The kidneys are vital organs that perform a variety of important functions (Table 27.1). The most prominent functions are removal of unwanted substances from plasma (both waste and surplus); homeostasis (maintenance of equilibrium) of the body’s water, electrolyte, and acid–base status; and participation in hormonal regulation. In the clinical laboratory, kidney function tests are used in the assessment of renal disease, water balance, and acid–base disorders and in situations of trauma, head injury, surgery, and infectious disease. This chapter focuses on renal anatomy and physiology and the analytic procedures available to diagnose, monitor, and treat kidney dysfunction.
TABLE 27.1 Kidney Functions
RENAL ANATOMY
The kidneys are paired, bean-shaped organs located retroperitoneally on either side of the spinal column. Macroscopically, a fibrous capsule of connective tissue encloses each kidney. When dissected longitudinally, two regions can be clearly discerned—an outer region called the cortex and an inner region called the medulla (Fig. 27.1). The pelvis can also be seen. It is a basin-like cavity at the upper end of the ureter into which newly formed urine passes. The bilateral ureters are thick-walled canals, connecting the kidneys to the urinary bladder. Urine is temporarily stored in the bladder until voided from the body by way of the urethra. The highlighted section in Figure 27.1 shows the arrangement of nephrons in the kidney, functional units of the kidney that can only be seen microscopically. Each kidney contains approximately 1 million nephrons. Each nephron is a complex apparatus composed of five basic parts, expressed diagrammatically in Figure 27.2.
FIGURE 27.1 Anatomy of the kidney.
FIGURE 27.2 Representation of a nephron and its blood supply.
- The glomerulus—a capillary tuft surrounded by the expanded end of a renal tubule known as Bowman’s capsule. Each glomerulus is supplied by an afferent arteriole carrying the blood in and an efferent arteriole carrying the blood out. The efferent arteriole branches into peritubular capillaries that supply the tubule.
- The proximal convoluted tubule—located in the cortex.
- The long loop of Henle—composed of the thin descending limb, which spans the medulla, and the ascending limb, which is located in both the medulla and the cortex, composed of a region that is thin and then thick.
- The distal convoluted tubule—located in the cortex.
- The collecting duct—formed by two or more distal convoluted tubules as they pass back down through the cortex and the medulla to collect the urine that drains from each nephron. Collecting ducts eventually merge and empty their contents into the renal pelvis.
The following section describes how each part of the nephron normally functions.
RENAL PHYSIOLOGY
There are three basic renal processes:
1. Glomerular filtration
2. Tubular reabsorption
3. Tubular secretion
Figure 27.3 illustrates how three different substances are variably processed by the nephron. Substance A is filtered and secreted, but not reabsorbed; substance B is filtered and a portion reabsorbed; and substance C is filtered and completely reabsorbed.1 The following is a description of how specific substances are regulated in this manner to maintain homeostasis.
FIGURE 27.3 Renal processes of filtration, reabsorption, and secretion.
Glomerular Filtration
The glomerulus is the first part of the nephron and functions to filter incoming blood. Several factors facilitate filtration. One factor is the unusually high pressure in the glomerular capillaries, which is a result of their position between two arterioles. This sets up a steep pressure difference across the walls. Another factor is the semipermeable glomerular basement membrane, which has a molecular size cutoff value of approximately 66,000 Da, about the molecular size of albumin. This means that water, electrolytes, and small dissolved solutes, such as glucose, amino acids, low-molecular-weight proteins, urea, and creatinine, pass freely through the basement membrane and enter the proximal convoluted tubule. Other blood constituents, such as albumin; many plasma proteins; cellular elements; and protein-bound substances, such as lipids and bilirubin, are too large to be filtered. In addition, because the basement membrane is negatively charged, negatively charged molecules, such as proteins, are repelled. Of the 1,200 to 1,500 mL of blood that the kidneys receive each minute (approximately one-quarter of the total cardiac output), the glomerulus filters out 125 to 130 mL of an essentially protein-free, cell-free fluid, called glomerular filtrate. The volume of blood filtered per minute is the glomerular filtration rate (GFR), and its determination is essential in evaluating renal function, as discussed in the section on Analytic Procedures.
Tubular Function
Proximal Convoluted Tubule
The proximal tubule is the next part of the nephron to receive the now cell-free and essentially protein-free blood. This filtrate contains waste products, which are toxic to the body above a certain concentration, and substances that are valuable to the body. One function of the proximal tubule is to return the bulk of each valuable substance back to the blood circulation. Thus, 75% of the water, sodium, and chloride; 100% of the glucose (up to the renal threshold); almost all of the amino acids, vitamins, and proteins; and varying amounts of urea, uric acid, and ions, such as magnesium, calcium, potassium, and bicarbonate, are reabsorbed. Almost all (98% to 100%) of uric acid, a waste product, is actively reabsorbed, only to be secreted at the distal end of the proximal tubule.
When the substances move from the tubular lumen to the peritubular capillary plasma, the process is called tubular reabsorption. With the exception of water and chloride ions, the process is active; that is, the tubular epithelial cells use energy to bind and transport the substances across the plasma membrane to the blood. The transport processes that are involved normally have sufficient reserve for efficient reabsorption, but they are saturable. When the concentration of the filtered substance exceeds the capacity of the transport system, the substance is then excreted in the urine. The plasma concentration above which the substance appears in urine is known as the renal threshold, and its determination is useful in assessing both tubular function and nonrenal disease states. A renal threshold does not exist for water because it is always transported passively through diffusion down a concentration gradient. Chloride ions in this instance diffuse in the wake of sodium.
A second function of the proximal tubule is to secrete products of kidney tubular cell metabolism, such as hydrogen ions, and drugs, such as penicillin. The term tubular secretion is used in two ways: (1) tubular secretion describes the movement of substances from peritubular capillary plasma to the tubular lumen, and (2) tubular secretion also describes when tubule cells secrete products of their own cellular metabolism into the filtrate in the tubular lumen. Transport across the membrane of the cell is again either active or passive.
