I. THE VEINS
Veins of the lower extremity (Figure 35–1) consist of superficial and deep systems joined by venous perforators. The great and small saphenous veins are superficial—veins, the name “saphenous” aptly derived from the Greek word for “manifest, clear,” or “visible.” They contain many valves and show considerable variation in their location and branching points. The great saphenous vein may be duplicated in up to 10% of patients. Typically, it originates from the superficial arch of the foot and is found anterior to the medial malleolus at the ankle. As it ascends in the calf just beneath the superficial fascia, it is joined by two major tributaries: an anterior vein, which crosses the tibia; and a posterior arch vein, which arises posterior to the medial malleolus beside the posterior tibial artery. The great saphenous vein then enters the fossa ovalis in the groin to empty into the deep femoral vein.
The saphenofemoral junction is marked by four or five prominent branches of the great saphenous vein: the superficial circumflex iliac vein, the external pudendal vein, the superficial epigastric vein, and the medial and lateral accessory saphenous veins. Another important anatomic landmark is the relationship of the great saphenous vein to the saphenous branch of the femoral nerve; as it emerges from the popliteal space, the nerve follows a course parallel to the vein. Injury during saphenous vein harvest for bypass produces neuropathic pain or numbness along the medial calf and foot. The small saphenous vein arises from the superficial dorsal venous arch behind the lateral malleolus at the ankle and curves toward the midline of the posterior calf, ascending to join the popliteal vein behind the knee.
Deep veins of the leg parallel the courses of the arteries. Two or three venae comitantes accompany each tibial artery. At the knee, these paired high-capacitance veins merge to form the popliteal vein, which continues proximally as the femoral vein. At the inguinal ligament, the femoral and deep (profunda) femoral veins join medial to the femoral artery to form the common femoral vein. Proximal to the inguinal ligament, the common femoral vein becomes the external iliac vein. In the pelvis, external and internal iliac veins join to form common iliac veins that empty into the inferior vena cava (IVC). The right common iliac vein ascends almost vertically to the IVC while the left common iliac vein takes a more transverse course. For this reason, the left common iliac vein may be compressed between the right common iliac artery and lumbosacral spine, a condition known as May–Thurner syndrome when thrombosis of the left iliac vein occurs.
Muscular sinusoids represent another component of the deep veins of the leg. These thin-walled, nonvalved venous lakes run longitudinally within soleus muscle bellies and then coalesce to join the posterior tibial and peroneal veins. Blood empties from these sinusoids during muscle contraction; inactivity leads to stasis and may contribute to the development of deep venous thrombosis (DVT).
Blood flow is directed from superficial to deep veins of the leg via valved perforating (communicating) veins. Perforators are located below the medial malleolus (inframalleolar perforator), in the medial calf (Cockett perforators), at the level of the adductor canal (Hunterian perforator), and just above (Dodd perforator) and below (Boyd perforator) the knee.
Delicate bicuspid venous valves prevent reflux and direct the flow of blood from the foot and leg toward the heart and generally against gravity. Valves are more numerous in the distal part of the extremity, decrease in number proximally, and are virtually absent in the IVC itself.
The IVC ascends in the abdomen and ends at the right atrium. It lies to the right of the midline, lateral to the aorta, and receives a number of lumbar veins that connect with the vertebral and paravertebral venous plexuses. The IVC and its tributaries are derived in the 6th to 10th week of life from the fusion and obliteration of several paired embryonic veins: the anterior and posterior cardinal veins, the subcardinal veins, the supracardinal veins, and the sacrocardinal veins. Because of this complex embryonic development, anomalies in the venous system are not uncommon. The most common abnormality is a circumaortic left renal vein (1.5%-8.7% incidence), followed by a retroaortic left renal vein (1.2%-3.4% incidence), duplication of the IVC (1%-3% incidence), azygos and hemiazygos continuation of the IVC (0.6%), and left-sided IVC (0.2%-0.5% incidence). An unsuspected retroaortic or circumaortic renal vein can be inadvertently injured during aortic cross-clamping. Most unusual is the congenital absence of the suprarenal IVC, which results from failure of the right subcardinal vein branches to join the veins of the embryologic liver. This ultimately results in the infrarenal segment of the IVC joining the azygos-hemiazygos veins to drain into the superior vena cava. The hepatic veins drain directly into the right atrium in patients with this unusual anomaly.
