CD4 precursors (Bandala-Sanchez et al. 2013). Transglutaminase 4 and seminal vesicle secretion 2 are proteins which are known to play a critical role in copulatory plug formation (Dean 2013; Kawano et al. 2014). Interestingly, the absence of these seminal proteins disrupts not only plug formation but also fertility. Sperm from ejaculates lacking transglutaminase 4 fertilised at normal rates but were significantly less likely to give birth to a litter (Dean 2013). Sperm from seminal vesicle secretion 2-deficient mice are disrupted by uterine-derived cytotoxic factors (Kawano et al. 2014), suggesting that seminal plasma plays a protective role in sperm function.
Consideration of species-specific differences is integral as it is highly likely, due to the different sites of semen deposition, that different species will utilise different active factors. Within a species, fluctuations in the seminal fluid profile between individuals, or even within an individual, may influence the female response leading to altered outcomes for offspring depending on male health and environmental exposures.
6.12 Seminal Fluid and Programming of Offspring Health
The studies detailed above document a complex role for seminal fluid in preparing the female reproductive tract for pregnancy, particularly through regulating the maternal immune response to tolerate exposure to paternal antigens. These changes not only influence the period of early pregnancy but also have the potential to influence fetal development and offspring health. It is well documented that the in utero environment is a major determinant in programming how adult individuals respond to stressors and challenge, and the risk of disease later in life (Godfrey et al. 2010). Increasingly the peri-conception period is identified as the most sensitive for imprinting later life consequences, and the health and experiences of both the mother and father even prior to conception contribute through effects transmitted by the male and female gametes (Lane et al. 2014). There is evidence that altered conditions at conception can induce adaptations to protect the fetus from immediate effects, but a consequence of these changes is an increased risk of later metabolic disease (Barker and Clark 1997; Hanson and Gluckman 2005). Studies on the paternal contribution towards health and disease have identified smoking, age, environmental exposures, and obesity being linked to later offspring illness (Fullston et al. 2012), with sperm transmission of epigenetic mechanisms including non-coding RNAs (Fullston et al. 2012; Liu et al. 2012) being postulated to contribute to these changes.
As well as a contribution from sperm, there is the prospect that seminal fluid can contribute at fertilisation to influence the peri-conception environment in turn affecting later development and offspring health (Lane et al. 2014). Some evidence supporting a role for seminal plasma through its influence over the female reproductive tract at coitus is emerging. In mice, Bromfield et al. (Bromfield et al. 2014) demonstrate that conception in the absence of seminal plasma using mice surgically rendered seminal vesicle deficient (SVX) leads to a reduction in fecundity and altered fetal and neonatal outcomes. Mice mated with SVX males had a significant reduction in progression to pregnancy and fewer implanting embryos compared to intact mated females. Changes in development were observed as early as the eight-cell stage, where most embryos from SVX fathers were developmentally delayed. Prior to birth, changes in phenotype were evident as placental hypertrophy. Male offspring were observed to have altered growth trajectory and metabolic parameters with evidence of obesity, distorted metabolic hormones, reduced glucose tolerance, and hypertension. Embryo transfer experiments confirmed the role of seminal plasma in programming altered phenotype as increased adiposity was also observed in adult male progeny when normal 2-cell embryos were transferred to females mated with SVX males (Bromfield et al. 2014).
Similar studies have been conducted in the golden hamster, where ablation of the accessory sex glands (ASG), which produce seminal plasma, delayed entry of the zygote into the first cell cycle (Ying and Chow 1998), reduced pre-implantation embryonic cell number (Chan et al. 2001), resulted in an early transit of the embryo into the uterus from the oviduct (Wong et al. 2008), decreased the rate of implantation, and ultimately resulted in embryo death (Jiang et al. 2001). The offspring of fathers deficient in seminal plasma exhibited altered postnatal growth and elevated anxiety (Wong et al. 2007). It is postulated that these changes may be associated with epigenetic mechanisms as cleavage stage embryos from females mated with ASG-deficient males showed reduced acetylation and altered methylation kinetics which were associated with dysregulated expression of the paternally expressed Igf2 and Dlk1 (Jiang et al. 2001).
In the human, there is no clear direct evidence implicating altered seminal fluid signalling activity with long-term health of offspring. However, there are clinical observations that are consistent with a similar function (discussed below). This means that alterations to the content of seminal fluid outside of the normal range may not manifest as impaired fertility, but could potentially alter fetal development and impart long-term consequences for offspring health. Carefully designed studies will be required to evaluate this, as effects on offspring can be subtle and require large populations to detect. Additionally, it will be important to distinguish effects of seminal fluid factors exerted via the female reproductive tract from those exerted through effects on sperm fertilising capacity and epigenetic status.
