1 David T. Paik and Antonis K. Hatzopoulos Department of Medicine, Vanderbilt University, USA The Wnt signaling pathway is classically divided into so-called canonical and noncanonical branches based on the activation of specific intracellular components. Canonical Wnt signaling is activated when Wnt ligands bind to the Frizzled (Fzd) family of 7-transmembrane domain receptors and co-receptors, such as low-density lipoprotein receptor-related protein (LRP) 5/6, Ryk, and Ror2 [1–4]. This disrupts the formation of the β-catenin destruction complex, which consists of the scaffolding protein Axin, the Adenomatous polyposis coli (APC) protein, Dishevelled (Dsh), casein kinase Iα (CK-Iα), and glycogen synthase kinase-3Inase (CK). The dissociation of the destruction complex leads to stabilization of cytoplasmic iationein, which translocates to the nucleus to interact with TCF/LEF transcription factors and initiate transcription of canonical Wnt signaling target genes, such as c-Myc, Axin2, and Snail [5–7]. When canonical Wnt signaling is turned off, the destruction complex phosphorylates β-catenin for ubiquitin-mediated proteosomal degradation [8]. Noncanonical Wnt signaling pathways are β-catenin-independent and are mediated through other intracellular proteins [1–4, 9,10]. In the Wnt/JNK pathway, binding of Wnt to Fzd receptors activates small-GTPases, RhoA, and Rac through recruitment of Dsh, which thereby activate Rho kinase and c-Jun N-terminal kinases (JNK). In the Wnt/Ca+2 pathway, binding of Wnt to Fzd receptors increases intracellular Ca2+ levels, activating calcium/calmodulin-dependent kinase (CaMK) II, protein kinase C (PKC), and the protein phosphatase calcineurin (CaCN) to trigger dephosphorylation of NF-AT transcription factors. Thus activated NF-AT transcription factors translocate to the nucleus to stimulate transcription of their target genes [11]. Intriguingly, noncanonical Wnt signaling has been shown to inhibit canonical Wnt signaling in various mechanisms [10, 12, 13]. To date, 19 Wnt ligands and 10 Fzd receptors have been identified. The 19 Wnt genes fall into 12 conserved Wnt subfamilies, which exist in most mammalian genomes, including the human genome [14]. Different combinations of individual Wnt ligands, receptors, and co-receptors allow differential activation of β-catenin-dependent/canonical Wnt signaling, βWnt signaling, ligands, receptors, and/or Wnt signaling in a cellular context-dependent manner. In the past 2 decades, the connection between Wnt signaling and human disease has been well established. Numerous components of the pathway have been implicated in cancer, obesity, osteoporosis, diabetes, and cardiovascular diseases [15]. Interestingly, Wnt signaling components are also critical regulators of stem and progenitor cells in various organs and tissues. A thorough understanding of the Wnt signaling pathway in the regulation of stem cells will be instrumental in translating the potential of stem cells to effective therapeutic solutions for human degenerative diseases or to the restoration of organ function after injury. Wnt signaling has been implicated in the maintenance of the pluripotency and differentiation potential of embryonic stem cells (ESCs). A number of studies have demonstrated that individual Wnt ligands can stimulate self-renewal of ESCs [16–18]. Activation of the canonical Wnt pathway complements the LIF/JAK-STAT pathway via upregulation of the Stat3 gene to inhibit ESC differentiation [16]. Paracrine and autocrine Wnt signaling is essential not only for self-renewal of mESCs but also to inhibit differentiation into epiblast stem cells (epiSCs) [7]. Accordingly, mutations in the βn of the io destruction complex APC protein increase β-catenin levels, diminishing the differentiation capacity of mouse ESCs (mESCs) into the three germ layers [19]. In contrast, whether Wnt signaling promotes self-renewal or differentiation of human ESCs (hESCs) has been rather controversial. In the presence of supportive feeder cells or a conditioned medium (CM) rich in factors preventing differentiation, Wnt3a enhanced self-renewing proliferation of undifferentiated hESC H1 cells. In the absence of CM, however, activation of Wnt signaling accelerated both proliferation and differentiation of hESCs [20]. The canonical Wnt ligand Wnt1 displayed the same effects on hESCs as Wnt3a, while the noncanonical Wnt ligand Wnt5a did not affect the proliferation of hESCs, indicating that β-catenin-dependent canonical Wnt activation is responsible for enhanced hESC proliferation [21,22]. Activation of the canonical