Veins of the upper extremity are also divided into superficial and deep groups, though direction of flow from superficial to deep is not as distinct as in the lower extremity. Dorsal veins of the hand empty into the cephalic vein (“intern vein”) on the radial aspect and into the basilic vein on the ulnar aspect of the forearm. The cephalic vein ascends lateral to the biceps muscle into the deltopectoral groove, where it passes through the clavipectoral fascia to join the axillary vein. The cephalic vein is useful for arteriovenous fistulas, both at the wrist and in the upper arm, because it is superficial and lateral in the arm, allowing easy access for hemodialysis needles. The basilic vein, which runs medially in the arm to become the axillary vein, is deeper and thicker walled than the cephalic vein. Its many branches make it tedious to harvest, but it can be used for a bypass conduit or for a laterally tunneled upper-arm dialysis fistula (basilic vein transposition). The median cubital vein links the cephalic and basilic veins in the antecubital space.
Paired brachial veins comprise the deep system of veins. They accompany the brachial artery and join the basilic vein as it becomes the axillary vein. The axillary vein continues medially as the subclavian vein, which passes through a tight space anterior to the first rib and anterior scalene muscle and posterior to the clavicle. The subclavian vein and the internal jugular vein join behind the clavicular head to form the brachiocephalic vein, which empties into the superior vena cava.
Knowledge of lower extremity venous anatomy is essential to understanding the physiology of venous flow. The volume of blood in the lower extremities may increase by one-half liter when an individual moves from a reclining to an erect position (Figure 35–2). Increased blood volume is accommodated by increased venous capacitance, which is regulated by smooth muscle contractility in the vein walls. Erect posture creates a vertical column of blood, exerting a hydrostatic pressure equal to the distance from the toes to the right atrium (about 100-120 mm Hg). The hydrostatic pressure must be overcome to avoid pooling of blood in the legs and to provide venous return to the heart. Several aspects of the venous system make it possible to move blood against the force of gravity. Because the circulation is a closed system, venous return is affected by arterial inflow and by the negative intrathoracic pressure created during inspiration. Functional valves assure unidirectional movement of blood from superficial to deep systems and from the foot back to the heart. Hydrostatic pressure is dissipated by lack of simultaneous opening of the valves. Soleal venous sinusoids are another central component to the system. When the muscle contracts during exercise, blood empties from the sinusoid into the deep veins of the calf and leg. This high-velocity flow siphons blood from deep veins of the foot upward into the calf, analogous to smoke being drawn up a smokestack by wind blowing past the chimney (Venturi effect).
Figure 35–2.
Pedal venous pressure measurements with exercise. The normal drop in pressure associated with ambulation is impaired by deep vein incompetence and proximal venous obstruction. (Reproduced, with permission, from DeWeese JA: Venous and lymphatic disease. In: Schwartz S, ed. Principles of Surgery, 4th ed. McGraw-Hill, 1983.)
DISEASES OF THE VENOUS SYSTEM
Varicose veins are very common, afflicting 10%-20% of the world’s population. Abnormally dilated veins occur in several locations in the body: the spermatic cord (varicocele), esophagus (esophageal varices), and anorectum (hemorrhoids). Varicosities of the legs were described as early as 1550 BC and in the 1600s AD were correlated with trauma, childbearing, and “standing too much before kings.” Modern studies identify female sex, pregnancy, family history, prolonged standing, and a history of phlebitis as risk factors for varicose veins. In the Framingham Study, the highest incidence was found in women between 40 and 49 years of age.
Varicose veins are classified as either primary or secondary. Primary varicose veins are thought to be due to genetic or developmental defects in the vein wall that cause diminished elasticity and valvular incompetence. Most cases of isolated superficial venous insufficiency are primary varicose veins. Secondary varicose veins arise from destruction or dysfunction of valves caused by trauma, DVT, arteriovenous fistula, or nontraumatic proximal venous obstruction (pregnancy, pelvic tumor). When valves of the deep and perforating veins are disrupted, chronic venous stasis changes may accompany superficial varicosities. It is important to recognize that untreated, longstanding venous dysfunction from either primary or secondary varicose veins may cause chronic skin changes that lead to infection, nonhealing venous ulceration, and chronic disability. To define the optimal method of treatment, the etiologic factors and distribution of disease must be clearly identified.
