© Springer International Publishing Switzerland 2015
Mahdi Balali-Mood and Mohammad Abdollahi (eds.)Basic and Clinical Toxicology of Mustard Compounds10.1007/978-3-319-23874-6_1313. Verification of SM Exposure in Biological Samples
(1)
Bundeswehr Institute of Pharmacology and Toxicology, Neuherbergstraße 11, Munich, 80937, Germany
Abstract
Sulfur mustard (SM) is a potent vesicant chemical warfare agent. Use of such agents is considered as “crossing a red line”. Exposure to SM via inhalational, cutaneous and ocular route can result in a systemic uptake causing the formation of specific biomarkers that can be of use for verification. Comprehensive methods for a free of doubt verification in biological samples do exist that detect either remaining pure SM in the circulation and tissues, or rely on biomarkers resulting from SM hydrolysis, SM biotransformation products, SM protein adduct or SM DNA adduct formation.
This chapter provides an overview about existing biomarkers that indicate a SM exposure and analytical methods for their detection with special focus on the respective toxicokinetics. Intact SM in urine or blood can be analyzed by GC- or LC-MS methods in a short time frame after exposure. Specific ß-lyase metabolites and non-specific TDG have also been successfully determined by GC- or LC-MS methods. Several specific protein adducts with SM do occur, including albumin and hemoglobin, and are frequently used for verification purposes. Finally, SM-DNA adducts can be visualized with immunohistochemical methods or with evidentiary LC-MS based methods.
Keywords
Sulfur MustardVerificationMass spectrometryBiotransformation productsProtein adductsDNA adducts13.1 Introduction
Sulfur mustard is a potent vesicant chemical warfare agent. Exposure via inhalation, cutaneous and ocular route results in typical clinical symptoms (Kehe et al. 2009a, c). Systemic uptake is commonly observed and is primarily dependent on the exposure dose, but is independent of the exposure route. Already vapor exposure of the skin is sufficient to result in significant systemic uptake and the possibility of a reliable verification (Steinritz et al. 2015).
The use of chemical warfare agents is considered as “crossing a red line”. Thus, evidentiary, free of doubt analytical methods for verification are necessary. With regard to human exposures simply identifying the agent in environmental samples is insufficient as individual exposures cannot be circumstantiated. Therefore, robust analytical methods for the investigation of biological samples are required.
Different methods exist that detect either remaining pure SM in the circulation and tissues, or rely on biomarkers resulting from SM hydrolysis, SM biotransformation products, SM protein adduct or SM-DNA adduct formation. If biomarkers are to be used for forensic purposes, they need to meet a number of requirements with regard to lifetime, chemical stability and specificity (John et al. 2009). Thus a detailed knowledge is necessary to choose the most applicable biomarker and the correspondent analytical method with regard to sample type, and sampling time.
In addition to the verification of SM or SM metabolites in biological samples, a plethora of GC or LC-Ms based analytical methods for the determination of SM in almost all environmental matrices (air, water and soil) do exist.
13.2 Toxicokinetic
Pure SM not having contact to aqueous environments is considered as stable for days to weeks. However, in aqueous surroundings the highly reactive compound reveals a comparably short half-life of 4–8 min at 37 °C in vitro (Vycudilik 1987; Bartlett and Swain 1949). However, in vivo it was shown that distribution of the lipophilic SM into adipose tissue after systemic uptake can result in a depot of SM thus decreasing the hydrolysis rate (Drasch et al. 1987). Intact SM was detected in rat plasma up to 8 h after exposure (Maisonneuve et al. 1992, 1993). Nevertheless, a reliable analysis of pure SM is considered to be limited to a short time frame within the first hours after exposure thus sampling has to be realized as soon as possible. After sampling SM hydrolysis can be decreased by adding sodium chloride in excess to the sample and immediately storage at −20 °C or below.
The hydrolysis of SM is complex. After intraperitoneal or cutaneous administration of SM to rats ten different biotransformation products have been described in the urine (Black et al. 1992a, b) which could also be found in human samples after SM exposures (Black and Read 1995). Analytical methods have been developed for four out of this ten biotransformation products. However, only the ß-lyase biotransformation products can be used for a verification of SM exposure as other metabolites revealed little, but distinct endogenous levels in non-exposed individuals (Black and Read 1988). A recent report demonstrated that specific-lyase biotransformation products in plasma were found eightfold greater than lower limit of quantification on day 6 after an accidental exposure (Xu et al. 2014). Nevertheless, in vivo studies using rats and rabbits point to an extraction of more than 99 % of the total amount of ß-lyase products in the first week after exposure (Lin et al. 2014a).
