This chapter covers the analytical tasks typically associated with quality control of botanicals. Whether it is TLC quickly offers reliable answers and many advantages. Based on the methodol ogy outlined in the previous chapter, you will find answers below to frequently asked questions including: What can I do with HPTLC? How do I interpret the result? What are the limitations of the method? Chapter 4 comprises three sections, which should be read in sequence. The first is a general discussion based on examples. It is focused on the specialties of HPTLC relative to other chromatographic techniques. The second section covers issues related to the identity and quality of botanical raw materials. Main elements of the last section on preparations and finished products are process control and stability testing. Information about quantitative determination of individual chemical substances in botanicals is also provided. For better understanding of all the terms (herbal drugs, HMP, etc.), please refer to Appendix A. ♦Why HPTLC? A comparison of HPTLC to other chromatographic methods was presented in Chapter 1. There, the principal advantages and limitations of each technique were discussed in general terms. Here, we will focus on the specifics of herbal analysis. Herbal drugs and herbal drug preparations are complex mixtures of compounds. From a medicinal point of view, the drug in its entirety must be considered as the active principle. This can create several problems for the analyst: Most analytical questions can be suitably answered on the basis of chromatographic fingerprints, which best represent the complexity of the analyte. For that reason, it seems that the chromatographic technique with the highest resolving power using a detector allowing positive identification would be the best choice. GC/MS, GC/IR, HPLC/DAD/MS, and HPLC/NMR come to mind. Such analyses tend to be costly, time consuming, and rather sophisticated, but substitution may not be possible. If answers to certain questions are sought, the full range of analytical tools is necessary. However, it should always be evaluated on a case-by-case basis whether HPTLC is able to provide either equally suitable results with less effort or complementary information that can help in a decision-making process. At times it can be useful also to rephrase the analytical question. For example, instead of basing a decision about acceptance or rejection of a batch of raw material on a sophisticated LC/MS method for quantitative determination of a certain marker X, a simpler qualitative but highly selective HPTLC fingerprint may be chosen, which indicates adulteration as a sequence of characteristic zones. The analytical question “Does the content on compound (specific marker for adulterant Y) in the sample exceed the specified limit?” can then be rephrased “Does the HPTLC fingerprint of the sample comply with the specification or are there additional zones indicative of adulteration with Y?” If zones indicative of adulteration are found, the sample can be subjected to further quantitative analysis of compound X. Some of the advantages of HPTLC shall be reemphasized at this point: Figure 4-1 Gas chromatograms of peppermint oil (Mentha piperita, black) and mint oil (Mentha arvensis, red). (Courtesy of R. Della Casa, Essencia, Switzerland.) Let us now look at some examples in greater detail. GC/MS is generally employed for the analysis of essential oils. Each component is easily identified, and the experienced analyst can even detect impurities. As seen in Figure 4-1 gas chromatograms are characterized by a large number of sharp and well-resolved peaks. In addition to the retention time, the mass spectrum of each compound can be recorded and used for identification. Peppermint oil (Mentha piperita) and mint oil (Mentha arvensis) can be mixed up. Yet in GC, the two oils give quite similar fingerprints with only a few distinctly different regions. Menthofuran at 25.61 minutes is characteristic for peppermint, isoisopulegol (28.49 minutes) identifies mint oil. The analysis time is about 40 minutes for each sample. For a quick preliminary investigation and reliable distinction of the two oils, HPTLC can also be used. The chromatograms shown in Figure 4-2 allow a clear differentiation based on the compound iso-isopulegol (gray arrow). The analysis of the two samples (including all steps) requires about 25 minutes. Each additional sample, which is analyzed on the same plate, would only extend the overall analysis time by the time required for sample application. With the selected application parameters, up to a total of 18 samples can be evaluated. The following example also demonstrates the power of HPTLC. Figure 4-3A compares sage oil samples. One oil was suspected to be adulterated, based on a simple solubility test regularly performed on raw materials. Whereas investigation by GC did not reveal any flaw in quality, a