The clinical presentation of patients with varicose veins can be quite variable. Many varicose veins are asymptomatic and come to medical attention because of aesthetic concerns. If symptomatic, varicose veins may be associated with localized pain, a burning sensation over the vein, a diffuse ache or “heaviness” in the calf (particularly with prolonged standing), or phlebitis. Mild ankle edema may occur. Symptoms generally improve with leg elevation.
The varicosities appear as dilated, tortuous, elongated veins predominantly on the medial aspect of the lower extremity along the course of the great saphenous vein. Overlying skin changes may be absent even in the presence of extensive large varicosities. Smaller flat, blue-green reticular veins, telangiectasias, and spider veins may accompany varicose veins and are further evidence of venous dysfunction. A cluster of telangiectasias below the inframalleolar perforator is termed a corona phlebectatica paraplantaris. Secondary varicose veins can cause symptoms characteristic of chronic venous insufficiency (CVI), including edema, hyperpigmentation, stasis dermatitis, and even venous ulcerations.
Physical examination begins with inspection of all extremities to determine the distribution and severity of the varicosities. Bimanual circumferential palpation of the thighs and calves is helpful. Palpation of a thrill or auscultation of a bruit indicates the presence of an arteriovenous fistula as a possible etiologic factor. Today, tourniquet tests have been virtually replaced by venous duplex ultrasound imaging, which is now used as the primary method with which to map the location and extent of reflux.
Ulceration, brawny induration, and hyperpigmentation often indicate accompanying chronic deep venous insufficiency. This is important to recognize because the changes generally do not resolve with saphenous vein stripping alone.
Klippel–Trenaunay syndrome (KTS) must be considered if extensive varicose veins are encountered in a young patient, especially if unilateral and in an atypical distribution (lateral leg). The classic triad of KTS is varicose veins, limb hypertrophy, and a cutaneous birthmark (port wine stain or venous malformation). Because the deep veins are often anomalous or absent, saphenous vein stripping can be hazardous. Standard treatment for patients with KTS is graduated support stockings, and a good program of intermittent leg elevation and exercise. Limited stab avulsion of symptomatic varices after thorough duplex ultrasound vein mapping, and occasional surgery for correction of limb length discrepancy may also be indicated.
Treatment for both primary and secondary varicose veins initially involves a program directed at management of venous insufficiency, including elastic stocking support, periodic leg elevation, and regular exercise. Prolonged sitting and standing are discouraged. For most patients, knee-high or thigh-high gradient compression stockings of 20-30 mm Hg are sufficient, although some patients require 30-40 mm Hg pressure. The compression stockings are worn all day to diminish venous distention during standing and are removed at night.
Traditionally, open surgical approaches have been the mainstay of treatment for saphenous vein disease. The preferred surgical option for saphenous veins remains high ligation with stripping or high ligation alone (Figure 35–3). High ligation and stripping of the saphenous system is performed for patients with an incompetent valve at the saphenofemoral junction and varicosities throughout the length of the great saphenous vein. This was traditionally performed by ligating the saphenofemoral junction and the major proximal saphenous vein branches through a small incision in the groin. Then the saphenous vein was removed to the point of clusters of varicosities. For the small saphenous vein, high ligation alone with short-length phlebectomy with local anesthesia is typically performed to minimize sural nerve injury. Epifascial veins may also be treated in an ambulatory setting, with phlebectomy performed under local anesthesia. Today, most open surgical approaches have been replaced with endovascular techniques (see next paragraph, C). A new treatment strategy called ASVAL (ambulatory selective varices ablation under local anesthesia) embraces the surgical treatment of epifascial veins while sparing the refluxing saphenous vein, minimizing the need to treat the saphenous vein in many cases. Although controversial, under the principle of anterograde reflux such that reflux spreads from epifascial to saphenous veins, the treatment of epifascial veins alone is said to correct saphenous reflux. Ongoing research has demonstrated that patients with primary varicose veins or less severe disease have done well with this approach.