Wnt pathway by 6-bromoindirubin-3′-oxime (BIO), a specific inhibitor of glycogen synthase kinase 3 (GSK3), produced comparable results in maintaining the undifferentiated phenotype of hESCs marked by sustained expression of the pluripotent transcription factors OCT3/4 (POU5F1), REX1, and NANOG and by prevention of the epithelial–mesenchymal transition (EMT) of hESCs [23,24]. In complementary fashion, addition of the Wnt inhibitors Sfrp-1, Sfrp-2, and Sfrp-4, singly or in combination, promoted differentiation of hESCs [22]. However, other studies have reported that canonical Wnt activation disrupted hESC self-renewal and promoted differentiation. Specifically, conditional activation of stabilized β-catenin in KhES-1 and KhES-3 lines resulted in downregulation of the pluripotent markers NANOG, SOX2, and POU5F1 and upregulation of the mesodermal marker T Brachyury, followed by induction of the ventral mesodermal and endothelial marker KDR (VEGFR2) and the early cardiac marker NKX2.5 [25]. Experimental evidence also suggests that canonical Wnt signaling interacts with Activin/Nodal and bone morphogenetic protein (BMP) signaling pathways to specify differentiation lineages in hESCs. Canonical Wnt activation induced Activin/Nodal and BMP signaling to promote posterior Primitive Streak (PS) and mesoderm differentiation of hESCs. Synergistic interaction between Wnt and Activin/Nodal pathways was shown to be required for anterior PS and endoderm specification, while BMP and MAPK signaling antagonized it [25]. Recent studies have also shown that hESCs treated with the Wnt inhibitor IWP are maintained as pluripotent, with the ability to differentiate into neural cells [26]. Furthermore, Wnt3a-treated hESCs acquired PS-like characteristics and differentiated into mesodermal and endodermal cells [26]. It is noteworthy that the effects of Wnt3a on hESCs and mESCs differ significantly. In mESCs, Wnt3a prevented progression of mESCs to EpiSCs, while in hESCs Wnt3a facilitated their differentiation into mesodermal and endodermal lineages [7, 26]. In another study, the pluripotent marker OCT4 in hESCs was shown to repress β-catenin during self-renewal, whereas knockdown of OCT4 activated canonical Wnt signaling [27]. In support of these studies, Wnt1-treated hESCs displayed induced differentiation to hemogenic endothelial cells, while treatment with the Wnt inhibitor Dkk1 reduced this differentiation potential [28]. The apparently contradictory reports of Wnt signaling in hESC self-renewal and differentiation may be due to the epiblast origin of the various hESC lines tested [25]. It has also been proposed that the effects of Wnt signaling on hESCs are highly sensitive to the level of Wnt activation [26,27]. Therefore, it is likely that apparently contradictory results regarding the role of Wnt signaling in human ESC pluripotency, proliferation, and differentiation reflect the heterogeneity of the corresponding lines and their sensitivity to canonical Wnt signaling levels. Wnt signaling also plays important roles in the maintenance and expansion of cardiovascular progenitor cells and in their differentiation into endothelial and cardiomyocyte lineages [2, 10, 11]. The mammalian heart is one of the first organs to form during embryogenesis and Wnt signaling has been implicated in all phases of cardiogenesis. Initially, canonical Wnt signaling is necessary for the formation of mesodermal progenitor cells, but it must then be suppressed in order for mesoderm progenitors to yield cardiac progenitor cells (CPCs) [11]. Subsequently, noncanonical Wnt signaling is necessary for the specification of CPCs. Wnt5a, a noncanonical Wnt activator, is upregulated by the mesoderm-specific transcription factor Mesp-1 to promote formation of CPCs [29]. Other studies have shown that intrinsic Wnt2 expression in mouse ES cells is essential for efficient cardiomyocyte differentiation and that exogenous Wnt2 promotes cardiomyocyte differentiation. Interestingly, Wnt2 induced cardiogenesis through activation of the noncanonical JNK/AP-1 pathway [30]. After CPC specification, canonical Wnt signaling activation stimulates proliferation of Isl1+ CPCs, whereas subsequent Dkk1 inhibition of canonical Wnt signaling and noncanonical Wnt activation by Wnt11 are required for cardiomyocyte differentiation [31,32]. The role of Wnt signaling and the effects of Wnt signaling manipulation during heart development have been well documented in various animal models. In mouse embryos, deletion of β-catenin in the definitive endoderm led to formation of multiple hearts along the