6.13 Clinical Implications of Altered Seminal Fluid Signalling
These considerations raise the question of the extent to which seminal fluid exposure may influence pregnancy outcomes in humans. The success of IVF even in women without male partners demonstrates that seminal plasma is not an absolute requirement for pregnancy. Rather, evidence suggests that rather than being an absolute prerequisite, seminal plasma influences the success and quality of pregnancy outcome (Robertson 2005). There are few definitive studies in humans focussed on evaluating effects of seminal fluid, but clinical studies point to a consistent beneficial effect in improving the chance of conception and protecting from immune-mediated gestational disorders such as preeclampsia.
Preeclampsia is characterised by a significant reduction in peripheral blood CD4+CD25high T regulatory cells and decidual Foxp3-positive cells within the CD3+ population (Sasaki et al. 2007). The altered Treg cell numbers may in part be due to altered seminal fluid function, with extensive evidence pointing to an important role for TGFB in regulating human Treg cell reactivity and autoimmunity (Rubtsov and Rudensky 2007). Clinical studies show that preeclampsia is more common in situations where limited exposure to the conceiving partners seminal fluid has occurred—including first conception, after a short period of cohabitation, when barrier contraception has been used, or when multiparous mothers conceive with a new male partner [reviewed in Schjenken and Robertson (2014)]. Treg cell numbers and function are found to be altered in the peripheral blood and decidua of pregnant women who have spontaneous abortions compared to induced abortions (Sasaki et al. 2004; Arruvito et al. 2007), while FOXP3 expression, used as a surrogate measure of Treg cells, is reduced in the endometrium of women with primary unexplained infertility (Jasper et al. 2006).
Data from ART further support a protective function for seminal fluid. The absence of seminal plasma in the in vivo setting has been associated with higher rates of implantation failure and decreases in embryo quality [reviewed in Schjenken and Robertson (2014)]. Exposure to semen around the time of embryo transfer is demonstrated to improve embryo viability 6–8 weeks post-transfer (Tremellen et al. 2000). Given the emerging recognition that IVF is linked with reduced birth weights and impaired metabolic health outcomes for IVF children (Hart and Norman 2013), and the overlap between these changes and the phenotypes of offspring conceived without seminal fluid in mouse studies (Bromfield et al. 2014), it would seem prudent to more formally investigate the impact of seminal fluid on perinatal outcome and offspring health in human IVF. One difficulty with advising couples to have coitus at the time of embryo transfer is the small but real prospect of multiple pregnancy due to natural conception, but this would be expected to be a small risk in couples experiencing long-term infertility.
6.14 Conclusions
Seminal fluid signalling appears to play an integral role in eliciting inflammatory changes in the female reproductive tract following coitus in all species examined so far, despite differences in sites of semen deposition and reproductive physiology. This implies that the capacity for seminal fluid to exert effects in the female reproductive tract has biological value beyond the support of sperm. The enormous variation between mammalian species in seminal fluid volume, tissue origin and composition, and actions in the female implies rapid evolution that is driven by sexual selection, analogous to that which occurs in invertebrate species. For sexual selection to occur there must be variation in the female response that impacts the likelihood of progression to pregnancy. The array of effects induced by seminal fluid that impact conception, embryo development, implantation, and fetal growth together impact fertility and fecundity and ultimately influence offspring survival and health. Signalling factors within seminal fluid, in particular in the seminal plasma fraction, have been identified, but there are clearly other, as yet unknown factors that contribute to signalling in the female tract, potentially including sperm.
Despite the advances that have been made in understanding the contribution of seminal fluid, there is still much to learn. In particular, the contribution of seminal fluid to offspring phenotype requires further study and the knowledge to be gained will have major implications in human and animal ART, where seminal fluid signalling is often overlooked or avoided. In humans there are substantial variations in seminal fluid profiles, but the implications and causes of this are not known. Whether these variations, or the presence of conditions such as reproductive tract infection, affect the capacity of seminal fluid to induce the appropriate response and ultimately affect male fertility, pregnancy health, or even offspring phenotype remains to be understood. Ultimately, a better understanding of seminal fluid signalling may improve success rates and outcomes of human IVF, and yield novel therapies for infertility and pathologies of pregnancy.