Loop of Henle
The osmolality in the medulla in this portion of the nephron increases steadily from the corticomedullary junction inward and facilitates the reabsorption of water, sodium, and chloride. The hyperosmolality that develops in the medulla is continuously maintained by the loop of Henle, a hairpin-like loop between the proximal tubule and the distal convoluted tubule. The opposing flows in the loop, the downward flow in the descending limb, and the upward flow in the ascending limb are termed a countercurrent flow. To understand how the hyperosmolality is maintained in the medulla, it is best to look first at what happens in the ascending limb. Sodium and chloride are actively and passively reabsorbed into the medullary interstitial fluid along the entire length of the ascending limb. Because the ascending limb is relatively impermeable to water, little water follows, and the medullary interstitial fluid becomes hyperosmotic compared with the fluid in the ascending limb. The fluid in the ascending limb becomes hypotonic or dilute as sodium and chloride ions are reabsorbed without the loss of water, so the ascending limb is often called the diluting segment. The descending limb, in contrast to the ascending limb, is highly permeable to water and does not reabsorb sodium and chloride. The high osmolality of the surrounding interstitial medulla fluid is the physical force that accelerates the reabsorption of water from the filtrate in the descending limb. Interstitial hyperosmolality is maintained because the ascending limb continues to pump sodium and chloride ions into it. This interaction of water leaving the descending loop and sodium and chloride leaving the ascending loop to maintain a high osmolality within the kidney medulla produces hypo-osmolal urine as it leaves the loop. This process is called the countercurrent multiplier system.2
Distal Convoluted Tubule
The distal convoluted tubule is much shorter than the proximal tubule, with two or three coils that connect to a collecting duct. The filtrate entering this section of the nephron is close to its final composition. About 95% of the sodium and chloride ions and 90% of water have already been reabsorbed from the original glomerular filtrate. The function of the distal tubule is to effect small adjustments to achieve electrolyte and acid–base homeostasis. These adjustments occur under the hormonal control of both antidiuretic hormone (ADH) and aldosterone. Figure 27.4 describes the action of these hormones.
FIGURE 27.4 Antidiuretic hormone (ADH) and aldosterone control of the renal reabsorption of water and Na+. (Reprinted with permission from Kaplan A, et al. The kidney and tests of renal function. In: Kaplan A, Jack R, Orpheum KE, et al., eds. Clinical Chemistry: Interpretation and Techniques. 4th ed. Baltimore, MD: Williams & Wilkins; 1995:158, Figure 6.2.).
Antidiuretic Hormone
ADH is a peptide hormone secreted by the posterior pituitary, mainly in response to increased blood osmolality; ADH is also released when blood volume decreases by more than 5% to 10%. Large decreases of blood volume will stimulate ADH secretion even when plasma osmolality is decreased. ADH stimulates water reabsorption. The walls of the distal collecting tubules are normally impermeable to water (like the ascending loop of Henle), but they become permeable to water in ADH. Water diffuses passively from the lumen of the tubules, resulting in more concentrated urine and decreased plasma osmolality.
Aldosterone
This hormone is produced by the adrenal cortex under the influence of the renin–angiotensin mechanism. Its secretion is triggered by decreased blood flow or blood pressure in the afferent renal arteriole and by decreased plasma sodium. Aldosterone stimulates sodium reabsorption in the distal tubules and potassium and hydrogen ion secretion. Hydrogen ion secretion is linked to bicarbonate regeneration and ammonia secretion, which also occur here. In addition to these ions, small amounts of chloride ions are reabsorbed.
Collecting Duct
The collecting ducts are the final site for either concentrating or diluting urine. The hormones ADH and aldosterone act on this segment of the nephron to control reabsorption of water and sodium. Chloride and urea are also reabsorbed here. Urea plays an important role in maintaining the hyperosmolality of the renal medulla. Because the collecting ducts in the medulla are highly permeable to urea, urea diffuses down its concentration gradient out of the tubule and into the medulla interstitium, increasing its osmolality.3
Elimination of Nonprotein Nitrogen Compounds
Nonprotein nitrogen compounds (NPNs) are waste products formed in the body as a result of the degradative metabolism of nucleic acids, amino acids, and proteins. Excretion of these compounds is an important function of the kidneys. The three principal compounds are urea, creatinine, and uric acid.4,5 For a more detailed treatment of their biochemistry and disease correlations, see Chapter 12.
Urea
Urea makes up the majority (more than 75%) of the NPN waste excreted daily as a result of the oxidative catabolism of protein. Urea synthesis occurs in the liver. Proteins are broken down into amino acids, which are then deaminated to form ammonia. Ammonia is readily converted to urea, avoiding toxicity. The kidney is the only significant route of excretion for urea. It has a molecular weight of 60 Da and, therefore, is readily filtered by the glomerulus. In the collecting ducts, 40% to 60% of urea is reabsorbed. The reabsorbed urea contributes to the high osmolality in the medulla, which is one of the processes of urinary concentration mentioned earlier (see loop of Henle).
Creatinine
Muscle contains creatine phosphate, a high-energy compound for the rapid formation of adenosine triphosphate (ATP). This reaction is catalyzed by creatine kinase (CK) and is the first source of metabolic fuel used in muscle contraction. Every day, up to 20% of total muscle creatine (and its phosphate) spontaneously dehydrates and cycles to form the waste product creatinine. Therefore, creatinine levels are a function of muscle mass and remain approximately the same in an individual from day to day unless muscle mass or renal function changes. Creatinine has a molecular weight of 113 Da and is, therefore, readily filtered by the glomerulus. Unlike urea, creatinine is not reabsorbed by the tubules. However, a small amount of creatinine is secreted by the kidney tubules at high serum concentrations.
Uric Acid
Uric acid is the primary waste product of purine metabolism. The purines, adenine and guanine, are precursors of nucleic acids ATP and guanosine triphosphate, respectively. Uric acid has a molecular weight of 168 Da. Like creatinine, it is readily filtered by the glomerulus, but it then undergoes a complex cycle of reabsorption and secretion as it courses through the nephron. Only 6% to 12% of the original filtered uric acid is finally excreted. Uric acid exists in its ionized and more soluble form, usually sodium urate, at urinary pH > 5.75 (the first pKa of uric acid). At pH < 5.75, it is undissociated. This fact has clinical significance in the development of urolithiasis (formation of calculi) and gout.
Water, Electrolyte, and Acid–Base Homeostasis
Water Balance
The kidney’s contribution to water balance in the body is through water loss or water conservation, which is regulated by the hormone ADH. ADH responds primarily to changes in osmolality and intravascular volume. Increased plasma osmolality or decreased intravascular volume stimulates secretion of ADH from the posterior pituitary. ADH then increases the permeability of the distal convoluted tubules and collecting ducts to water, resulting in increased water reabsorption and excretion of more concentrated urine. In contrast, the major system regulating water intake is thirst, which appears to be triggered by the same stimuli that trigger ADH secretion.
In states of dehydration, the renal tubules reabsorb water at their maximal rate, resulting in production of a small amount of maximally concentrated urine (high urine osmolality, 1,200 mOsm/L).6 In states of water excess, the tubules reabsorb water at only a minimal rate, resulting in excretion of a large volume of extremely dilute urine (low urine osmolality, down to 50 mOsm/L).7,8 The continuous fine-tuning possible between these two extreme states results in the precise control of fluid balance in the body (Fig. 27.5).
FIGURE 27.5 Antidiuretic hormone (ADH) control of thirst mechanism.
Electrolyte Balance
The following is a brief overview of the notable ions involved in maintenance of electrolyte balance within the body. For a more comprehensive treatment of this subject, refer Chapter 16.
Sodium
Sodium is the primary extracellular cation in the human body and is excreted principally through the kidneys. Sodium balance in the body is controlled only through excretion. The renin–angiotensin–aldosterone hormonal system is the major mechanism for the control of sodium balance.