single HPTLC analysis was able to show very distinctly that an additional substance was present in the questionable sample (arrow in Figure 4-3B). Also seen is a correspondingly lower content of the remaining constituents of the oil. This indicates a cut with a simple material and not with a different essential oil. After isolation by preparative TLC, the compound was identified as fatty oil. Ginkgo extracts are usually analyzed by HPLC/DAD.1 The fingerprint is clearly structured, and most components are well resolved. Identification is not problematic, but the analysis is time consuming. To speed up analysis and save costs, HPTLC fingerprints can be used for quality control as well. Figure 4-4 compares the HPLC and HPTLC chromatograms of several ginkgo extracts. Figure 4-2 HPTLC fingerprints of peppermint oil (left tracks) and mint oil (right tracks) under white light (A) and UV 366 nm (B). MP: toluene, ethyl acetate (95:5). D: anisaldehyde reagent. The arrows mark the zones specific for mint oil; gray arrows, iso-isopulegol; pink arrow, unknown substance. ♦ HPTLC Analysis of Raw Material Identification General Regulatory agencies recommend fingerprint chromatography as the basis for proper identification of herbal drugs, herbal drug preparations, and herbal medicinal products. In general, the fingerprint of one sample (unknown) is compared with that of another sample (i.e., reference material). The reference material can either be of the same kind as the unknown (herbal drug, or preparation thereof) or be a solution of any number of chemically defined substances. In the identity test, the sample must meet certain predefined acceptance criteria. The only requirement of the procedure is specificity in the sense that the sample, of which the identity is to be established, meets the acceptance criteria and that any other material such as adulterants will fail the test. Figure 4-3 Comparison of sage oils (Salvia officinalis) by GC (A) and HPTLC (A courtesy of R. Della Casa, Essencia, Switzerland.) (B). MP: toluene, ethyl acetate (95:5). D: anisaldehyde reagent. Tracks 1/2 and 3/4: sage oils of good quality; tracks 5/6, adulterated oil (2 and 5 µL each, respectively, in each pair). The substance marked by the arrow is indicative for the Figure 4-4 Comparison of the flavonoid profiles of several ginkgo extracts by HPLC (A) and HPTLC (B). (A) HPLC: mini-bore Phenomenex Luna 5 µ C18 (2) 250 2.0–mm column at 45C. MP acetonitrile and 0.3% formic acid ratios were changed after 15 minutes from 15:85 to 25:75. Total run time 33 minutes at a flow rate of 400 L/min. Detection at 350 nm. (B) HPTLC MP: ethyl acetate, acetic acid, formic acid, water (100:11:11:26). D: Natural Products reagent, UV 366 nm. Track 1, rutin; tracks 2 and 3, ginkgo leaves; tracks 4 to 11, ginkgo extracts. (A from Dubber MJ, Kanfer I. High-performance liquid chromatographic determination of selected flavonols in Ginkgo biloba solid oral dosage forms. J Pharm Pharmaceut Sci 2004;7[3]:303–309.) Compared with other chromatographic techniques, HPTLC is an ideal tool for identification tests because it is faster, easier, and more flexible. The unknown and the reference material can be chromatographed side by side on the same plate. Fingerprints are compared with respect to number, sequence, position, and color of separated zones. In the simplest case, finding out whether substances A and B are the same, this can be done with very little instrumentation, even under “field conditions.” The possibilities for advanced investigations are numerous. Many unknowns can be investigated in parallel, and an HPTLC fingerprint, or better, sets of fingerprints based on multiple detection of the same plate, can provide very characteristic visual impressions, which distinctly “describe” even complex samples. Compared with GC or HPLC chromatograms, such HPTLC fingerprints show less resolution but are still selective enough to detect differences between samples. Given a reliable methodology, this allows establishing two samples as equal or different within certain limits. In the following subsections, we will illustrate the requirements for successful application of HPTLC for identification of raw materials. First, we discuss method selection for generating a suitable fingerprint of authenticated plant material. Then, we want to apply this method to the analysis of a large number of batches for evaluation of the natural variability. A fingerprint is established, which, within limits, is representative of a given species. Finally, we discuss how the identity of an unknown sample can be established and properly described paying attention to possible adulteration. Selecting the Fingerprint In fingerprint analysis it is desirable to obtain a broad spectrum of information about the sample. A representative sample should be taken for extraction. That means typically more than one plant must be included to ensure that