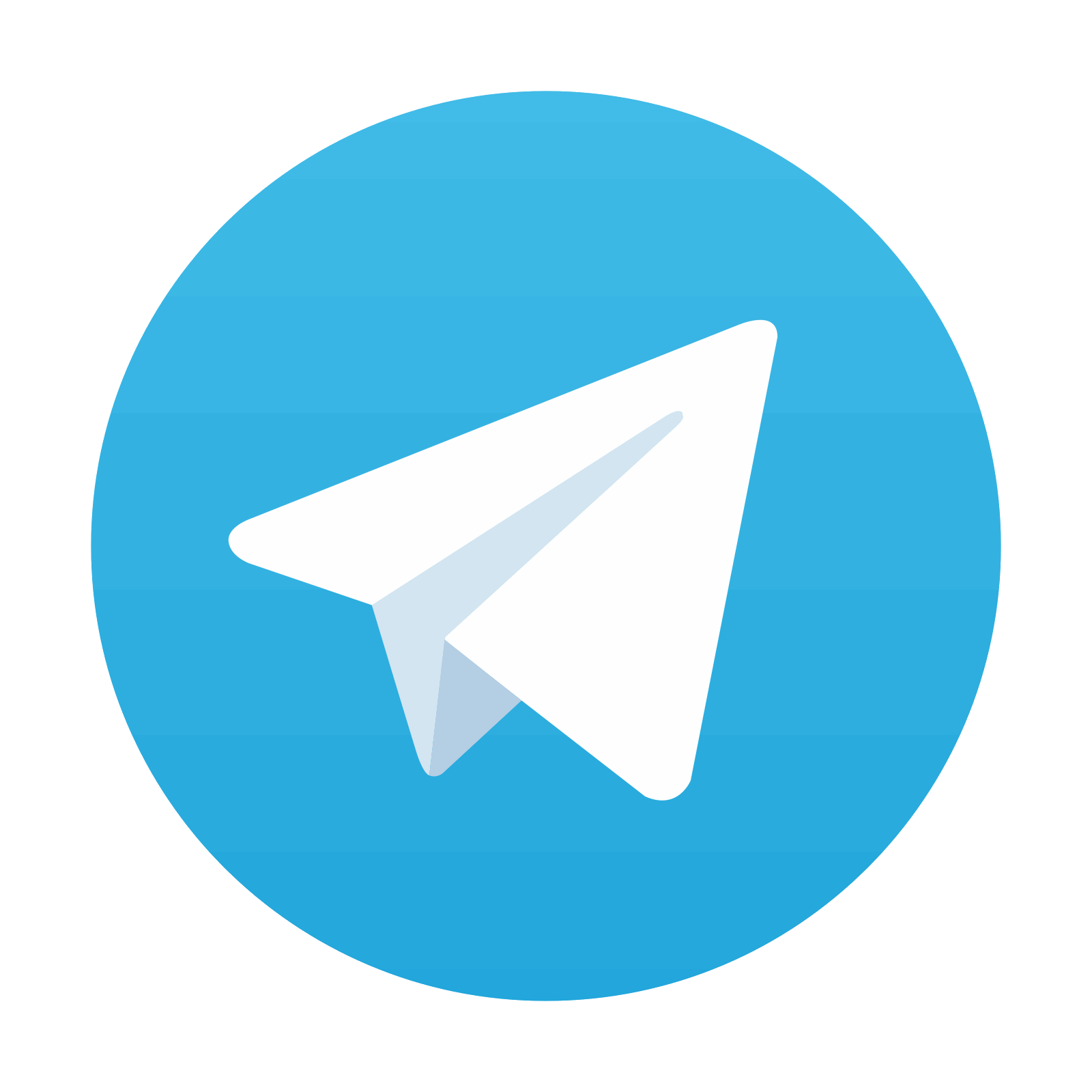
Stay updated, free articles. Join our Telegram channel

Full access? Get Clinical Tree
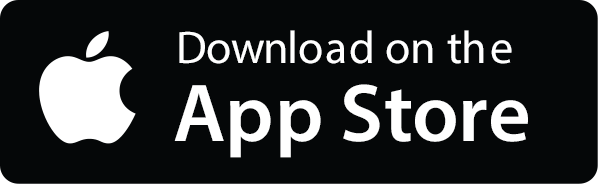
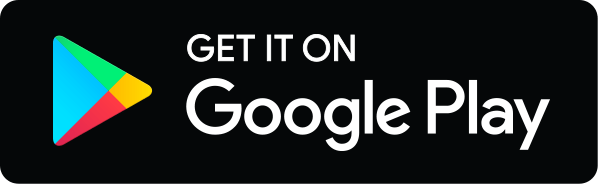