In recent years, great advances have been made in endovascular techniques for treatment of varicose veins. Ablation techniques include thermal (radiofrequency and laser) and chemical ablation. Thermal ablation causes treated veins to collapse due to vein wall and lumen fibrosis. Vein reabsorption occurs over the course of several months. Both radiofrequency and laser ablation have been found to be safe and effective in the treatment of incompetent greater saphenous veins (GSVs), and the latest 2011 consensus opinion recommends thermal ablation to surgical or chemical ablation for GSVs. Its use has also been reported in successful treatment of perforator veins, though recent guidelines recommend against selective treatment of incompetent perforating veins in patients with simple varicose veins. However, in patients with “pathologic” perforating veins, subfascial endoscopic perforating vein surgery, ultrasonographically guided sclerotherapy, and thermal ablations remain viable options. Finally, advances have been made in chemical ablation techniques in the form of foam sclerotherapy. Compared to liquid sclerotherapy, foam has increased time of endothelial contact as it expands to fill the vein. Given its ease of use, it is a suggested option for treatment of incompetent saphenous veins and a recommended option along with phlebectomy for treatment of varicose tributaries.
DVT and pulmonary embolism (PE) affect up to 900,000 people per year in the United States, and their incidence increases with age. Treatment is estimated to cost billions of dollars per year, not even including expenditures associated with long-term sequelae of this disease.
The Virchow triad (stasis, vascular injury, and hypercoagulability) should be the cornerstone for assessment of risk factors for DVT. In most cases, the cause is multifactorial.
Acquired risk factors include advanced age, cancer, surgery, trauma, immobilization, hormone replacement therapy, oral contraceptive use, pregnancy, neurologic disease, cardiac disease, and antiphospholipid antibodies. Approximately 30%-40% of patients with a new DVT (both upper and lower extremity) have been found to present with malignancy within 5 years. In men, pancreatic and colorectal cancers are most frequently associated with thrombotic risk, while hematological malignancies carry a lower risk. Cancers of the pancreas, ovary, and brain are mostly associated with thrombotic complications. Breast cancer, especially during its chemotherapy treatment, is also a risk factor. Most of these malignancies are associated with increased fibrinogen or thrombocytosis.
Endothelial injury can result from direct trauma (severed vein, venous cannulation, or transvenous pacing) or local irritation secondary to infusion of chemotherapy, previous DVT, or phlebitis. Damaged endothelium leads to platelet aggregation, degranulation, and formation of thrombus as well as vasoconstriction and activation of the coagulation cascade. Thrombin activation from release of tissue factor and diminished fibrinolysis mediated by plasminogen activator inhibitor are intraoperative events that may be related to endothelial disruption.
Hypercoagulable states may also be inherited. Genetic causes include deficiencies of natural coagulation inhibitors (antithrombin III, protein C, protein S), factor V Leiden, prothrombin 20210A gene variant, blood group non-O, elevated homocysteine levels, plasminogen abnormalities, elevated levels of coagulation factors (such as factor VIII), and reduced heparin cofactor II activity. Hematologic disorders associated with DVT include disseminated intravascular coagulation, heparin-induced thrombocytopenia, antiphospholipid antibody syndrome, thrombotic thrombocytopenic purpura, hemolytic uremic syndrome, polycythemia vera, and essential thrombocythemia. Inflammatory bowel disease, systemic lupus erythematosus, and obesity are additionally associated with DVT.
DVT occurs most frequently in the calf veins, though it may arise in the femoral or iliac veins. Thrombi originate in soleal sinusoids or in valve sinuses, where there are flow eddies. Treatment of isolated calf vein thrombosis is controversial, as it is associated with a low risk of PE. However, a 2011 meta-analysis of studies spanning nearly 25 years demonstrated significant reductions both in progression to PE and in proximal thrombus propagation in patients treated with anticoagulation compared to patients who not receiving anticoagulation. While the studies were heterogeneous, the summation of the data indicated no statistical increased risk of bleeding in patients who received anticoagulation compared to the control arms. These observations and the improved safety profile of low-molecular-weight heparin (LMWH) are compelling practitioners to treat isolated calf DVTs aggressively. In the latest 2012 ACCP guidelines, it is recommended that “in patients with acute isolated distal DVT of the leg and without severe symptoms or risk factors for extension, we suggest serial imaging of the deep veins for 2 weeks over initial anticoagulation” while “in patients with acute isolated distal DVT of the leg and severe symptoms or risk factors for extension, we suggest initial anticoagulation over serial imaging of the deep veins.” The length of anticoagulation recommended is for 3 months.