Potassium
Potassium is the main intracellular cation in the body. The precise regulation of its concentration is of extreme importance to cellular metabolism and is controlled chiefly by renal means. Like sodium, it is freely filtered by the glomerulus and then actively reabsorbed throughout the entire nephron (except for the descending limb of the loop of Henle). Both the distal convoluted tubule and the collecting ducts can reabsorb and excrete potassium, and this excretion is controlled by aldosterone. Potassium ions can compete with hydrogen ions in their exchange with sodium (in the proximal convoluted tubule); this process is used by the body to conserve hydrogen ions and, thereby, compensate in states of metabolic alkalosis.
Chloride
Chloride is the principal extracellular anion and is involved in the maintenance of extracellular fluid balance. It is readily filtered by the glomerulus and is passively reabsorbed as a counterion when sodium is reabsorbed in the proximal convoluted tubule. In the ascending limb of the loop of Henle, potassium is actively reabsorbed by a distinct chloride “pump,” which also reabsorbs sodium. This pump can be inhibited by loop diuretics, such as furosemide. As expected, the regulation of chloride is controlled by the same forces that regulate sodium.6,8
Phosphate, Calcium, and Magnesium
The phosphate ion occurs in higher concentrations in the intracellular than in the extracellular fluid environments. It exists as either a protein-bound or a non–protein-bound form; homeostatic balance is chiefly determined by proximal tubular reabsorption under the control of parathyroid hormone (PTH). Calcium, the second most predominant intracellular cation, is the most important inorganic messenger in the cell. It also exists in protein-bound and non–protein-bound states. Calcium in the non–protein-bound form is either ionized and physiologically active or nonionized and complexed to small, diffusible ions, such as phosphate and bicarbonate. The ionized form is freely filtered by the glomerulus and reabsorbed in the tubules under the control of PTH. However, renal control of calcium concentration is not the major means of regulation. PTH- and calcitonin-controlled regulation of calcium absorption from the gut and bone stores is more important than renal secretion or reabsorption. Magnesium, a major intracellular cation, is important as an enzymatic cofactor. Like phosphate and calcium, it exists in both protein-bound and ionized states. The ionized fraction is easily filtered by the glomerulus and reabsorbed in the tubules under the influence of PTH. See Chapter 24 for more detailed information.
Acid–Base Balance
Many nonvolatile acidic waste products are formed by normal body metabolism each day. Carbonic acid, lactic acid, ketoacids, and others must be continually transported in the plasma and excreted from the body, causing only minor alterations in physiologic pH. The renal system constitutes one of three means by which constant control of overall body pH is accomplished. The other two strategies involved in this regulation are the respiratory system and the acid–base buffering system.9
The kidneys manage their share of the responsibility for controlling body pH by dual means: conserving bicarbonate ions and removing metabolic acids. For a more in-depth examination of these processes, refer Chapter 17.
Regeneration of Bicarbonate Ions
In a complicated process, bicarbonate ions are first filtered out of the plasma by the glomerulus. In the lumen of the renal tubules, this bicarbonate combines with hydrogen ions to form carbonic acid, which subsequently degrades to carbon dioxide (CO2) and water. This CO2 then diffuses into the brush border of the proximal tubular cells, where it is reconverted by carbonic anhydrase to carbonic acid and then degrades back to hydrogen ions and regenerated bicarbonate ions. This regenerated bicarbonate is transported into the blood to replace what was depleted by metabolism; the accompanying hydrogen ions are secreted back into the tubular lumen, and from there, they enter the urine. Filtered bicarbonate is “reabsorbed” into the circulation, helping to return blood pH to its optimal level and effectively functioning as another buffering system.
Excretion of Metabolic Acids
Hydrogen ions are manufactured in the renal tubules as part of the regeneration mechanism for bicarbonate. These hydrogen ions, as well as others that are dissociated from nonvolatile organic acids, are disposed of by several different reactions with buffer bases.
Reaction with Ammonia (NH3)
The glomerulus does not filter NH3. However, this substance is formed in the renal tubules when the amino acid glutamine is deaminated by glutaminase. This NH3 then reacts with secreted hydrogen ions to form ammonium ions (NH4+), which are unable to readily diffuse out of the tubular lumen and, therefore, are excreted into the urine. This mode of acid excretion is the primary means by which the kidneys compensate for states of metabolic acidosis.
Reaction with Monohydrogen Phosphate 
Phosphate ions filtered by the glomerulus can exist in the tubular fluid as disodium hydrogen phosphate (Na2HPO4) (dibasic). This compound can react with hydrogen ions to yield dihydrogen phosphate (monobasic), which is then excreted. The released sodium then combines with bicarbonate to yield sodium bicarbonate and is reabsorbed. These mechanisms can excrete increasing amounts of metabolic acid until a maximum urine pH of approximately 4.4 is reached. After this, renal compensation is unable to adjust to any further decreases in blood pH, and metabolic acidosis ensues. Few free hydrogen ions are excreted directly in the urine.
Endocrine Function
In addition to numerous excretory and regulatory functions, the kidney has endocrine functions as well. It is both a primary endocrine site, as the producer of its own hormones, and a secondary site, as the target locus for hormones manufactured by other endocrine organs. The kidneys synthesize renin, erythropoietin, 1,25-dihydroxy vitamin D3, and the prostaglandins.
Renin
Renin is the initial component of the renin–angiotensin–aldosterone system. Renin is produced by the juxtaglomerular cells of the renal medulla when extracellular fluid volume or blood pressure decreases. It catalyzes the synthesis of angiotensin by cleavage of the circulating plasma precursor angiotensinogen. Angiotensin is converted to angiotensin II by angiotensin-converting enzyme. Angiotensin II is a powerful vasoconstrictor that increases blood pressure and stimulates release of aldosterone from the adrenal cortex. Aldosterone, in turn, promotes sodium reabsorption and water conservation.7,8 For a more detailed look at the complexities of this feedback loop, see Chapter 21.
Erythropoietin
Erythropoietin is a single-chain polypeptide produced by cells close to the proximal tubules, and its production is regulated by blood oxygen levels. Hypoxia produces increased serum concentrations within 2 hours. Erythropoietin acts on the erythroid progenitor cells in the bone marrow, increasing the number of red blood cells (RBCs). In chronic renal insufficiency, erythropoietin production is significantly reduced. The routine administration of recombinant human erythropoietin is common in chronic renal failure patients. Before this therapy was available, anemia was a clinical reality in these patients.4,7 Erythropoietin concentrations in blood can be measured by immunoassays. Recombinant human erythropoietin has also been used in sports doping to stimulate erythrocyte production and increase the oxygen-carrying capacity in the blood of endurance athletes. Assays capable of detecting posttranslational modifications on erythropoietin have been produced and are capable of distinguishing exogenous from endogenous erythropoietin.