the fingerprint is representative of a batch. From another perspective, it is important to choose a fingerprint that is selective enough to bring out special features. This will allow detecting significant differences between two or more samples with certainty. Rather than including the substances common to many plants, it is a good approach to select only a certain group of chemical constituents as a target for fingerprint analysis. Box 4–1 Which Fingerprint Is Best? A balance must be found between the number/type of compounds extracted and those of interest because unwanted substances will disturb the analysis. Think of proteins, lignanes, or sugars; they may constitute an undesirable matrix! In cases where the active principles are known, those can serve as markers. If no actives have been determined, secondary metabolites like essential oils, flavonoids, alkaloids, or others may be chosen. Even ubiquitous constituent groups such as amino acids, plant acids, or sterols can provide plant-specific profiles. There are also cases where very little or nothing is known about chemical constituents of a given herbal drug. For those cases, the option of generating multiple fingerprints is very valuable and should be mentioned again. Multiple finger prints can be obtained either from the same plate by multiple detections or from the same sample looking at different fingerprints repre senting various substance classes (i.e., polar andnonpolar compounds). Multiple detection allows observation of different features of the same chromatogram. As an example, the identification of valerian according to the AHP monograph2shall be discussed (Fig. 4–5). Four different detections were performed on the same plate. From all of them, the same conclusion can be drawn: The species differ significantly, and there is resemblance within V. officinalis (see also Box 4–2).Each individual detection would be sufficient, but it is the complete package that offers comprehensive information: (a) Quenching of the fluorescence indicator: all compounds absorbing UV 254 nm are visible. Sample 3 is different from 1, 2, and 4 because of an additional zone. (b) White light after derivatization with hydrochloric acid–acetic acid: in particular, valepotriates appear as brown-green to blue zones especially in samples 6 and 7. (c) Fluorescence under UV 366 nm after additional derivatization with anisaldehyde reagent: same information as in (b), but the lower RF region is better structured. The samples on tracks 6 and 7 show very distinctive fluorescent zones. (d) White light (same derivatization mode as (c): in particular, valerenic acid (and derivatives) is detected as a purple zone. Multiple fingerprints based on different chromatographic systems generate comprehensive information about a botanical drug (Fig. 4–6). Green tea contains several substances, including alkaloids (caffeine, etc.), flavonoids, polyphenols (epicatechin, etc.) and amino acids (theanine). The combination of all fingerprints allows a comprehensive description of the sample. Figure 4-5 HPTLC fingerprints of various valerian samples (Valeriana spp.). MP: hexane, ethyl acetate, acetic acid (65:35:0.5). (A) UV 254 nm. (B) D: hydrochloric acid–acetic acid, white light. (C) D: anisaldehyde reagent, UV366 nm. (D) D: anisaldehyde reagent, white light. Tracks 1 to 4, four samples of V. officinalis; track 5, valerenic acid; track 6, V. sitchensis; track 7, V. wallichii. For details, see Box 4–1. Let us start with the example of a well-investigated plant. For Echinacea, several groups of active constituents are known. They include, among others, alkylamides and phenylpropanoids. Both substance classes are suitable for fingerprint analysis. Figure 4–7 shows fingerprints of E. pallida, E. angustifolia, E. purpurea, and E. atrorubens root. The alkylamide pattern allows identification of E. pallida based on the absence of dodecatetraenic acid isobutylamide and the presence of two characteristic zones (RF 0.25 and 0.6) not seen in any other samples. To differentiate all species, the phenylpropanoids can be used (for details, see Table 4–1). These fingerprints are sufficient to identify individual Echinacea specimens. As we will see later, there are cases of suspected adulteration where it is helpful to also include the information from the alkylamide profile to increase the certainty of the analytical result. Both methods for fingerprint identification of Echinacea provide sufficient separation power for the respective target compounds. In other cases such as separation of flavonoids, this does not apply, and several methods may be required. The most common mobile phase for separation of flavonoids on silica gel is ethyl acetate, formic acid, acetic acid, and water (100:11:11:26). This mobile phase is suitable for initial investigations of herbal drugs. Due to the very large number of flavonoids, it must be expected that several will