The diagnosis of DVT cannot be made solely on the basis of presenting symptoms and signs, as up to half of patients with acute thromboses have no abnormality detectable in the involved extremity. Homans sign (pain on passive dorsiflexion of the ankle) is nonspecific and positive in only half of cases, and is unreliable. Some patients present with acute PE unaccompanied by leg edema or pain.
Symptomatic patients most often complain of a dull ache or pain in the calf or leg associated with mild edema. With extensive proximal DVT, there can be massive edema, cyanosis, and dilated superficial collateral veins. Low-grade fever and tachycardia occasionally occur. Iliofemoral venous thrombosis can result in phlegmasia. In phlegmasia alba dolens, the leg is pulseless, pale, and cool, which may progress to phlegmasia cerulea dolens, characterized by cyanosis of the limb and a precursor to gangrene.
Because DVT is difficult to diagnose on the basis of physical signs or symptoms, some objective diagnostic study is required before treatment is started. Historically, the standard for diagnosis was ascending phlebography, accomplished by fluoroscopic imaging during contrast injection into an intravenous line on the dorsum of the foot. The patient stands but is non–weight-bearing on the extremity studied. An abrupt cutoff of the contrast column indicates DVT. The complications of this procedure include risk of contrast allergy, contrast-induced nephropathy, and phlebitis.
Duplex ultrasound is now the best initial test to detect the presence of DVT. It is a noninvasive examination, does not expose the patient to radiation, is easily reproducible, and has specificity and sensitivity of greater than 95%. In this method, the transducer produces a two-dimensional image of the imaged vein and the surrounding tissue. Normally, veins collapse completely when compressed. Vein incompressibility is the primary ultrasonographic diagnostic criterion for acute lower limb DVT. Secondary diagnostic criteria include vein distention, echogenic thrombus within the vein lumen, absence of spectral or color Doppler signal from the vein lumen, and loss of venous flow phasicity and/or loss of response to Valsalva maneuver. Additionally, augmentation is expected in normal, compressible veins. When pressure is applied to the lower extremity distal to the area of evaluation, normally there is increased blood from through the vein with compression. Lack of augmentation is suggestive of an occlusion in the segment of vein between the site of compression and the transducer. It is worth noting that direct thrombus visualization using B-mode imaging is variable depending on the age of the clot: fresh thrombus is anechoic and therefore not visible. Finally, while duplex ultrasound has a high accuracy for proximal DVT, it is less accurate in distal thrombosis and is highly operator dependent. Magnetic resonance venography shows promise as a diagnostic study for this disorder. The sensitivity and specificity of magnetic resonance venography are 100% and 96%, respectively. The injection of gadolinium is useful for determining the age of the thrombus. Advances in magnetic resonance direct thrombus imaging with methemoglobin as an endogenous contrast allows for accurate diagnosis of femoropopliteal DVT with a sensitivity and specificity greater than 100%. This method allows for the paramagnetic properties of methemoglobin to provide a high signal on T1-weighted images against a background of flowing blood and fat. The thrombus may be directly visualized without administration of contrast, making it a viable option for patients with renal insufficiency. CT imaging, especially as part of a PE protocol CT image, may also be a good alternative to establish the diagnosis.
Measurement of D-dimer levels is too nonspecific for use alone and, when combined with a negative risk assessment, may be useful to rule out DVT but not to rule in the diagnosis of DVT. Radiolabeled fibrinogen is too sensitive in the pelvis for use in the acute clinical setting and carries with it a risk of transmission of infectious disease. It is no longer used. Older tests such as impedance plethysmography and venous pressure measurements do not achieve the same accuracy as duplex ultrasound and have been largely abandoned. Recent studies have demonstrated the potential for using soluble P-selectin as a marker for diagnosing DVT. P-selectin is a cell adhesion molecule that is the first upregulated glycoprotein on activated endothelial cells and platelets. As thrombosis and inflammation are interrelated, elevated levels of soluble P-selectin acts as an indicator or biomarker of inflammation. Consequently, a combination of soluble P-selectins with a Wells score has been shown to have a positive predictive value of approximately 90% with a negative predictive value greater than 95% for establishing the diagnosis of DVT.