1,25-Dihydroxy Vitamin D3
The kidneys are the sites of formation of the active form of vitamin D, 1,25-(OH)2 vitamin D3. This form of vitamin D is one of three major hormones that determine phosphate and calcium balance and bone calcification in the human body. Chronic renal insufficiency is, therefore, often associated with osteomalacia (inadequate bone calcification, the adult form of rickets), owing to the continual distortion of normal vitamin D metabolism.
Prostaglandins
The prostaglandins are a group of potent cyclic fatty acids formed from essential (dietary) fatty acids, primarily arachidonic acid. They are formed in almost all tissue and their actions are diverse. The prostaglandins produced by the kidneys increase renal blood flow, sodium and water excretion, and renin release. They act to oppose renal vasoconstriction due to angiotensin and norepinephrine.
ANALYTIC PROCEDURES
All laboratory methods used for the evaluation of renal function rely on the measurement of waste products in blood, usually urea and creatinine, which accumulate when the kidneys begin to fail. Renal failure must be advanced, with only about 20% to 30% of the nephrons still functioning, before the concentration of either substance begins to increase in the blood. The rate at which creatinine and urea are removed or cleared from the blood into the urine is termed clearance. Clearance is defined as that volume of plasma from which a measured amount of substance can be completely eliminated into the urine per unit of time expressed in milliliters per minute.5 Calculation of creatinine clearance has become the standard laboratory method for determining the GFR. Urea clearance was one of the first clearance tests performed; however, it is no longer widely used since it does not accurately provide a full clearance assessment. Older tests used administration of insulin, sodium [125I] iothalamate, or p-aminohippurate to assess glomerular filtration or tubular secretion. These tests are difficult to administer and are no longer common.
Creatinine Clearance
Creatinine is a nearly ideal substance for the measurement of clearance. It is an endogenous metabolic product synthesized at a constant rate for a given individual and cleared essentially only by glomerular filtration. It is not reabsorbed and is only slightly secreted by the proximal tubule. Serum creatinine levels are higher in males than in females due to the direct correlation with muscle mass. Analysis of creatinine is simple and inexpensive using colorimetric assays; however, different methods for assaying plasma creatinine, such as kinetic or enzymatic assays, have varying degrees of accuracy and imprecision (see Chapter 12).
Creatinine clearance is derived by mathematically relating the serum creatinine concentration to the urine creatinine concentration excreted during a period of time, usually 24 hours. Specimen collection, therefore, must include both a 24-hour urine specimen and a serum creatinine value, ideally collected at the midpoint of the 24-hour urine collection. The urine container must be kept refrigerated throughout the duration of both the collection procedure and the subsequent storage period until laboratory analysis can be performed. The concentration of creatinine in both serum and urine is measured by the applicable methods discussed in Chapter 12. The total volume of urine is carefully measured, and the creatinine clearance is calculated using the following formula:

where Cr is creatinine clearance, UCr is urine creatinine clearance, VUr is urine volume excreted in 24 hours, PCr is serum creatinine concentration, and 1.73/A is normalization factor for body surface area (1.73 is the generally accepted average body surface in square meters, and A is the actual body surface area of the individual determined from height and weight). If the patient’s body surface area varies greatly from the average (e.g., obese or pediatric patients), this correction for body mass must be included in the formula. The reference range for creatinine clearance is lower in females compared with males and normally decreases with age.
Estimated GFR
The National Kidney Foundation recommends that estimated GFR (eGFR) be calculated each time a serum creatinine level is reported. (Additional information is available at the National Kidney Foundation Web site at http://www.kidney.org/.) The equation is used to predict GFR and is based on serum creatinine, age, body size, gender, and race, without the need of a urine creatinine. Because the calculation does not require a timed urine collection, it should be used more often than the traditional creatinine clearance and result in earlier detection of chronic kidney disease (CKD). There are a number of formulas that can be used to estimate GFR on the basis of serum creatinine levels.
Cockcroft-Gault Formula
The Cockcroft-Gault formula is one of the first formulas used to estimate GFR. This formula predicts creatinine clearance, and the results are not corrected for body surface area. This equation assumes that women will have a 15% lower creatinine clearance than men at the same level of serum creatinine.
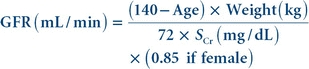
Modification of Diet in Renal Disease Formula
The modification of diet in renal disease (MDRD) formula was developed in the Modification of Diet in Renal Disease Study of chronic renal insufficiency. The study showed that the MDRD formula provided a more accurate assessment of GFR than the Cockcroft-Gault formula. The MDRD formula was validated in a large population that included European Americans and African Americans. It does not require patient weight and is corrected for body surface area. The MDRD formula is known to underestimate the GFR in healthy patients with GFRs over 60 mL/min and to overestimate GFR in underweight patients. The four-variable MDRD equation includes age, race, gender, and serum creatinine as variables.

CKD-EPI Formula
The CKD-EPI (Chronic Kidney Disease Epidemiology Collaboration) formula was published in 2009.10 It was developed in an effort to create a formula more accurate than the MDRD formula. Multiple studies have shown the CKD-EPI formula to perform better and with less bias than the MDRD formula, especially in patients with higher GFR. Many laboratories still use the MDRD formula; however, some have converted to the CKD-EPI formula.
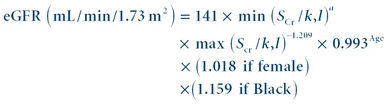
k is 0.7 for females and 0.9 for males, a is −0.329 for females and −0.411 for males, min indicates the minimum of SCr/k or 1, and max indicates the maximum of SCr/k or 1.
Cystatin C
Cystatin C is a low-molecular-weight protein produced at a steady rate by most body tissues. It is freely filtered by the glomerulus, reabsorbed, and catabolized by the proximal tubule. Levels of cystatin C rise more quickly than creatinine levels in acute kidney injury (AKI). Plasma concentrations appear to be unaffected by diet, gender, race, age, and muscle mass. Studies have shown measurement of cystatin C to be at least as useful as serum creatinine and creatinine clearance in detecting early changes in kidney function. A rise in cystatin C is often detectable before there is a measureable decrease in the GFR or increase in creatinine. Cystatin C can be measured by immunoassay methods.11 Some findings suggest that an equation that uses both serum creatinine and cystatin C with age, sex, and race would be better than equations that use only one of these serum markers.12,13
Biologic Variation
When the same test is performed on various individuals, we find that the mean of each person’s results is not the same, showing that individual homeostatic setting points often vary. Biologic variation is defined as the random fluctuation around a homeostatic setting point.14 This includes the fluctuation around the homeostatic setting point for a single individual, termed within-subject biologic variation, and differences between the homeostatic setting points of multiple individuals, termed between-subject biologic variation. In the case of creatinine, levels for an individual differ slightly over time, and the mean values of all individuals vary significantly from each other. Therefore, each individual’s results span only a small portion of the population-based reference interval. This means that for creatinine, within-subject biologic variation is less than between-subject biologic variation. When this is true of a given analyte, the analyte is said to have marked individuality. Interestingly, the between-subject biologic variation is much smaller for cystatin C compared with creatinine, showing that population-based reference values are more useful for cystatin C compared with creatinine. However, the within-subject variation is greater for cystatin C compared with creatinine. As a result, creatinine is more helpful in monitoring renal function over time for a given individual, whereas cystatin C is potentially more useful for detecting minor renal impairment.
