Chapter 29 Vitamins and Minerals
Table 29.1 summarizes the DRIs for adult men and women. Actual requirements depend also on age, body weight, diet, and physiological status. Increases in dietary intake of many nutrients are recommended during pregnancy and lactation.
Table 29.1 Dietary Reference Intakes for Vitamins and Minerals
Nutrient | 70-kg Man | 55-kg Woman |
---|---|---|
Water-Soluble Vitamins | ||
Niacin | 16 mg | 14 mg |
Riboflavin | 1.3 mg | 1.1 mg |
Thiamin | 1.2 mg | 1.1 mg |
Pyridoxine (B6) | 1.3 mg | 1.3 mg |
Pantothenic acid | 5 mg | 5 mg |
Biotin | 30 μg | 30 μg |
Ascorbic acid | 90 mg | 75 mg |
Folic acid | 400 μg | 400 μg |
Cobalamin (B12) | 2.4 μg | 2.4 μg |
Fat-Soluble Vitamins | ||
Vitamin A | 900 μg | 700 μg |
Vitamin D | 5 μg | 5 μg |
Vitamin K | 120 μg | 90 μg |
Vitamin E | 15 mg | 15 mg |
Macrominerals | ||
Sodium | 1.5 g | 1.5 g |
Potassium | 4.7 g | 4.7 g |
Calcium | 1 g | 1 g |
Magnesium | 420 mg | 320 mg |
Chloride | 2.3 g | 2.3 g |
Phosphate | 700 mg | 700 mg |
Microminerals | ||
Iron | 8 mg | 18 mg |
Copper | 900 μg | 900 μg |
Zinc | 11 mg | 8 mg |
Manganese | 2.3 mg | 1.8 mg |
Molybdenum | 45 μg | 45 μg |
Chromium | 35 μg | 25 μg |
Selenium | 55 μg | 55 μg |
Iodide | 150 μg | 150 μg |
Fluoride | 4 mg | 3 mg |
Riboflavin is a precursor of flavin mononucleotide and flavin adenine dinucleotide
Riboflavin (vitamin B2) consists of a dimethylisoalloxazine ring covalently bound to the sugar alcohol ribitol (Fig. 29.1). Riboflavin is the dietary precursor of flavin adenine dinucleotide (FAD) and flavin mononucleotide (FMN), the prosthetic groups of the flavoproteins (from Latin flavus meaning “yellow”). Both riboflavin and the flavin coenzymes are yellow in their reduced form, with an absorption band at 450 nm. Although riboflavin and its derivatives are heat stable, they are rapidly degraded to inactive products on exposure to visible light. Therefore riboflavin deficiency can occur in infants receiving phototherapy for hyperbilirubinemia (see Chapter 27), when riboflavin as well as bilirubin is destroyed by light in the skin.
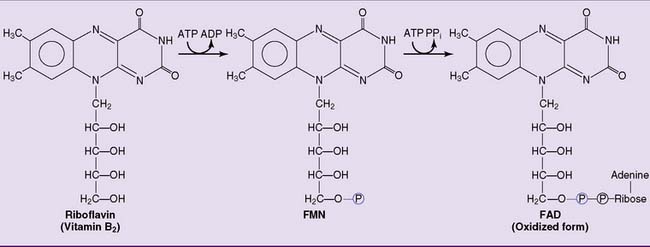
Figure 29.1 Synthesis of flavin mononucleotide (FMN) and flavin adenine dinucleotide (FAD) from dietary riboflavin.
Dietary status can be assessed by fluorometric or microbiological determination of urinary riboflavin. Alternatively, the activity of erythrocyte glutathione reductase (see Chapter 22) is determined in freshly lysed red blood cells before and after the addition of its coenzyme FAD. In riboflavin deficiency, the apoenzyme is not completely saturated with its coenzyme; therefore, the enzymatic activity is increased by added FAD.
Niacin is a precursor of NAD and NADP
In both the human body and dietary sources, niacin is present as a constituent of NAD and NADP. The dietary coenzymes are hydrolyzed in the gastrointestinal tract, and free nicotinic acid and nicotinamide are absorbed in the small intestine. After their transport to the tissues, the vitamin forms are incorporated into the coenzymes (Fig. 29.2). Excess niacin is readily excreted by the kidneys.
Thiamin deficiency causes weakness and amnesia
About 30 mg of the vitamin is present in the body, 80% of this in the form of TPP.