A variety of pathologies may mimic the symptoms of DVT. Lymphatic conditions such as adenopathies or lymphangitis can present with lower extremity pain and edema, but without flow on color Doppler images seen with DVTs. Vascular lesions such as hematomas and pseudoaneurysms secondary to catheterization of the common femoral artery may simulate DVTs. These can be differentiated from DVTs under evaluation by gray-scale images showing a delineation of the pseudoaneurysm or hematoma, or by color Doppler imaging which will illustrate the typical “yin-yang” sign of bidirectional flow. Muscular lesions of the thigh such as muscle strains, tears, and contusions may result in pain, stiffness, edema, and a mass. Cellulitis may cause edema, localized pain, and erythema. Unilateral leg swelling can also result from lymphedema, obstruction of the popliteal vein by Baker cyst, or obstruction of the iliac vein by retroperitoneal mass or idiopathic fibrosis. Bilateral leg edema suggests heart, liver, or kidney failure or IVC obstruction by tumor or pregnancy.
Treatment is aimed at reducing the incidence of complications associated with DVT. Short-term complications include recurrent DVT or pulmonary thromboembolism, while long-term complications include the development of varicose veins and chronic venous insufficiency. The primary treatment of DVT is systemic anticoagulation. This reduces the risk of PE and extension of venous thrombosis and also decreases the rate of recurrent DVT by 80%. Systemic anticoagulation does not directly lyse thrombi but stops propagation and allows natural fibrinolysis to occur. Heparin is initiated immediately and dosed to a goal partial thromboplastin time (PTT) of 1.5-2.5 times normal, or, more currently, LMWH, weight-based without monitoring. Achieving therapeutic heparinization within the first 24 hours after diagnosis is shown to reduce the rate of recurrent DVT.
Warfarin is started after therapeutic heparinization. The two therapies should overlap to diminish the possibility of a hypercoagulable state, which can occur during the first few days of warfarin administration, because warfarin also inhibits the synthesis of natural anticoagulant proteins C and S. For both acute lower extremity DVT and acute PE, guidelines recommend starting warfarin the same day as the start of parenteral anticoagulation over delayed initiation, with a continuation of parenteral anticoagulation for a minimum of 5 days until the INR is greater than 2.0 for more than 24 hours. Specifically, in patients with a PE or proximal DVT of the leg provoked by surgery, the recommended treatment is 3 months compared to a longer or shorter time period. Three months of anticoagulation is similarly recommended in patients with (i) PE/proximal DVT of the leg provoked by a nonsurgical transient risk factor, (ii) in patients with PE/an isolated distal DVT of the leg provoked by surgery or by a nonsurgical transient risk factor. In patients who have had an unprovoked PE or DVT of the proximal or distal leg, the recommended treatment is a minimum of 3 months’ treatment followed by reevaluation of risk-benefit ratio of extended therapy. After a second episode of DVT, the usual recommendation is prolonged or lifelong warfarin in patients with a low or moderate bleeding risk. In patients with a high bleeding risk, the guidelines recommend 3 months of anticoagulation over extended therapy. The risk for recurrent venous thrombosis is increased markedly in the presence of homozygous factor V Leiden mutations, antiphospholipid antibody, antithrombin III, and protein C or protein S deficiencies, so lifelong anticoagulation is usually recommended for these conditions as well.