β2-Microglobulin
β2-Microglobulin (β2-M) is a small, nonglycosylated peptide (molecular weight, 11,800 Da) found on the surface of most nucleated cells. The plasma membrane sheds β2-M at a constant rate, as a relatively intact molecule. β2-M is easily filtered by the glomerulus, and 99.9% is reabsorbed by the proximal tubules and catabolized. Elevated levels in serum indicate increased cellular turnover as seen in myeloproliferative and lymphoproliferative disorders, inflammation, and renal failure. Both blood and urine β2-M tests may be ordered to evaluate kidney damage and to distinguish between disorders that affect the glomeruli and the renal tubules. Measurement of serum β2-M is used clinically to assess renal tubular function in renal transplant patients, with elevated levels indicating organ rejection.
Myoglobin
Myoglobin is a low-molecular-weight protein (16,900 Da) associated with acute skeletal and cardiac muscle injury. Myoglobin functions to bind and transport oxygen from the plasma membrane to the mitochondria in muscle cells. Blood levels of myoglobin can rise very quickly with severe muscle injury. In rhabdomyolysis, myoglobin release from skeletal muscle is sufficient to overload the proximal tubules and cause acute renal failure. Early diagnosis and aggressive treatment of elevated myoglobin may prevent or lessen the severity of renal failure. Serum and urine myoglobin can be measured easily and rapidly by immunoassays.
Albuminuria
The term albuminuria, previously referred to as microalbuminuria, describes the presence of albumin in the urine. Urine albumin measurement is important in the management of patients with diabetes mellitus, who are at serious risk for developing nephropathy over their lifetime. In the early stages of nephropathy, there is renal hypertrophy, hyperfunction, and increased thickness of the glomerular and tubular basement membranes. In this early stage, there are no overt signs of renal dysfunction. In the next 7 to 10 years, there is progression to glomerulosclerosis, with increased glomerular capillary permeability. This permeability allows small (micro) amounts of albumin to pass into the urine. If detected in this early phase, rigid glucose control, along with treatment to prevent hypertension, can be instituted and progression to kidney failure prevented. Quantitative albumin-specific immunoassays, usually using nephelometry or immunoturbidimetry, are widely used. For a 24-hour urine collection, 30 to 300 mg of albumin is diagnostic of albuminuria. A 24-hour urine collection is preferred, but a random urine sample in which the ratio of albumin to creatinine is measured, is common. An albumin to creatinine ratio (ACR) of greater than 30 mg/g is diagnostic of albuminuria.
Neutrophil Gelatinase–Associated Lipocalin
Neutrophil gelatinase–associated lipocalin (NGAL) is a 25-kDa protein expressed by neutrophils and epithelial cells including those of the proximal tubule. The gene encoding NGAL is upregulated in the presence of renal ischemia, tubule injury, and nephrotoxicity.15 It can be measured in plasma and urine and is elevated within 2 to 6 hours of AKI. NGAL has been shown to be a useful early predictor of AKI and has prognostic value for clinical endpoints, such as initiation of dialysis and mortality. However, urinary NGAL excretion may also arise from systemic stress in the absence of AKI, limiting its specificity.
NephroCheck
NephroCheck is the first FDA-cleared test used to determine if critically ill patients are at risk for developing moderate to severe AKI in the 12 hours following administration of the test. NephroCheck quantifies concentrations of tissue inhibitor of metalloproteinase 2 (TIMP-2) and insulin-like growth factor binding protein 7 (IGFBP-7) in urine specimens and multiplies the results to generate a quantitative AKI risk index (AKIRisk Score). The two biomarkers invoke cell cycle arrest in response to tissue insults that cause AKI.16 Elevation of these two biomarkers is thought to signal the kidney’s attempt to protect itself from harmful insults and signal the increased risk for imminent AKI. The biomarker concentrations have been shown to correspond to severity across all stages of AKI.
Urinalysis
Urinalysis (UA) permits a detailed, in-depth assessment of renal status with an easily obtained specimen. UA also serves as a quick indicator of an individual’s glucose status and hepatic–biliary function. Routine UA includes assessment of physical characteristics, chemical analyses, and a microscopic examination of the sediment from a (random) urine specimen.
Specimen Collection
The importance of a properly collected and stored specimen for UA cannot be overemphasized. Initial morning specimens are preferred, particularly for protein analyses, because they are more concentrated from overnight retention in the bladder. The specimen should be obtained by a clean midstream catch or catheterization. The urine should be freshly collected into a clean, dry container with a tight-fitting cover. It must be analyzed within 1 hour of collection if held at room temperature or else refrigerated at 2°C to 8°C for not more than 8 hours before analysis. If not assayed within these time limits, several changes will occur. Bacterial multiplication will cause false-positive nitrite tests, and urease-producing organisms will degrade urea to ammonia and alkalinize the pH. Loss of CO2 by diffusion into the air adds to this pH elevation, which, in turn, causes cast degeneration and red cell lysis.
Physical Characteristics
Visual Appearance
Color intensity of urine correlates with concentration: the darker the color, the more concentrated is the specimen. The various colors observed in urine are a result of different excreted pigments. Yellow and amber are generally due to urochromes (derivatives of urobilin, the end product of bilirubin degradation), whereas a yellowish-brown to green color is a result of bile pigment oxidation. Red and brown after standing are due to porphyrins, whereas reddish-brown in fresh specimens comes from hemoglobin or red cells. Brownish-black after standing is seen in alkaptonuria (a result of excreted homogentisic acid) and in malignant melanoma (in which the precursor melanogen oxidizes in the air to melanin). Drugs and some foods, such as beets, may also alter urine color.
Odor
Odor ordinarily has little diagnostic significance. The characteristic pungent odor of fresh urine is due to volatile aromatic acids, in contrast to the typical ammonia odor of urine that has been allowed to stand. Urinary tract infections impart a noxious, fecal smell to urine, whereas the urine of diabetics often smells fruity as a result of ketones. Certain inborn errors of metabolism, such as maple sugar urine disease, are associated with characteristic urine odors.
Turbidity
The cloudiness of a urine specimen depends on pH and dissolved solids composition. Turbidity generally may be due to gross bacteriuria, whereas a smoky appearance is seen in hematuria. Threadlike cloudiness is observed when the specimen is full of mucus. In alkaline urine, suspended precipitates of amorphous phosphates and carbonates may be responsible for turbidity, whereas in acidic urine, amorphous urates may be the cause.17
Volume
The volume of urine excreted indicates the balance between fluid ingestion and water lost from the lungs, sweat, and intestine. Most adults produce from 750 to 2,000 mL every 24 hours, averaging about 1.5 L per person. Polyuria is observed in diabetes mellitus and insipidus (in insipidus, as a result of lack of ADH), as well as in chronic renal disease, acromegaly (overproduction of the growth hormone somatostatin), and myxedema (hypothyroid edema). Anuria or oliguria (<200 mL/d) is found in nephritis, urinary tract obstruction, AKI, and kidney failure.