A plethora of reports are available that have investigated the abundance of SM-protein adducts in vitro (Smith et al. 2008; Noort et al. 2002, 2008) and also in vivo (Benschop et al. 1997; Xu et al. 2014). However, although most reports have investigated the biological fate of these SM-protein adducts over time, only a few reports actually have investigated the biological fate of these SM-protein adducts from initial peak concentrations until return to zero-levels. A reliable identification of SM-proteins adducts (N-alkylated valine originated from the N-terminal valine of hemoglobin) was shown for up to 6 weeks post exposure (Noort et al. 1999). Recent reports reported a reliable verification of SM exposure by determination of the albumin adduct even 90 days post exposure (Xu et al. 2014).
Formation of SM-DNA adducts has been intensively investigated. It was shown in vitro that alkylation of guanine bases at N7 is the most frequent adduct resulting in the formation of N7-[2-[2-hydroxyethyl)thio[ethyl]guanine (N7-HETEG), followed by the inter- and intrastrand diadduct between two guanine bases resulting in bis[2-(guanine-7-yl)ethyl]sulfide (Bis-G) and N3-[2-[2-hydroxyethyl)thio]ethyl]adenine (N3-HETEA) was shown to be another major adduct (Fidder et al. 1994). The frequency of these major adducts may vary to some extend but in general the N7-guanine monoadduct represents about 60 % of all observed adducts, followed by N3-adenine monoadduct with about 15 % and the formation of N7-guanine diadduct is observed in 10 % (Fidder et al. 1994; Ludlum et al. 1986). During DNA repair SM-DNA adducts are cut out of affected DNA strands, are released into the circulation and then cleared from the body by renal filtration. N7-HETEG was also found to the most abundant SM-DNA adduct in vivo (Xu et al. 2014). A detection of free alkylated nucleoside bases in urine was possible at least to day 14 after exposure. This is supported by in vivo experiments using mice with topical SM exposure that revealed a time frame of 21 day for detection of N7-HETEG (Batal et al. 2013). HETE adducts cleaved from precipitated plasma proteins were detected at least up to day 40 in an accidental human exposure to SM (Smith et al. 2008).
13.3 Detection of Intact SM and SM Biotransformation Products
The high abundant availability of urine in combination with a non-invasive sampling and the clean matrix makes urine ideal for analyzing intact SM and its biotransformation products. Intact SM in urine or blood can be analyzed using GC-MS techniques (Vycudilik 1985, 1987; Koller and Szinicz 2009). Sodium chloride should be added to the samples to decrease SM hydrolysis (John et al. 2009). Later on, two-dimensional GC-MS/ESI in SIM mode allowed detection of 10 pg SM per mL blood or per gram tissue (Oostdijk et al. 2007). LC-MS based methods were also successfully applied to detect SM in blood (Dangi et al. 1994). In general, reports on the successful analysis of non-hydrolyzed SM in biological samples are rare most probably due to the limited stability of the highly reactive SM in biological matrices. In the majority of all reports, techniques addressing SM biotransformation products were used.
SM biotransformation products are eliminated via renal filtration. Thus, a detection of these compounds in urine can easily be achieved. The main SM hydrolysis product thiodiglycol (TDG) is a polar compound that has to be derivatized into more suitable compounds for GC-MS analysis (Koller and Szinicz 2009; Jakubowski et al. 1990). In addition, total TDG can be assessed by acidic or enzymatic hydrolysis from glucuronidated TDG. Moreover, TDG bound to blood proteins can be measured after release by alkaline hydrolysis (Capacio et al. 2004, 2008). The sulphur atom in SM biotransformation products is oxidized to sulphoxise or sulphone (Black and Noort 2007). TDGO is best analyzed after reduction to TDG using GC-MS. However, TDG was found at low levels in unexposed humans (Black and Noort 2007). Therefore, measurement of TDG only is inappropriate for verification. Conjugation of SM with glutathion and further transformation results in the formation of 1,1′-Sulfonyl-bis[2-S-(N-acetylcysteinyl)ethane]. Metabolic biotransformation through ß-lyase leads to the formation of specific ß-lyase biotransformation products (MSMTSE, SBMSE, SBMTE and SBETE) that were found unequivocal biomarkers of exposure to sulfur mustard (Noort et al. 2002; Koller and Szinicz 2009). GC-MS techniques are suitable to detect ß-lyase products. As for TDGO, an initial reduction is necessary. In vivo studies revealed that detection of ß-lyase products was possible up to 14 days after exposure (Lin et al. 2014b). A simultaneous detection of seven relevant biomarkers (SMO, TDG, TDGO, SBMTE, MSMTESE, SBMSE, SBESE and SBSNAE) using UPLC-MS/MS was described (Li et al. 2013). Using that approach verification of a s.c. injection of 3.3 mg SM per kg in rats was possible. A successful analysis of ß-lyase products in urine of SM exposed human individuals using either GC-MS/MS or in urine, blood and blister fluid using LC-MS/MS was reported (Barr et al. 2008; Xu et al. 2014).