migrate to the same RF value due to the limited separation power of HPTLC. On the other hand, the technique offers the flexibility of fine-tuning the selectivity with respect to specific regions of the chromatogram. An example is given in Fig. 4–8 Several species of hawthorn (Crataegus spp.) qualify for use as herbal drugs. If those species are to be discriminated, the detection of rutin and vitexin- 2-O“-rhamnoside may be of analytical interest. Using the standard flavonoid mobile phase, which is also recommended by NF19, this task cannot be accomplished, because rutin and vitexin-2- O”-rhamnoside co-elute. Another mobile phase of different selectivity (ethyl acetate, methanol, formic acid, water: 50:3:3:6) achieves the separation of the two compounds in question.2 Regarding the presence of hyperoside in the sample, only the first method is able to make a positive or negative statement. Using the second method, the hyperoside zone may be covered by a stronger fluorescing unidentified blue zone at the same R,F. Box 4–2 Botanical Identity and Fingerprint When looking again at Fig. 4–5, the three Valeriana spp. can be clearly distinguished. The four samples of Valeriana officinalis have all been botanically identified. Their fingerprints are more similar to each other than to those of the other species. By no means, though, can the four fingerprints be labeled as identical. In addition, samples on tracks 1 and 2 from botanical gardens in the United States show greater similarity among themselves than to samples on tracks 3 and 4, which are from sources in Europe. The sample on track 3 contains a zone at middle RF not present in any of the other samples. As a summary of this discussion, there is always a certain range, within which the identity of a sample must be looked at. With this in mind, one may ask the question: What is the “representative” fingerprint of valerian root? Without an answer, let us explore this further. When looking at raw materials of different sources, it may seem logical to expect a greater variety for wild-crafted drugs. Several individual samples of a large batch may be more different than samples of a large batch of cultivated drugs, which can be more easily “maintained” at a certain quality. If good agricultural practice (GAP) is followed, the chemical profile of the material even becomes traceable. On the other hand, if several individual wild-crafted samples are combined and “averaged” for the fingerprint, such average may be more representative for the “true” fingerprint of a given botanical species than that of an average of cultivars. These issues become critical when defining botanical reference material to be used like a “chemical standard” during fingerprint analysis. To arrive at the “true” representative fingerprint for a drug, variability due to climate, geography, nutrition, and harvest time should be included whenever possible. Figure 4-6 HPTLC fingerprints of green tea (Camellia sinensis). (A) Flavonoids. MP: ethyl formate, toluene, formic acid, water (30:1.5:4:3). D: Natural Product reagent, UV 366 nm.Alkaloids. MP: ethyl acetate, methanol, water (20:2.7:2). UV 254 nm. (C) Amino acids. MP: 1-butanol, acetone, acetic acid, water (7:7:2:4). D: ninhydrin, white light. (D) Polyphenols. MP: toluene, acetone, formic acid (9:9:2). D: Fast blue salt B. Track 1, standards; 2, green tea BRM; 3, green tea extract; 4, green tea commercial product. Standards with increasing RF values in rutin, chlorogenic acid, isoquercitrin, astragalin, and caffeic acid (A); theobromin and caffeine (B); aspartic acid, glutamic acid, theanine, and tyrosine (C); epigallocatechin gallate, epigallocatechin, epicatechin gallate, and epicatechin (D). (Courtesy of Wolfram and R. Jorns, Frutarom Ltd., Switzerland.) Figure 4-7 HPTLC fingerprints of various Echinacea root samples. (A) Alkylamide profile. MP: toluene, ethyl acetate, cyclohexane, formic acid (24:6:3:0.9). D: anisaldehyde reagent, white light. (B) Phenylpropanoid profile. MP: ethyl acetate, ethylmethyl ketone, formic acid, water (15:9:3:3). D: Natural Products reagent, UV 366 nm. Track 1, E. pallida; track 2, E. angustifolia; track 3, E. purpurea; track 4, E. atrorubens; track 5 in A, dodecatetraenic acid isobutylamide; track 6 in A, -sitosterol; track 5 in B, caftaric acid, cynarine, chicoric acid; track 6 in B, echinacoside, chlorogenic acid, caffeic acid (increasing RF). The flexibility of using multiple detection techniques is used in the analysis of Ganoderma. Figure 4–9 shows the fingerprints of Reishi mushrooms (Ganoderma lucidum). When the method was developed, very little was known about the chemical constituents of this mushroom. There was neither information about the identity of the components extracted during sample preparation nor about the compounds separated on the chromatogram. Figure 4-8 HPTLC fingerprint of hawthorn (Crataegus spp. leaf and flowers). (A) MP: ethyl acetate, formic