Specific Gravity
The specific gravity (SG) of urine is the weight of 1 mL of urine in grams divided by the weight of 1 mL of water. SG gives an indication of the density of a fluid that depends on the concentration of dissolved total solids. SG varies with the solute load to be excreted (consisting primarily of NaCl and urea), as well as with the urine volume. It is used to assess the state of hydration/dehydration of an individual or as an indicator of the concentrating ability of the kidneys.
The most commonly encountered analytic method consists of a refractometer, or total solids meter. This operates on the principle that the refractive index of a urine specimen will vary directly with the total amount of dissolved solids in the sample. This instrument measures the refractive index of the urine as compared with water on a scale that is calibrated directly into the ocular and viewed while held up to a light source. Correct calibration is vital for accuracy. Most recently, an indirect colorimetric reagent strip method for assaying SG has been added to most dipstick screens. Unlike the refractometer, dipsticks measure only ionic solutes and do not take into account glucose or protein.
The normal range for urinary SG is 1.003 to 1.035 g/mL. SG can vary in pathologic states. Low SG can occur in diabetes insipidus, pyelonephritis, and glomerulonephritis, in which the renal concentrating ability has become dysfunctional. High SG can be seen in diabetes mellitus, congestive heart failure, dehydration, adrenal insufficiency, liver disease, and nephrosis. SG will increase about 0.004 units for every 1% change in glucose concentration and about 0.003 units for every 1% change in protein. Fixed SG (isosthenuria) around 1.010 is observed in severe renal damage, in which the kidney excretes urine that is iso-osmotic with the plasma. This generally occurs after an initial period of anuria because the damaged tubules are unable to concentrate or dilute the glomerular filtrate.16
pH
Determinations of urinary pH must be performed on fresh specimens because of the significant tendency of urine to alkalinize on standing. Normal urine pH falls within the range of 4.7 to 7.8. Acidity in urine (pH < 7.0) is primarily caused by phosphates, which are excreted as salts conjugated to Na+, K+, Ca2+, and NH4+. Acidity also reflects the excretion of the nonvolatile metabolic acids pyruvate, lactate, and citrate. Owing to the Na+/H+ exchange pump mechanism of the renal tubules, pH (H+ concentration) increases as sodium is retained. Pathologic states, in which increased acidity is observed, include systemic acidosis, as seen in diabetes mellitus, and renal tubular acidosis (RTA). In RTA, the tubules are unable to excrete excess H+ even though the body is in metabolic acidosis, and urinary pH remains around 6.
Alkaline urine (pH > 7.0) is observed postprandially as a normal reaction to the acidity of gastric HCl dumped into the duodenum and then into the circulation or following ingestion of alkaline food or medications. Urinary tract infections and bacterial contamination also will alkalinize pH. Medications such as potassium citrate and sodium bicarbonate will reduce urine pH. Alkaline urine is also found in Fanconi syndrome, a congenital generalized aminoaciduria resulting from defective proximal tubular function.
Chemical Analyses
Routine urine chemical analysis is rapid and easily performed with commercially available reagent strips or dipsticks. These strips are plastic coated with different reagent bands directed toward different analytes. When dipped into urine, a color change signals a deviation from normality. Colors on the dipstick bands are matched against a color chart provided with the reagents. Automated and semiautomated instruments that detect by reflectance photometry provide an alternative to the color chart and offer better precision and standardization. Abnormal results are followed up by specific quantitative or confirmatory urine assays. The analytes routinely tested are glucose, protein, ketones, nitrite, leukocyte esterase, bilirubin/urobilinogen, and hemoglobin/blood.
Glucose and Ketones
These constituents are normally absent in urine. The clinical significance of these analytes and their testing methods are discussed in Chapter 14.
Protein
Reagent strips for UA are used as a general qualitative screen for proteinuria. They are primarily specific for albumin, but they may give false-positive results in specimens that are alkaline and highly buffered. Positive dipstick results should be confirmed by more specific chemical assays, as described in Chapter 11, or more commonly by microscopic evaluation to detect casts.
Nitrite
This assay semiquantitates the amount of urinary reduction of nitrate (on the reagent strip pad) to nitrite by the enzymes of gram-negative bacteria. A negative result does not mean that no bacteriuria is present. A gram-positive pathogen, such as Staphylococcus, Enterococcus, or Streptococcus, may not produce nitrate-reducing enzymes; alternatively, a spot urine sample may not have been retained in the bladder long enough to pick up a sufficient number of organisms to register on the reagent strip.16
Leukocyte Esterase
White blood cells (WBCs), especially phagocytes, contain esterases. A positive dipstick for esterases indicates possible WBCs in urine.
Bilirubin/Urobilinogen
Hemoglobin degradation ultimately results in the formation of the waste product bilirubin, which is then converted to urobilinogen in the gut through bacterial action. Although most of this urobilinogen is excreted as urobilin in the feces, some is excreted in urine as a colorless waste product. This amount is normally too small to be detected as a positive dipstick reaction. In conditions of prehepatic, hepatic, and posthepatic jaundice, however, urine dipstick tests for urobilinogen and bilirubin may be positive or negative, depending on the nature of the patient’s jaundice. A more in-depth view of bilirubin metabolism and assay methods is given in Chapter 25. Reagent strip tests for bilirubin involve diazotization and formation of a color change. Dipstick methods for urobilinogen differ, but most rely on a modification of the Ehrlich reaction with p-dimethylaminobenzaldehyde.16
Hemoglobin/Blood
Intact or lysed RBCs produce a positive dipstick result. The dipstick will be positive in cases of renal trauma/injury, infection, and obstruction that result from calculi or neoplasms.
Sediment Examination
Centrifuged, decanted urine aliquot leaves behind a sediment of formed elements that is used for microscopic examination.
Cells
For cellular elements, evaluation is best accomplished by counting and then taking the average of at least 10 microscopic fields.
Red Blood Cells
Erythrocytes greater in number than 0 to 2/high-power field (HPF) are considered abnormal. Such hematuria may result simply from severe exercise or menstrual blood contamination. However, it may also be indicative of trauma, particularly vascular injury, renal/urinary calculi obstruction, pyelonephritis, or cystitis. Hematuria in conjunction with leukocytes is diagnostic of infection.
White Blood Cells
Leukocytes greater in number than 0 to 1/HPF are considered abnormal. These cells are usually polymorphonuclear phagocytes, commonly known as segmented neutrophils. They are observed when there is acute glomerulonephritis, urinary tract infection, or inflammation of any type. In hypotonic urine (low osmotic concentration), WBCs can become enlarged, exhibiting a sparkling effect in their cytoplasmic granules. These cells possess a noticeable Brownian motion and are called glitter cells, but they have no pathologic significance.