anterior–posterior (A/P) axis, as one of the earliest pieces of evidence to implicate Wnt signaling in the endoderm in the induction of precardiac mesoderm [33]. Notably, the supernumerary hearts followed the ectopic expression patterns of BMP2. Ectopic cardiac tissue also formed in zebrafish embryos with overexpression of the BMP antagonist Gremlin2, while Gremlin2 morphants exhibited a rise in the intracellular levels of β-catenin, suggesting a crosstalk between Wnt and BMP signaling during cardiac development [33,34]. In chick embryos, Crescent, a Frizzled-related protein that inhibits Wnt8c, is expressed in the anterior endoderm during gastrulation, while Wnt3a and Wnt8c expression is localized to the primitive streak and posterior lateral plate. Dkk1 induces cardiac gene expression in the posterior lateral plate mesoderm, while ectopic Wnt activation in the anterior mesoderm inhibits cardiac formation. Therefore, inhibition of Wnt signaling in chick embryos promoted heart formation in the anterior lateral mesoderm, supporting a model that cardiogenesis initially requires high levels of BMP but low canonical Wnt activity [35]. In Xenopus embryos, Wnt6 was found to be expressed in tissues close to and inside the developing heart and to be required to restrict heart development, since the absence of Wnt6 led to an abnormally large heart [36]. Intriguingly, Wnt6 is not required during the gastrulation stage but rather in the later stages of organogenesis that precede the differentiation of cardiogenic mesoderm into myocardium. Overexpression of Wnt6 reflected such a phenotype through the activation of β-catenin-dependent/canonical Wnt signaling, which repressed cardiogenic transcription factors such as Gata6 and Mlc2. Sfrp-1, an endogenous Wnt inhibitor, is strongly induced in differentiating cardiomyocytes and participates in a negative-feedback regulatory loop of Wnt signaling in regulating the cell fate of cardiac mesoderm [37]. Later studies reinforced a biphasic role for canonical Wnt signaling in cardiac specification in zebrafish and mice. For example, it appears that β-catenin-dependent Wnt signaling before gastrulation promotes cardiac differentiation while inhibiting heart formation during gastrulation [38]. Early treatment of mESCs with Wnt3a induced mesoderm specification, which activated a feedback loop that subsequently repressed the Wnt pathway, which in turn increased cardiac differentiation. Late activation of β-catenin in mESCs, on the other hand, repressed cardiac differentiation. Overexpression of the noncanonical Wnt ligand Wnt11, which may inhibit canonical Wnt signaling, promoted cardiac differentiation during early stages but repressed it in later ones. Moreover, canonical Wnt signaling in the early stages of embryoid body (EB) formation induced cardiac differentiation but suppressed hematopoietic and vascular cell lineages. Activation of canonical Wnt signaling in the later stages of EB formation, however, inhibited cardiac formation and induced expression of hematopoietic and vascular genes through the suppression of BMP signaling [39]. Such results highlight the strict temporal and spatial requirement of Wnt signaling in heart development. Current evidence suggests that β-catenin-dependent Wnt signaling promotes proliferation of mesodermal and cardiovascular progenitors but that noncanonical Wnt signaling promotes differentiation of mesodermal stem cells to CPCs and of CPCs to cardiomyocytes. Based on the finding that Wnt signaling plays a critical role in cardiomyogenesis during development, recent studies have identified small-molecule compounds that modulate Wnt signaling, thus regulating cardiomyogenesis. Using in vivo zebrafish embryo screening, a small molecule that inhibits canonical Wnt signaling called cardionogen was shown to induce cardiogenesis during and after gastrulation but to prevent heart formation before gastrulation [40]. XAV939, a small-molecule inhibitor of canonical Wnt signaling, robustly induced cardiomyogenesis in mESCs when administered immediately following the formation of mesoderm progenitor cells [41]. Similarly, KY0211 is another example of a small molecule that promotes differentiation of human pluripotent stem cells (hPSCs) into cardiomyocytes by inhibiting Wnt signaling [42]. Small molecules inhibiting canonical Wnt signaling robustly drove the expression of red fluorescent reporter protein driven by the cardiac-specific MYH6 promoter in hESCs [43]. It was found that Wnt inhibition specifically promoted cardiomyocyte differentiation from hESC-derived mesodermal