22
Oxidation of Fatty Acids: Ketogenesis
Kathleen M. Botham, PhD, DSc & Peter A. Mayes, PhD, DSc
OBJECTIVES
After studying this chapter, you should be able to:
Describe the processes by which fatty acids are transported in the blood and activated and transported into the matrix of the mitochondria for breakdown to obtain energy.
Outline the β-oxidation pathway by which fatty acids are metabolized to acetyl-CoA and explain how this leads to the production of large quantities of ATP from the reducing equivalents produced during β-oxidation and further metabolism of the acetyl-CoA via the citric acid cycle.
Identify the three compounds termed “ketone bodies” and describe the reactions by which they are formed in liver mitochondria.
Appreciate that ketone bodies are important fuels for extrahepatic tissues and indicate the conditions in which their synthesis and use are favored.
Indicate the three stages in the metabolism of fatty acids where ketogenesis is regulated.
Understand that overproduction of ketone bodies leads to ketosis and, if prolonged, ketoacidosis, and identify pathological conditions when this occurs.
Give examples of diseases associated with impaired fatty acid oxidation.
BIOMEDICAL IMPORTANCE
Although fatty acids are broken down by oxidation to acetyl-CoA and also synthesized from acetyl-CoA, fatty acid oxidation is not the simple reverse of fatty acid biosynthesis but an entirely different process taking place in a separate compartment of the cell. The separation of fatty acid oxidation in mitochondria from biosynthesis in the cytosol allows each process to be individually controlled and integrated with tissue requirements. Each step in fatty acid oxidation involves acyl-CoA derivatives, is catalyzed by separate enzymes, utilizes NAD+ and FAD as coenzymes, and generates ATP. It is an aerobic process, requiring the presence of oxygen.
Increased fatty acid oxidation is a characteristic of starvation and of diabetes mellitus, and leads to ketone body production by the liver (ketosis). Ketone bodies are acidic and when produced in excess over long periods, as in diabetes, cause ketoacidosis, which is ultimately fatal. Because gluconeogenesis is dependent upon fatty acid oxidation, any impairment in fatty acid oxidation leads to hypoglycemia. This occurs in various states of carnitine deficiency or deficiency of essential enzymes in fatty acid oxidation, for example, carnitine palmitoyltransferase, or inhibition of fatty acid oxidation by poisons, for example, hypoglycin.
OXIDATION OF FATTY ACIDS OCCURS IN MITOCHONDRIA
Fatty Acids Are Transported in the Blood as Free Fatty Acids
Free fatty acids (FFA)—also called unesterified (UFA) or non-esterified (NEFA) fatty acids—are fatty acids that are in the unesterified state. In plasma, longer chain FFA are combined with albumin, and in the cell they are attached to a fatty acid binding protein, so that in fact they are never really “free.” Shorter chain fatty acids are more water-soluble and exist as the unionized acid or as a fatty acid anion.
Fatty Acids Are Activated Before Being Catabolized
Fatty acids must first be converted to an active intermediate before they can be catabolized. This is the only step in the complete degradation of a fatty acid that requires energy from ATP. In the presence of ATP and coenzyme A, the enzyme acyl-CoA synthetase (thiokinase) catalyzes the conversion of a fatty acid (or FFA) to an “active fatty acid” or acyl-CoA, which uses one high-energy phosphate with the formation of AMP and PPi (Figure 22–1). The PPi. is hydrolyzed by inorganic pyrophosphatase with the loss of a further high-energy phosphate, ensuring that the overall reaction goes to completion. Acyl-CoA synthetases are found in the endoplasmic reticulum, peroxisomes, and inside and on the outer membrane of mitochondria.
FIGURE 22–1 Role of carnitine in the transport of long-chain fatty acids through the inner mitochondrial membrane. Long-chain acyl-CoA cannot pass through the inner mitochondrial membrane, but its metabolic product, acylcarnitine, can.