Epithelial Cells
Several types of epithelial cells are frequently encountered in normal urine because they are continuously sloughed off the lining of the nephrons and urinary tract. Large, flat, squamous vaginal epithelia are often seen in urine specimens from female patients, and samples heavily contaminated with vaginal discharge may show clumps or sheets of these cells. Renal epithelial cells are round, uninucleated cells and, if present in numbers greater than 2/HPF, indicate clinically significant active tubular injury or degeneration. Transitional bladder epithelial cells (urothelial cells) may be flat, cuboidal, or columnar and also can be observed in urine on occasion. Large numbers will be seen only in cases of urinary catheterization, bladder inflammation, or neoplasm.
Miscellaneous Elements
Spermatozoa are often seen in the urine of both males and females. They are usually not reported because they are of no pathologic significance. In males, however, their presence may indicate prostate abnormalities. Yeast cells are also frequently found in urine specimens. Because they are extremely refractile and of a similar size to RBCs, they can easily be mistaken under low magnification. Higher power examination for budding or mycelial forms differentiates these fungal elements from erythrocytes. Parasites found in urine are generally contaminants from fecal or vaginal material. In fecal contaminant category, the most commonly encountered organism is Enterobius vermicularis (pinworm) infestation in children. In the vaginal contaminant category, the most common is the intensely motile flagellate, Trichomonas vaginalis. A true urinary parasite, sometimes seen in patients from endemic areas of the world, is the ova of the trematode Schistosoma haematobium. This condition will usually occur in conjunction with a significant hematuria.16
Bacteria
Normal urine is sterile and contains no bacteria. Small numbers of organisms seen in a fresh urine specimen usually represent skin or air contamination. In fresh specimens, however, large numbers of organisms, or small numbers accompanied by WBCs and the symptoms of urinary tract infection, are highly diagnostic for true infection. Clinically significant bacteriuria is considered to be more than 20 organisms/HPF or, alternatively, 105 or greater registered on a microbiologic colony count. Most pathogens seen in urine are gram-negative coliforms (microscopic “rods”) such as Escherichia coli and Proteus spp. Asymptomatic bacteriuria, in which there are significant numbers of bacteria without appreciable clinical symptoms, occurs somewhat commonly in young girls, pregnant women, and patients with diabetes. This condition must be taken seriously because, if left untreated, it may result in pyelonephritis and, subsequently, permanent renal damage.
Casts
Casts are precipitated, cylindrical impressions of the nephrons. They comprise Tamm-Horsfall mucoprotein (uromucoid) from the tubular epithelia in the ascending limb of the loop of Henle. Casts form whenever there is sufficient renal stasis, increased urine salt or protein concentration, and decreased urine pH. In patients with severe renal disease, truly accurate classification of casts may require use of “cytospin” centrifugation and Papanicolaou staining for adequate differentiation. Unlike cells, casts should be examined under low power and are most often located around the edges of the coverslip.
Hyaline
The matrix of these casts is clear and gelatinous, without embedded cellular or particulate matter. They may be difficult to visualize unless a high-intensity lamp is used. Their presence indicates glomerular leakage of protein. This leakage may be temporary (as a result of fever, upright posture, dehydration, or emotional stress) or may be permanent. Their occasional presence is not considered pathologic.
Granular
These casts are descriptively classified as either coarse or finely granular. The type of embedded particulate matter is simply a matter of the amount of degeneration that the epithelial cell inclusions have undergone. Their occasional presence is not pathologic; however, large numbers may be found in chronic lead toxicity and pyelonephritis.
Cellular
Several different types of casts are included in this category. RBC or erythrocytic casts are always considered pathologic because they are diagnostic for glomerular inflammation that results in renal hematuria. They are seen in subacute bacterial endocarditis, kidney infarcts, collagen diseases, and acute glomerulonephritis. WBC or leukocytic casts are also always considered pathologic because they are diagnostic for inflammation of the nephrons. They are observed in pyelonephritis, nephrotic syndrome, and acute glomerulonephritis. In asymptomatic pyelonephritis, these casts may be the only clue to detection. Epithelial cell casts are sometimes formed by fusion of renal tubular epithelia after desquamation; occasional presence is normal. Many, however, are observed in severe desquamative processes and renal stases that occur in heavy metal poisoning, renal toxicity, eclampsia, nephrotic syndrome, and amyloidosis. Waxy casts are uniformly yellowish, refractile, and brittle appearing, with sharply defined, often broken edges. They are almost always pathologic because they indicate tubular inflammation or deterioration. They are formed by renal stasis in the collecting ducts and are, therefore, found in chronic renal diseases. Fatty casts are abnormal, coarse, granular casts with lipid inclusions that appear as refractile globules of different sizes. Broad (renal failure) casts may be up to two to six times wider than “regular” casts and may be cellular, waxy, or granular in composition. Like waxy casts, they are derived from the collecting ducts in severe renal stasis.
Crystals
Acid Environment
Crystals seen in urine with pH values of less than 7 include calcium oxalate, which are normal colorless octahedrons or “envelopes”; they may have an almost starlike appearance. Also seen are amorphous urates, normal yellow-red masses with a grain of sand appearance. Uric acid crystals found in this environment are normal yellow to red-brown crystals that appear in extremely irregular shapes, such as rosettes, prisms, or rhomboids. Cholesterol crystals in acid urine are clear, flat, rectangular plates with notched corners. They may be seen in nephrotic syndrome and in conditions producing chyluria and are always considered abnormal. Cystine crystals are also sometimes observed in acid urine; they are highly pathologic and appear as colorless, refractile, nearly flat hexagons, somewhat similar to uric acid. These are observed in homocystinuria (an aminoaciduria resulting in mental retardation) and cystinuria (an inherited defect of cystine reabsorption resulting in renal calculi).
Alkaline Environment
Crystals seen in urine with pH values greater than 7 include amorphous phosphates, which are normal crystals that appear as fine, colorless masses, resembling sand. Also seen are calcium carbonate crystals, which are normal forms that appear as small, colorless dumbbells or spheres. Triple phosphate crystals are also observed in alkaline urines; they are colorless prisms of three to six sides, resembling “coffin lids.” Ammonium biurate crystals are normal forms occasionally found in this environment, appearing as spiny, yellow-brown spheres, or “thorn apples.”
Other
Sulfonamide crystals are abnormal precipitates shaped like yellow-brown sheaves, clusters, or needles, formed in patients undergoing antimicrobial therapy with sulfa drugs. These drugs are seldom used today. Tyrosine/leucine crystals are abnormal types shaped like clusters of smooth, yellow needles or spheres. These are sometimes seen in patients with severe liver disease.16
PATHOPHYSIOLOGY
Glomerular Diseases
Disorders or diseases that directly damage the renal glomeruli may, at least initially, exhibit normal tubular function. With time, however, disease progression involves the renal tubules as well. The following syndromes have discrete symptoms that are recognizable by their patterns of clinical laboratory findings.