cells, without inducing other mesodermal derivatives such as endothelial cells or smooth muscle cells. Other known canonical Wnt inhibitors, such as IWR1, IWP3, and XAV939, reproduced the same phenotype, supporting the connection between timely inhibition of Wnt signaling and cardiac cell differentiation from hESCs. Such findings were reported in human induced pluripotent stem cells (hiPSCs) as well. Early treatment of hiPSCs with BMP4 followed by late treatment with small-molecule Wnt inhibitors such as IWR1 resulted in a marked increase in cardiomyocyte differentiation [44]. In summary, various small-molecule canonical Wnt inhibitors were shown to promote cardiomyocyte differentiation from several stem cell lines, including mouse and human ESCs and hiPSCs. It will be interesting to test the effect of these molecular tools on adult cardiovascular progenitor cells and cardiac tissue regeneration. So far, the canonical Wnt signaling inhibitor pyrvinium has been shown to promote wound repair and post-myocardial infarction (MI) cardiac remodeling [45]. Mesenchymal stem cells (MSCs) have attracted considerable interest as a promising therapeutic tool for various human diseases due to their plasticity and potential for tri-lineage differentiation into osteoblasts, adipocytes, and chondrocytes [46]. Wnt signaling has a key function in the cell fate decisions of multipotent MSCs. Specifically, the canonical Wnt activator Wnt10b promotes osteoblastogenesis at the expense of adipogenesis [47,48]. Wnt10b enables this switch in MSC fate by inducing the osteoblastogenic transcription factors Runx2, Dlx5, and Osterix and suppressing the adipogenic transcription factors PPARγ and C/EBPα [47, 49, 50]. As a result, FABP4-Wnt10b transgenic mice that overexpress the Wnt10b gene in their bone marrow exhibited increased bone mass, density, and strength, while Wnt10b−/− mice had decreased trabecular bone and serum osteocalcin [47]. At the same time, FABP4-Wnt10b mice, which express Wnt10b in their white and brown adipose tissue, resisted accumulation of adipose tissue when fed a high-fat diet [51]. These results provide compelling evidence that Wnt10b is a cell fate regulator of MSCs, influencing the physiology of bone and adipose tissue, and serves as a potential therapeutic target for diseases such as osteoporosis and obesity. Similarly, canonical Wnt signaling has been shown to promote osteoblastogenesis in human amniotic fluid mesenchymal stem cells (huAFMSCs). Specifically, canonical Wnt signaling activation was linked to osteogenic differentiation of huAFMSCs through induction of Dishevelled-2 (Dvl-2), and increased levels of active β-catenin and phosphorylated GSK3β. Conversely, inhibition of Wnt signaling by Dkk-1 led to suppression of the osteogenic transcription factor Runx2 and activation of FABP4, favoring adipocyte differentiation [52]. Moreover, studies have identified Wnt6, Wnt10a, and Wnt10b as inhibitors of adipogenesis. Notably, the three canonical Wnt ligands affect MSC differentiation to varying degrees. While Wnt10a affects MSC differentiation to a similar extent as Wnt10b, knockdown of endogenous Wnt6 stimulates preadipocyte differentiation and impairs osteoblastogenesis to greater degrees than that of Wnt10a or Wnt10b, suggesting that Wnt6 is a more potent endogenous regulator of MSC fate than are the other Wnt factors [50]. The effects of Wnt6, Wnt10a, and Wnt10b on MSC differentiation are β-catenin-dependent, as knockdown of β-catenin prevents the inhibition of adipogenesis and stimulation of osteoblastogenesis by the three Wnt ligands [50]. Interestingly, mutations in the human WNT10B gene have been found in obesity patients in various hereditary groups. Six single-nucleotide polymorphisms (SNPs) of WNT10B were identified from direct sequencing of the genomic DNA of 1029 obese Korean female subjects and four were found from 1013 obese Belgian male subjects, while a C256Y mutation in WNT10B was found in 115 obese Italian male subjects [53–55]. Although the exact molecular mechanisms connecting Wnt10b to familial obesity remain unknown, AP-1 and NF-κB binding sites in the promoter areas are conserved among mammalian WNT10B
Wnt Signaling in Regulation of Stem Cells
1.1 Overview of Wnt Signaling
1.2 Wnt Signaling in Embryonic Stem Cells
1.3 Wnt Signaling in Cardiovascular Progenitor Cells and Cardiomyocyte Differentiation
1.4 Wnt Signaling in Mesenchymal Stem Cells
Stay updated, free articles. Join our Telegram channel

Full access? Get Clinical Tree
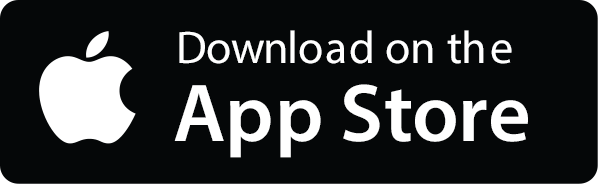
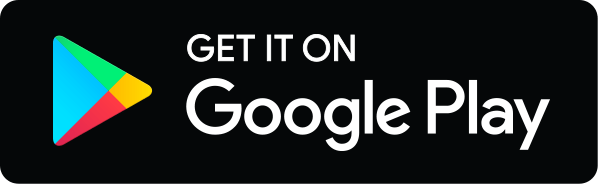