13.4 Detection of SM-Protein Adducts
Methods for detection of SM adducts with cysteine, valine, histidine, aspartate/glutamic acid residues have been reported and have been successfully applied to verify a SM exposure (Andacht et al. 2014; Black and Noort 2007; Noort et al. 1996, 2002, 2008; John et al. 2009; Fidder et al. 1996a; Steinritz et al. 2015).
Regarding human serum albumin alkylation of the only cysteine 34 residue by SM has been reported (Andacht et al. 2014; Noort et al. 1999, 2004, 2008). Digestion of the alkylated albumin by pronase results in the liberation of an alkylated tripeptide (Cys-Pro-Phe) that can be analyzed by LC-MS/MS methods (Noort et al. 1999, 2004). Recent reports revealed that the current available pronase produced the dipeptide (Cys-Pro) instead of the expected tripeptide (Gandor et al. 2015). A LC-ESI MS/MS method was established for the simultaneous detection of CP dipeptide, CPF tripeptide and also QCPF tetrapeptide (Gandor et al. 2015). SM albumin adducts were successfully monitored in four individuals after accidental SM exposure up to 90 days after exposure (Xu et al. 2014).
Sensitive methods are available to detect SM adducts with the N-terminal valine of hemoglobin. Using a modified Edman degradation the alkylated valine is released. After further derivatization a detection using GC-MS methods is possible (Fidder et al. 1996a; Noort et al. 2008). This approach was successfully applied to verify SM exposure in five human casualties (Black et al. 1997).
13.5 Detection of SM-DNA Adducts
Alkylation of DNA bases after SM exposure does occur frequently resulting in the formation of four SM-DNA adducts. Alkylation of guanine bases at N7 (N7-[2-[2-hydroxyethyl)thio[ethyl]guanine (N7-HETEG) is the most frequent adduct. Inter- and intrastrand diadducts between two guanine bases resulting in bis[2-(guanine-7-yl)ethyl]sulfide (Bis-G) are next frequent. N3-[2-[2-hydroxyethyl)thio]ethyl]adenine (N3-HETEA) was shown to be another major adduct. A less abundant but biological highly relevant adduct is the alkylation product of guanine at the O6-residue resulting in the formation of O6-[2-[(2-hydroxyethyl)thio]ethyl]guanine (O6-HETEG). This adduct was found to account only for some 0.1 % of all adducts but apparently this lesion is lacking repair via DNA alkyltransferases and thus has to be considered as mutagenic (Ludlum et al. 1986). The most prevalent adduct N7-HETEG can be detected and quantified using LC-MS techniques (Fidder et al. 1994, 1996b). Comprehensive LC-MS based methods that allow a simultaneous quantification of all adducts in urine or blood are reported (Yue et al. 2014; Xu et al. 2014; Zhang et al. 2014). Alkylated bases were positively detected from day 3 up to day 32 after exposure, with a maximum peak on days 4–7 in a human exposure scenario (Xu et al. 2014).