Acute Glomerulonephritis
Pathologic lesions in acute glomerulonephritis primarily involve the glomerulus. Histologic examination shows large, inflamed glomeruli with a decreased capillary lumen. Abnormal laboratory findings usually include rapid onset of hematuria and proteinuria (usually albumin and generally <3 g/d). The rapid development of a decreased GFR, anemia, elevated blood urea nitrogen (BUN) and serum creatinine, oliguria, sodium and water retention (with consequent hypertension and some localized edema), and, sometimes, congestive heart failure is typical. Numerous hyaline and granular casts are generally seen on UA. The actual RBC casts are regarded as highly suggestive of this syndrome. Acute glomerulonephritis is often related to recent infection by group A β-hemolytic streptococci. It is theorized that circulating immune complexes trigger a strong inflammatory response in the glomerular basement membrane, resulting in a direct injury to the glomerulus itself. Other possible causes include drug-related exposures, acute kidney infections due to other bacterial (and, possibly, viral) agents, and other systemic immune complex diseases, such as systemic lupus erythematosus and bacterial endocarditis.
Chronic Glomerulonephritis
Lengthy glomerular inflammation may lead to glomerular scarring and the eventual loss of functioning nephrons. This process often goes undetected for lengthy periods because only minor decreases in renal function occur at first and only slight proteinuria and hematuria are observed. Gradual development of uremia (or azotemia, excess nitrogen compounds in the blood) may be the first sign of this process.
Nephrotic Syndrome
Nephrotic syndrome (Fig. 27.6) can be caused by several different diseases that result in injury and increased permeability of the glomerular basement membrane. This defect almost always yields several abnormal findings, such as massive proteinuria (>3.5 g/d) and resultant hypoalbuminemia. The subsequent decreased plasma oncotic pressure causes a generalized edema as a result of the movement of body fluids out of vascular and into interstitial spaces. Other hallmarks of this syndrome are hyperlipidemia and lipiduria. Lipiduria takes the form of oval fat bodies in the urine. These bodies are degenerated renal tubular cells containing reabsorbed lipoproteins. Primary causes are associated directly with glomerular disease states.
FIGURE 27.6 Pathophysiology of nephrotic syndrome.
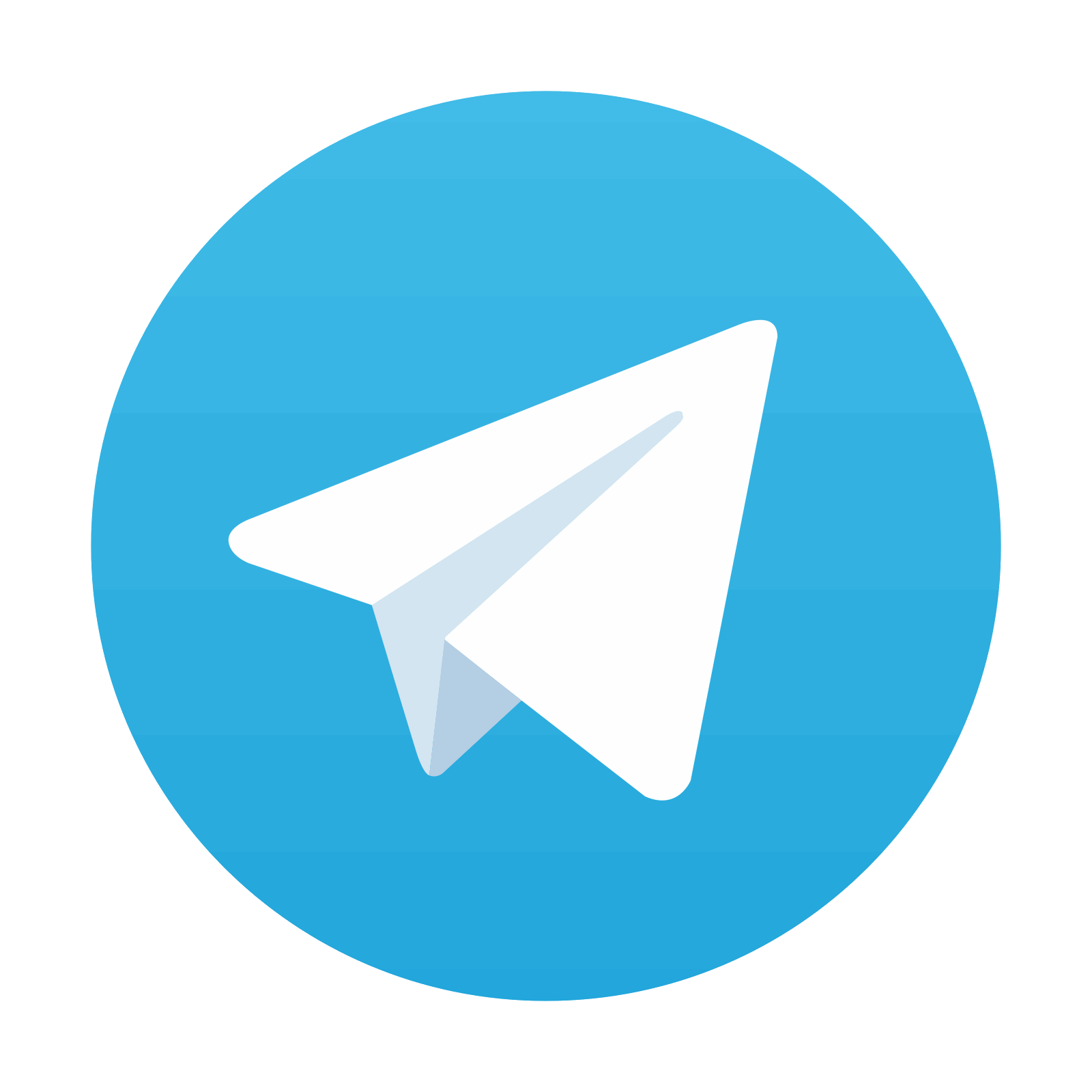
Stay updated, free articles. Join our Telegram channel

Full access? Get Clinical Tree
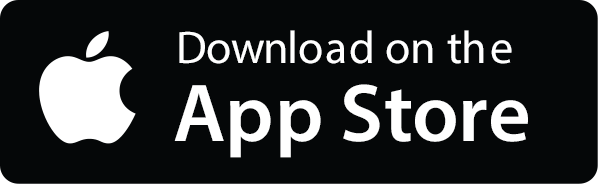
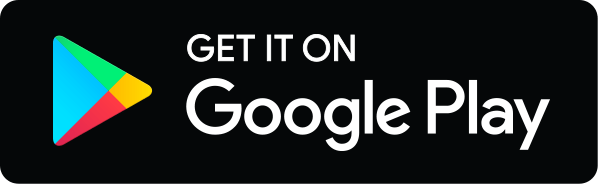