The TPP-dependent reactions are aldehyde transfers in which the aldehyde is bound covalently to one of the carbons in the thiazole (sulfur-and-nitrogen) ring of the coenzyme. One reaction type, the oxidative decarboxylation of α-ketoacids, is catalyzed by mitochondrial multienzyme complexes. Pyruvate dehydrogenase, α-ketoglutarate dehydrogenase (see Chapter 21), branched-chain α-ketoacid dehydrogenase, and α-ketobutyrate dehydrogenase (see Chapter 26) all use the same thiamin-dependent catalytic mechanism.
A different reaction type is encountered in the cytoplasmic transketolase reaction (see Chapter 22) in which TPP transfers a glycolaldehyde from one monosaccharide to another. In general, the major catabolic, energy-producing pathways are most dependent on TPP.
Vitamin B6 plays a key role in amino acid metabolism
Vitamin B6 is the generic name for the dietary precursors of the coenzyme pyridoxal phosphate (PLP). They include pyridoxine, pyridoxal, and pyridoxamine as well as their phosphorylated derivatives (Fig. 29.3).
The phosphate is removed by intestinal alkaline phosphatase, and the dephosphorylated forms are absorbed. The total body content of PLP is only 25 mg in adults, and pyridoxal and PLP are the major circulating forms of the vitamin. Synthesis of the coenzyme form is described in Figure 29.4.
Several dozen enzymes of amino acid metabolism contain PLP as a tightly bound prosthetic group. In these reactions, the aldehyde group of PLP forms an aldimine derivative with the amino group of the amino acid. The aldimine is stabilized by an intramolecular hydrogen bond with the phenolic hydroxyl group (Fig. 29.5).
Liver, fish, whole grains, nuts, legumes, egg yolk, and yeast are good sources of vitamin B6. Serious deficiency is rare, but when it occurs it is characterized by peripheral neuropathy, stomatitis, glossitis, irritability, psychiatric symptoms, and, especially in children, epileptic seizures. Some of the neurological derangements may result from impaired activity of the PLP-dependent enzyme glutamate decarboxylase, which forms the inhibitory neurotransmitter γ-aminobutyric acid (GABA) (see Chapter 16).
Pantothenic acid is a building block of coenzyme A
Pantothenic acid consists of pantoic acid and β-alanine:
Pantothenic acid functions as a constituent of coenzyme A (CoA) and of the phosphopantetheine group in the fatty acid synthase complex (see Chapter 23). The structure and biosynthesis of CoA are summarized in Figure 29.6.
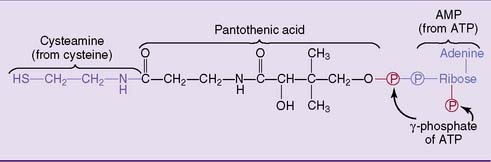
Figure 29.6 Structure of coenzyme A. Pantothenic acid is the only nutritionally essential component of this coenzyme.
Folic acid deficiency causes megaloblastic anemia
THF is a carrier of one-carbon units, which are bound to one or both of the nitrogen atoms N-5 and N-10 (Fig. 29.8):
CLINICAL EXAMPLE 29.4: Biotinidase Deficiency
The proteolytic degradation of biotin-containing enzymes, both in the intestinal lumen and in the tissues, produces the biotin-lysine conjugate biocytin (Fig. 29.7). Biotin is released from biocytin by biotinidase. Biotinidase deficiency causes nondietary biotin deficiency. Affected infants present with hypotonia, seizures, optic atrophy, dermatitis, and conjunctivitis. This condition can be cured easily with biotin supplements. Biotinidase deficiency is often included in newborn screening programs, along with other treatable congenital diseases. It can be diagnosed by enzyme assay in fresh serum or, as a screening test, on a strip of blood-soaked filter paper.
CLINICAL EXAMPLE 29.5: Sulfonamides
Unlike humans, most bacteria make their own folate from pteridine and PABA (Fig. 29.9). Therefore their growth can be inhibited with drugs that block folate synthesis. The sulfonamides are structural analogs of PABA that inhibit the synthesis of pteroic acid in bacteria. Although they do not kill the bacteria immediately, they prevent their growth. They are bacteriostatic, not bactericidal.
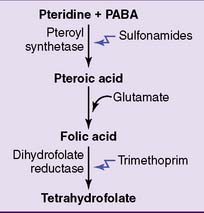
Figure 29.9 Pharmacological inhibition of tetrahydrofolate synthesis in bacteria. PABA, Para-aminobenzoic acid.