acid, acetic acid, water (100:11:11: 26). (B) MP: ethyl acetate, methanol, formic acid, water (50:3:3:6). D: Natural Products reagent, UV 366 nm. Track 1, C. laevigata; track 2, standards (with increasing RF: rutin/vitexin-2O-rhamnoside, chlorogenic acid, hyperoside, vitexin, caffeic acid); track 3, C. monogyna. Figure 4-9 HPTLC fingerprint of Reishi mushrooms (Ganoderma lucidum) under white light (A) or UV 366 nm (B). MP: dichloromethane, methanol (9:1). D: sulfuric acid reagent. Tracks 1 and 2, G. lucidum fruiting body; track 3, G. lucidum mycelium; track 4, G. applanatum fruiting body. The fingerprint still allows distinguishing fruiting body from mycelium of the same species as well as different species from each other.3 The pink zone around R F 0.4 is characteristic for the fruiting body of G. lucidum, also the gray zone just below. The mycelia show a particularly strong zone around R F 0.6. The profile of G. applanatum is distinctly different, and using multiple detections, the difference becomes even more apparent. Defining Identity Standards Whereas synthetic drugs can easily be defined based on their physical properties, botanical drugs are more complex and cannot be described using a single parameter. Even in cases when the chemical composition of a given drug is known, there are no two raw materials that are exactly alike. In many cases, the detailed chemical composition is not known, and the problem of defining identity gets more complicated. A combination of macroscopic and microscopic description together with an organoleptic test is usually sufficient to define a given drug botanically. That means a drug authenticated by a botanist could be expected to serve as reference material for establishing identity. Unfortunately, there is no direct link between the botanical identity and the chromatographic fingerprint of a drug: The constituent profile of the plant material may be affected by several factors as listed in Table 4–2. The following examples will illustrate some problems associated with the selection of representative raw material for a standard fingerprint. In the case of the chaste tree (Vitex agnus-castus), the flavonoid profile can be used for identification. The comparison of 23 samples is presented in Figure 4–10. The overall intensity of the bands in the fingerprints of the samples varies considerably due to quantitative flavonoid differences. It is not problematic to declare all of the samples similar to the reference material (track 2) and therefore acceptable, because the number, sequence, and color of the bands are the same. This statement can be supported even for the “weak” samples on tracks 15, 16, 18, 19, 23, and 24 if they are applied in larger quantities. As we will see later, the situation is completely different when the content of diterpenes is considered. Another example is presented in Figure 4–11. The triterpene glycoside profile of 25 samples of black cohosh (Cimicifuga or Actea racemosa) has been analyzed on two HPTLC plates with actein as reference (tracks 1 and 14). The sample on track 2 can quickly be identified as belonging to a different species, namely C. americana. The fingerprint of that species is distinctly different. The other chromatograms are all similar and characteristic for C. racemosa, but some variability can be observed. Unlike in the previous example, it is not the overall content of triterpene glycosides but the number and sequence of bands that varies. The samples on tracks 5, 18, and 25 deviate more than others from the “typical” pattern due to additional bands in the lower RF region. Based on this difference, these samples should fail the identity test. The reason for the deviation is not yet clear. In a subsequent study, genetic fingerprints of all samples were compared. Genetically, the C. racemosa samples on tracks 5, 18, and 25 also differ distinctly from the rest. The presented approach is not limited to drugs with known constituent profiles but can also be applied successfully for those with unknown constituents. In the case of Reishi mushrooms (see discussion above), the overall similarity of the samples is obvious (Figure 4–12). A completely different problem of defining a fingerprint for identification arises if an herbal drug can consist of plant material of different species. This is the case for hawthorn. As seen in Figure 4–15, the profiles of different species are quite different, and acceptance criteria must be defined broadly so that a given sample would pass based on common features of the fingerprint. Figure 4-10 HPTLC fingerprints of chaste tree fruits (Vitex agnus-castus) of different provenience. MP: tetrahydrofuran, toluene, formic acid, water (8:4:1:0.5). D: Natural Products reagent, UV 366 nm. Tracks 1, 7, 17, and 27, chlorogenic acid; track 2, authenticated reference material; tracks 3, 10, 20, and 30, isoorientin; tracks 4 to 6, 8, 9, and 11 to 