41
Protein Synthesis Inhibitors and Miscellaneous Antibacterial Agents
This chapter will be most useful after having a basic understanding of the material in Chapter 55, Protein Synthesis Inhibitors and Miscellaneous Antibacterial Agents in Goodman & Gilman’s The Pharmacological Basis of Therapeutics, 12th Edition. In addition to the material presented here Chapter 55 contains:
• Table 55-2 which provides information about the activity of most of the antibacterial agents covered in this chapter against key gram-positive pathogens
DRUGS INCLUDED IN THIS CHAPTER
• Azithromycin (ZITHROMAX)
• Bacitracin
• Chloramphenicol
• Clarithromycin (BIAXIN, others)
• Clindamycin (CLEOCIN PHOSPHATE, others)
• Daptomycin (CUBICIN)
• Doxycycline
• Erythromycin
• Linezolid (ZYVOX)
• Mupirocin (BACTROBAN)
• Polymyxin
• Quinupristin/dalfopristin (SYNERCID)
• Spectinomycin
• Teicoplanin
• Telithromycin (KETEX)
• Tetracycline
• Tigecycline
• Vancomycin (VANCOCIN)
LEARNING OBJECTIVES
Understand the mechanisms of action and resistance of tetracyclines, macrolides, vancomycin, linezolid, daptomycin, and quinupristin/dalfopristin
Describe the unique toxicities of antibiotics that are inhibitors of bacterial protein synthesis
Describe the uses and untoward reactions of vancomycin
Identify the drug–drug interactions that occur with some of these antibiotics
Understand how linezolid, daptomycin, and quinupristin/dalfopristin are used to treat methicillin-resistant and vancomycin-resistant organisms
MECHANISMS OF ACTION AND RESISTANCE OF PROTEIN SYNTHESIS INHIBITORS
FIGURE 41-1 Inhibition of bacterial protein synthesis by tetracyclines. Messenger RNA (mRNA) attaches to the 30S subunit of bacterial ribosomal RNA. The P (peptidyl) site of the 50S ribosomal RNA subunit contains the nascent polypeptide chain; normally, the aminoacyl tRNA charged with the next amino acid (aa) to be added to the chain moves into the A (acceptor) site, with complementary base pairing between the anticodon sequence of tRNA and the codon sequence of mRNA. Tetracyclines inhibit bacterial protein synthesis by binding to the 30S subunit and blocking tRNA binding to the A site.
FIGURE 41-2 Inhibition of bacterial protein synthesis by chloramphenicol. Chloramphenicol binds to the 50S ribosomal subunit at the peptidyltransferase site and inhibits the transpeptidation reaction. Chloramphenicol binds to the 50S ribosomal subunit near the site of action of clindamycin and the macrolide antibiotics. These agents interfere with the binding of chloramphenicol and thus may interfere with each other’s actions if given concurrently. See Figure 41-1 and its legend for additional information.
FIGURE 41-3 Inhibition of bacterial protein synthesis by the macrolide antibiotics erythromycin, clarithromycin, and azithromycin. Macrolide antibiotics are bacteriostatic agents that inhibit protein synthesis by binding reversibly to the 50S ribosomal subunits of sensitive organisms. Erythromycin appears to inhibit the translocation step such that the nascent peptide chain temporarily residing at the A site of the transferase reaction fails to move to the P, or donor, site. Alternatively, macrolides may bind and cause a conformational change that terminates protein synthesis by indirectly interfering with transpeptidation and translocation. See Figure 41-1 and its legend for additional information.
A 56-year-old man has developed a community-acquired skin infection that is shown to be methicillin-resistant S. aureus. An infectious disease consultant recommends initial treatment with doxycycline.
a. Why doxycycline?
Tetracyclines, doxycycline, and minocycline have generally retained excellent levels of activity against staphylococci, including methicillin-resistant Staphylococcus aureus (MRSA). Community-acquired strains of MRSA often are susceptible to tetracycline, doxycycline, or minocycline.
b. What is the mechanism of action of doxycycline?
Tetracyclines and glycylcyclines inhibit bacterial protein synthesis by binding to the 30S bacterial ribosome and preventing access of aminoacyl tRNA to the acceptor site on the mRNA-ribosome complex (see Figure 41-1).
c. Another consultant has recommended chloramphenicol. Is this a good choice?
Chloramphenicol use should be limited to infections for which the benefits of the drug outweigh the risks of the potential toxicities. In this case, the benefits do not outweigh the risks.
Chloramphenicol is potentially toxic to the bone marrow in two ways: (1) a dose-related toxicity that presents as anemia, leukopenia, or thrombocytopenia, and (2) an idiosyncratic response manifested by aplastic anemia. This latter response may be fatal if the bone marrow aplasia is complete. The risk of aplastic anemia does not contraindicate the use of chloramphenicol in situations in which it may be lifesaving. However, the drug should never be used in undefined situations or in diseases that are readily, safely, and effectively treated with other less toxic antibiotics.
A 23-year-old woman college student is given a prescription for clarithromycin for a Haemophilus influenzae infection.
a. What is the mechanism of action of clarithromycin and how does it differ from that of tetracycline and chloramphenicol?