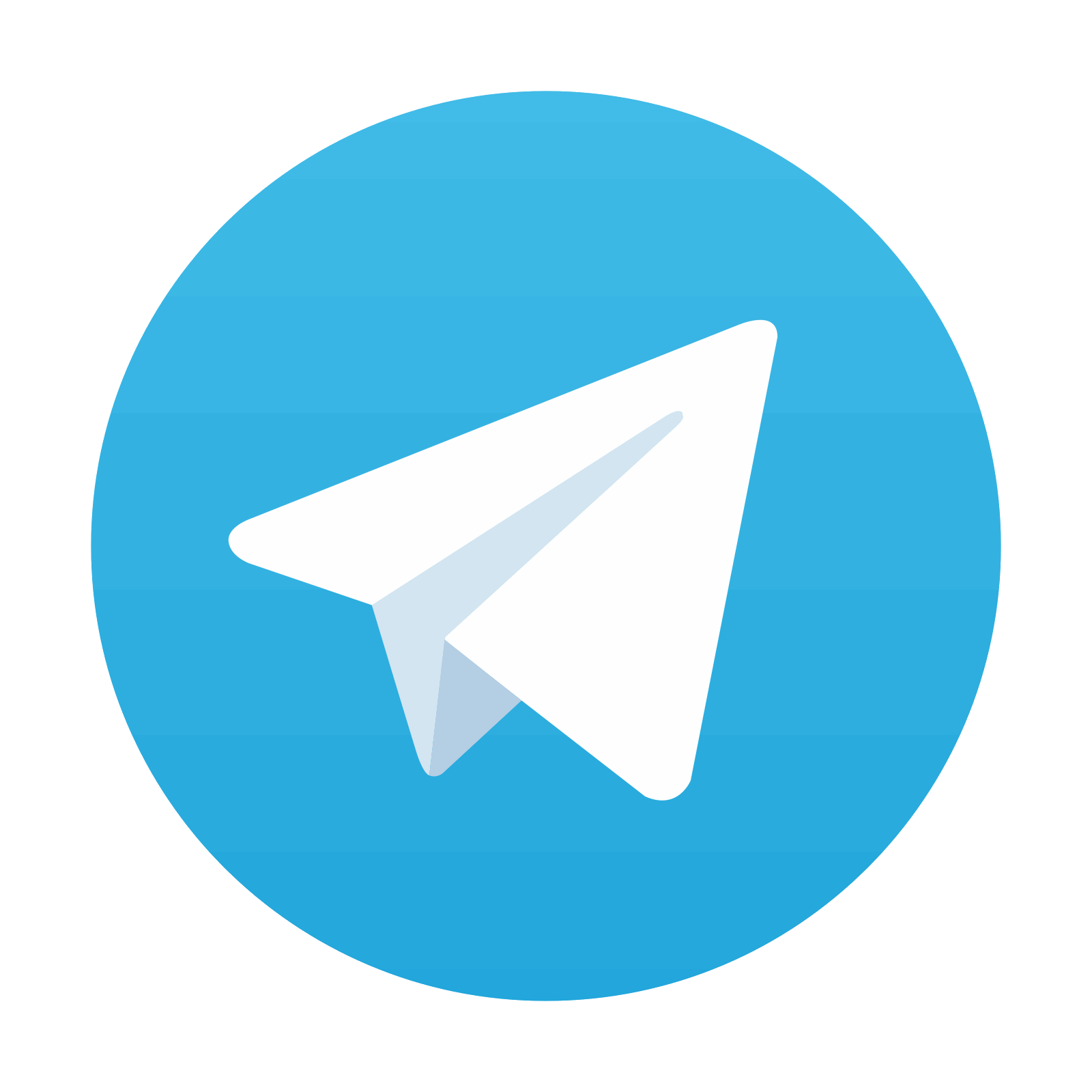
Stay updated, free articles. Join our Telegram channel

Full access? Get Clinical Tree
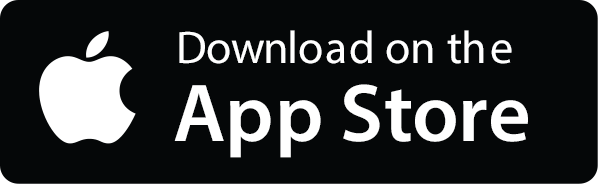
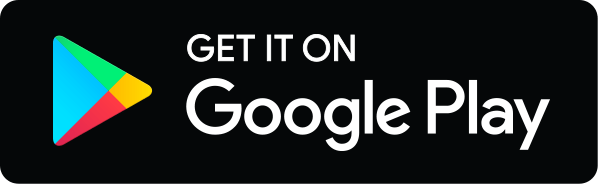