Long-Chain Fatty Acids Penetrate the Inner Mitochondrial Membrane as Carnitine Derivatives
Carnitine (β-hydroxy-γ-trimethylammonium butyrate), (CH3)3N+—CH2—CH(OH)—CH2—COO–, is widely distributed and is particularly abundant in muscle. Long-chain acyl-CoA (or FFA) cannot penetrate the inner membrane of mitochondria. In the presence of carnitine, however, carnitine palmitoyltransferase-I, located in the outer mitochondrial membrane, converts long-chain acyl-CoA to acylcarnitine, which is able to penetrate the inner membrane and gain access to the β-oxidation system of enzymes (Figure 22–1). Carnitine-acylcarnitine translocase acts as an inner membrane exchange transporter. Acylcarnitine is transported in, coupled with the transport out of one molecule of carnitine. The acylcarnitine then reacts with CoA, catalyzed by carnitine palmitoyltransferase-II, located on the inside of the inner membrane, reforming acyl-CoA in the mitochondrial matrix, and carnitine is liberated.
β-OXIDATION OF FATTY ACIDS INVOLVES SUCCESSIVE CLEAVAGE WITH RELEASE OF ACETYL-CoA
In β-oxidation (Figure 22–2), two carbons at a time are cleaved from acyl-CoA molecules, starting at the carboxyl end. The chain is broken between the α(2)- and β(3)-carbon atoms—hence the name β-oxidation. The two-carbon units formed are acetyl-CoA; thus, palmitoyl-CoA forms eight acetyl-CoA molecules.
FIGURE 22–2 Overview of β-oxidation of fatty acids.
The Cyclic Reaction Sequence Generates FADH2 & NADH
Several enzymes, known collectively as “fatty acid oxidase,” are found in the mitochondrial matrix or inner membrane adjacent to the respiratory chain. These catalyze the oxidation of acyl-CoA to acetyl-CoA, the system being coupled with the phosphorylation of ADP to ATP (Figure 22–3).
FIGURE 22–3 β-Oxidation of fatty acids. Long-chain acyl-CoA is cycled through reactions –
, acetyl-CoA being split off, each cycle, by thiolase (reaction
). When the acyl radical is only four carbon atoms in length, two acetyl-CoA molecules are formed in reaction
.
The first step is the removal of two hydrogen atoms from the 2(α)- and 3(β)-carbon atoms, catalyzed by acyl-CoA dehydrogenase and requiring FAD. This results in the formation of Δ2-trans-enoyl-CoA and FADH2 The reoxidation of FADH2 by the respiratory chain requires the mediation of another flavoprotein, termed electron-transferring flavoprotein (Chapter 12). Water is added to saturate the double bond and form 3-hydroxyacyl-CoA, catalyzed by Δ2-enoyl-CoA hydratase. The 3-hydroxy derivative undergoes further dehydrogenation on the 3-carbon catalyzed by L(+)-3-hydroxyacyl-CoA dehydrogenase to form the corresponding 3-ketoacyl-CoA compound. In this case, NAD+ is the coenzyme involved. Finally, 3-ketoacyl-CoA is split at the 2,3-position by thiolase (3-ketoacyl-CoA-thiolase), forming acetyl-CoA and a new acyl-CoA two carbons shorter than the original acyl-CoA molecule. The acyl-CoA formed in the cleavage reaction reenters the oxidative pathway at reaction 2 (Figure 22–3). In this way, a long-chain fatty acid may be degraded completely to acetyl-CoA (C2 units). Since acetyl-CoA can be oxidized to CO2 and water via the citric acid cycle (which is also found within the mitochondria), the complete oxidation of fatty acids is achieved.