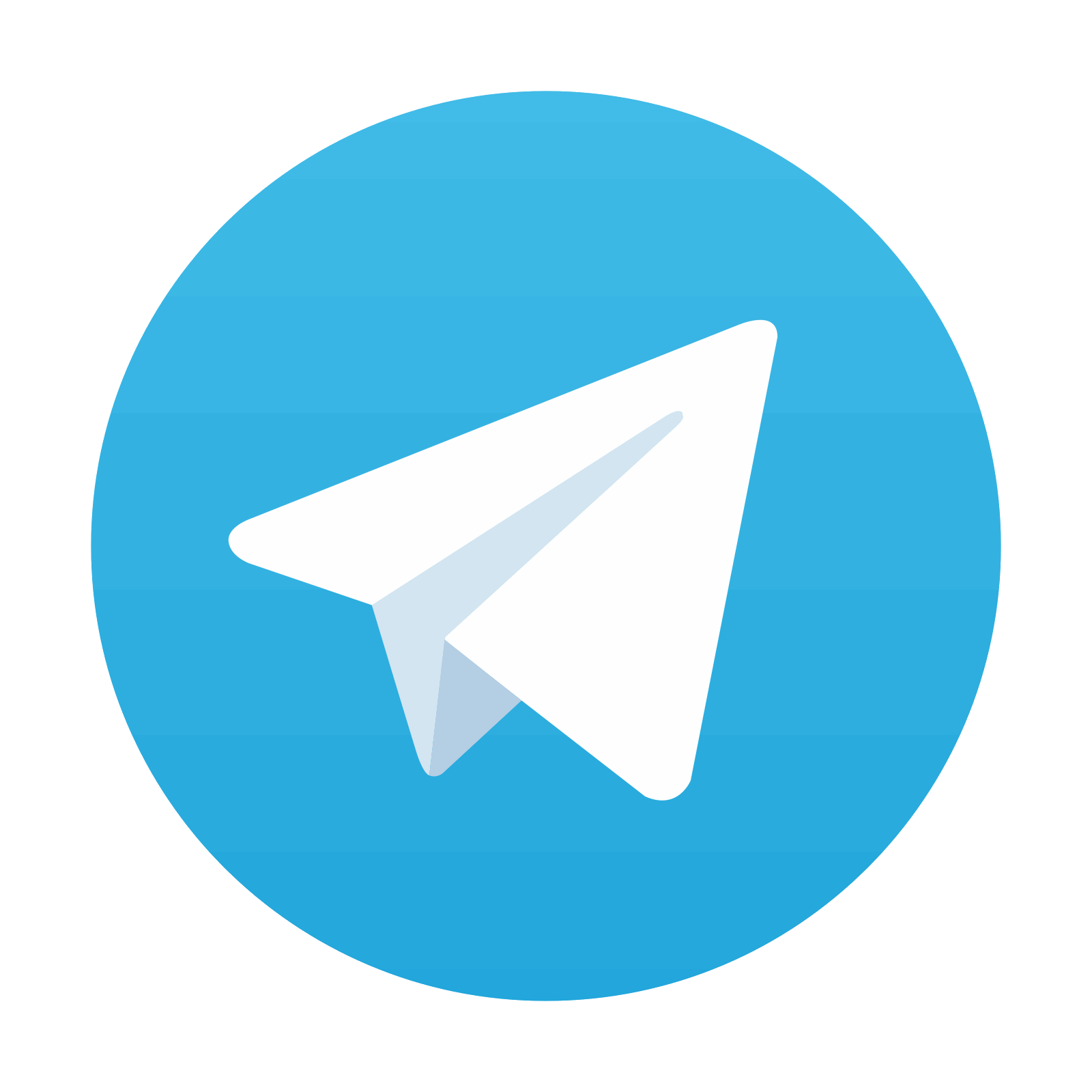
Stay updated, free articles. Join our Telegram channel

Full access? Get Clinical Tree
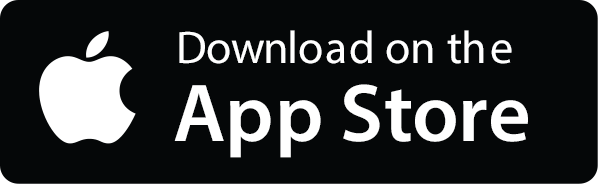
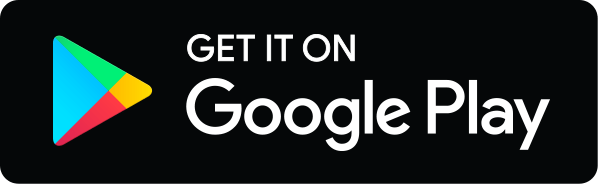