Clarithromycin acts by binding to the bacterial 50S ribosome (see Figure 41-3) to inhibit bacterial protein synthesis. This is the same site of action for chloramphenicol and clindamycin and these agents (macrolides, clindamycin, and chloramphenicol) may interfere with each other’s actions if given concurrently. Tetracycline binds to the 30S subunit of the bacterial ribosome.
b. What atypical infectious disease is commonly treated with clarithromycin?
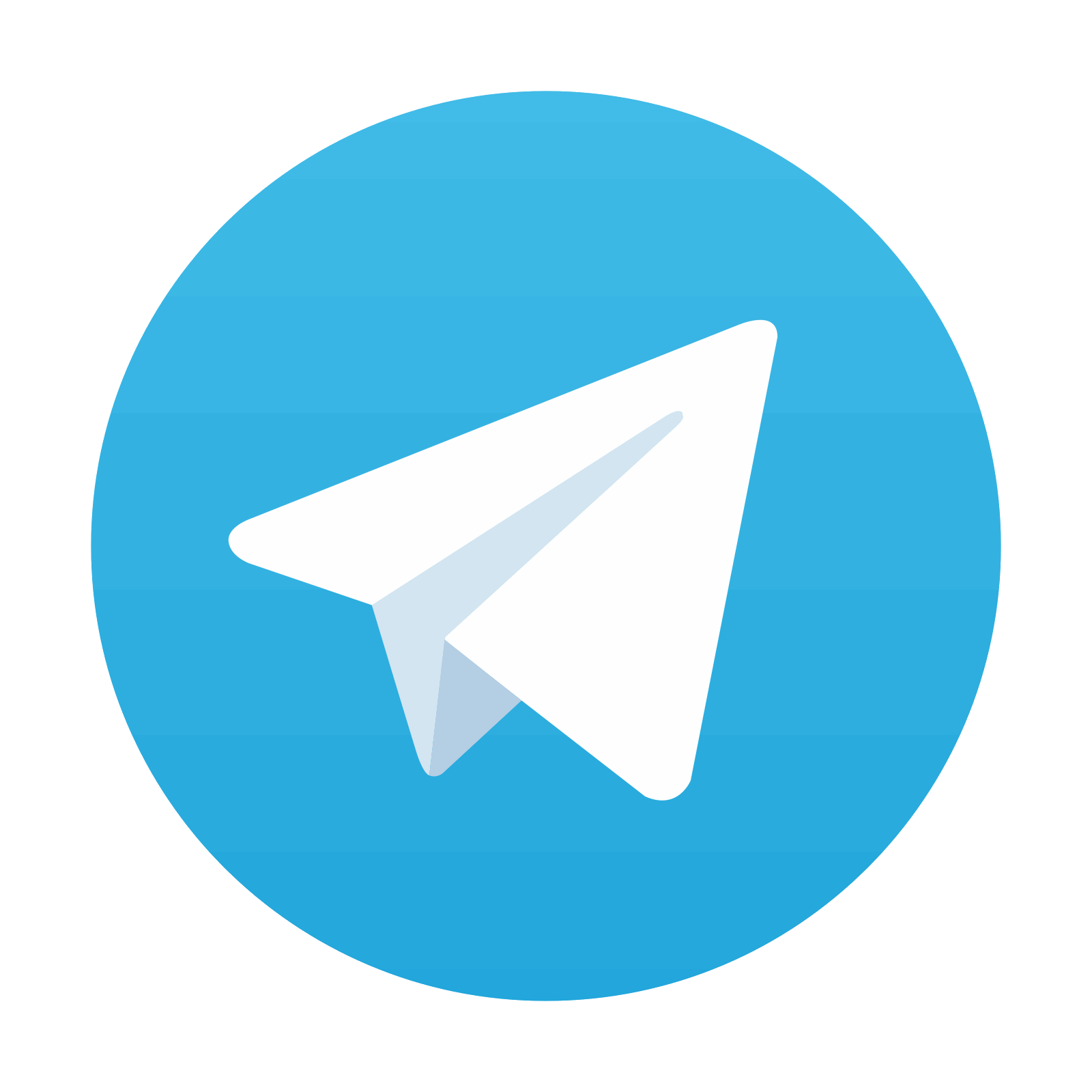
Stay updated, free articles. Join our Telegram channel

Full access? Get Clinical Tree
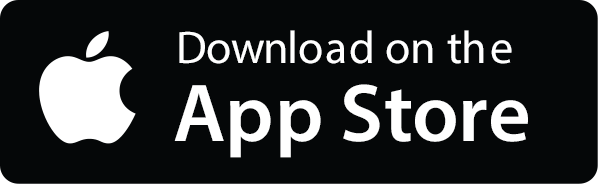
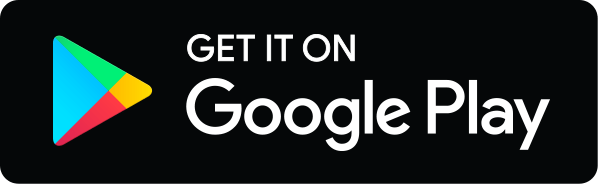