Oxidation of a Fatty Acid with an Odd Number of Carbon Atoms Yields Acetyl-CoA Plus a Molecule of Propionyl-CoA
Fatty acids with an odd number of carbon atoms are oxidized by the pathway of β-oxidation, producing acetyl-CoA, until a three-carbon (propionyl-CoA) residue remains. This compound is converted to succinyl-CoA, a constituent of the citric acid cycle (Figure 20–2
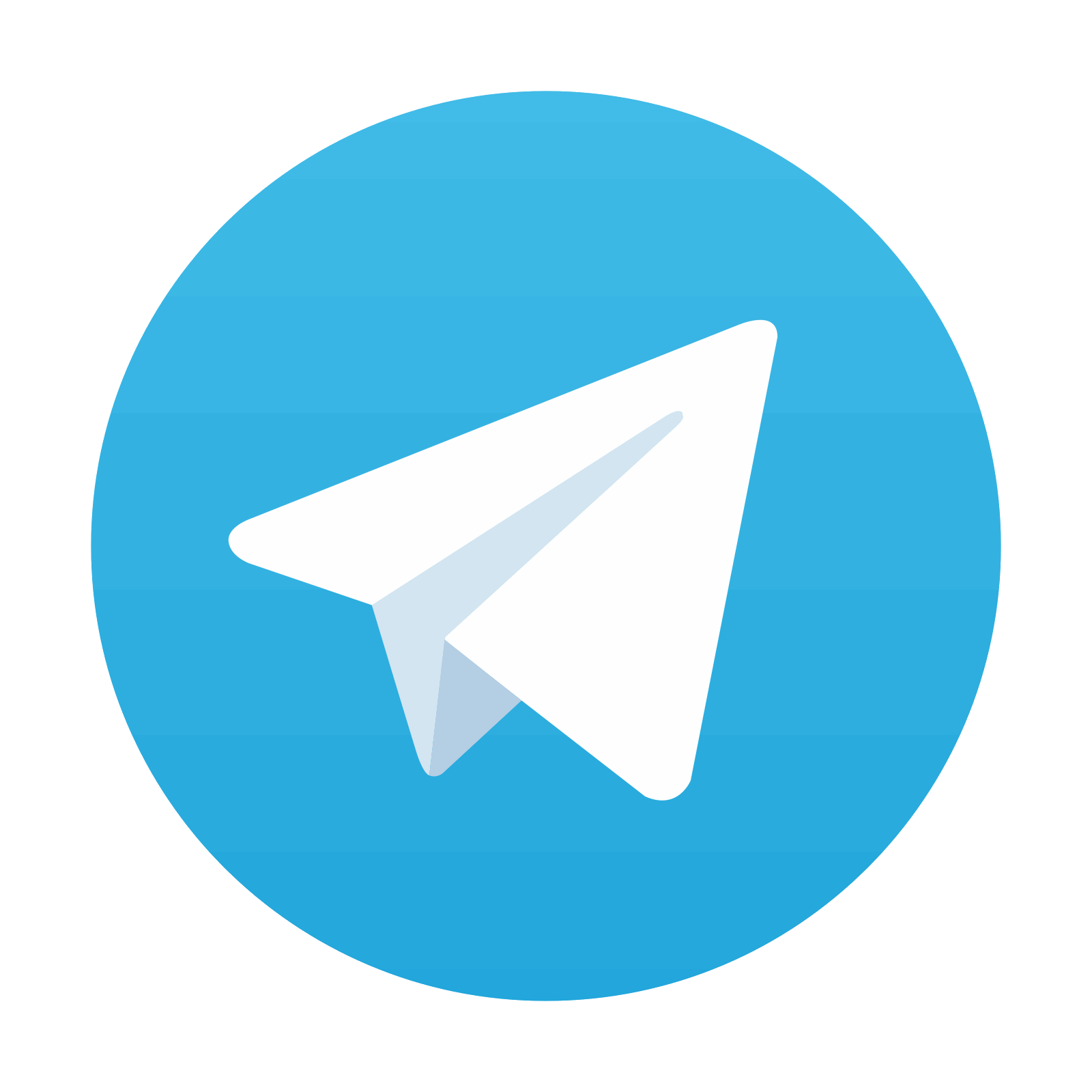
Stay updated, free articles. Join our Telegram channel

Full access? Get Clinical Tree
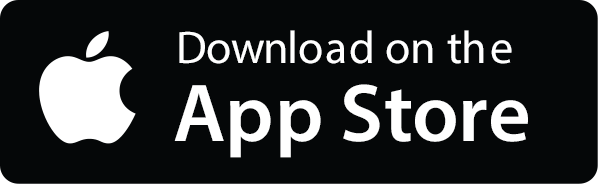
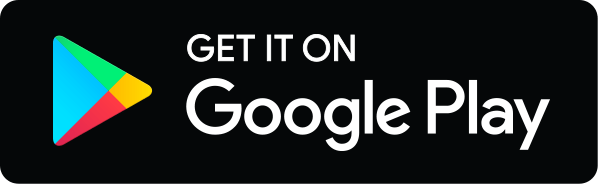