13, Argentinian samples; tracks 14 to 16, 18, 19, and 21 to 23, Turkish samples; tracks 24 to 26, 28, 29, 31, and 32, Croatian samples. Figure 4-11 HPTLC fingerprints of various black cohosh samples (Cimicifuga racemosa). MP: toluene, ethyl formate, formic acid (50:30:20). D: anisaldehyde reagent, white light. Tracks 1 and 14, actein; track 2, C. americana; all other tracks, C. racemosa. Figure 4-12 HPTLC fingerprint of Reishi mushrooms (Ganoderma lucidum). MP: dichloromethane, methanol (9:1). D: sulfuric acid reagent. Tracks 1 to 13, G. lucidum fruiting body; tracks 14 to 16, G. lucidum mycelium. Figure 4-13 HPTLC fingerprints of different chemical races of Ashwagandha (Withania somnifera). MP: toluene, ethyl acetate, formic acid (50:15:5). D: sulfuric acid reagent. (A) UV 254 nm prior to derivatization. (B) Derivatized plate, white light. (C) Derivatized plate, UV 366 nm. Track 1, -sitosterol; track 2, Ashwagandha type 1; track 3, Ashwagandha type 2; track 4, Ashwagandha type 3. Figure 4-14 HPTLC fingerprints of various Ashwagandha (Withania somnifera). MP: toluene, ethyl 144 acetate, formic acid (50:15:5). D: sulfuric acid reagent. Tracks 1 and 8, -sitosterol; tracks 2 and 9 to 15, Ashwagandha type 1; tracks 3 to 7, Ashwagandha type 2; track 16, Ashwagandha type 3. Let us now address the question of how a fingerprint chromatogram can be described in reference to an established standard. Establishing Identity (Description) The traditional way of describing an HPTLC fingerprint chromatogram is comparison of the sequence, color, and intensity of the separated zones of the sample and the reference. Among analysts and administrators, the decision about which type of reference should be preferred (chemical or botanical reference material) is still controversial. The European Pharmacopoeia exclusively uses chemical reference substances. There is a tendency in the Chinese Pharmacopoeia to compare fingerprints to botanical reference materials. The United States Pharmacopoeia/National Formulary (USP/NF) favors a combination of both. Recently, the U.S. Pharmacopoeial Forum has published identifications based on comparison against standardized extracts. The use of chemically defined compounds as reference material may be preferred, because such compounds are readily available in suitable purity. Only against known substances can zones of the HPTLC fingerprint be identified, unless the unknown is isolated and externally analyzed. Chemicals provide the option of estimating the quantitative composition of the sample. Compounds that are expected to be present in the investigated samples or that are characteristic for common adulterants should be employed. Unfortunately, high-quality chemical markers are often difficult to find and can be rather expensive. Because they are not naturally present in botanical samples, the practice of using inexpensive chemicals such as dyes (methyl orange, sudan red, fluoresceine) or synthetic drugs (phenazone) as references should be abandoned. For further details, see the discussion of the Rrel value in Chapter 2. What are critical aspects of describing an HPTLC fingerprint? Box 4–3 Variability or Chemotypes? When looking at variability, it easily becomes difficult to set limits beyond which a given fingerprint is regarded as different. If samples of a drug are botanically identified as belonging to the same species, they may be either more or less similar specimens of the same species or representative of different chemical races (chemotypes). Figure 4–13 illustrates the problem for Ashwagandha (Withania somnifera), for which the existence of five chemotypes is known.2 There is little information available about the differences in the constituent profiles of these types. Based on their HPTLC fingerprint, three types can be distinguished, even though the plant samples are macroscopically and microscopically identical and represent the same botanical species. This situation is in accordance with the presence of chemotypes. If any of the three samples had been selected as reference material, the other two would not pass. To determine whether the differences between the three types are insignificant natural variations or characteristics of chemical races, a large number of specimens must be analyzed and compared. Of the 13 samples shown in Figure 4–14 five belong to one type of fingerprint (tracks 3 to 7) and the other seven to a second type (tracks 9 to 15). Tracks 1 and 8 represent chemical references. The samples on tracks 2 and 9 are the same. Within each type of fingerprint there is actually little variability, but the two types are significantly different. The sample on track 16 likely represents the third chemical race, but a number of similar samples would have to be found to substantiate this statement. No indication for the existence of two more chemotypes was found during this investigation.
4
Typical Applications in Herbal Analysis
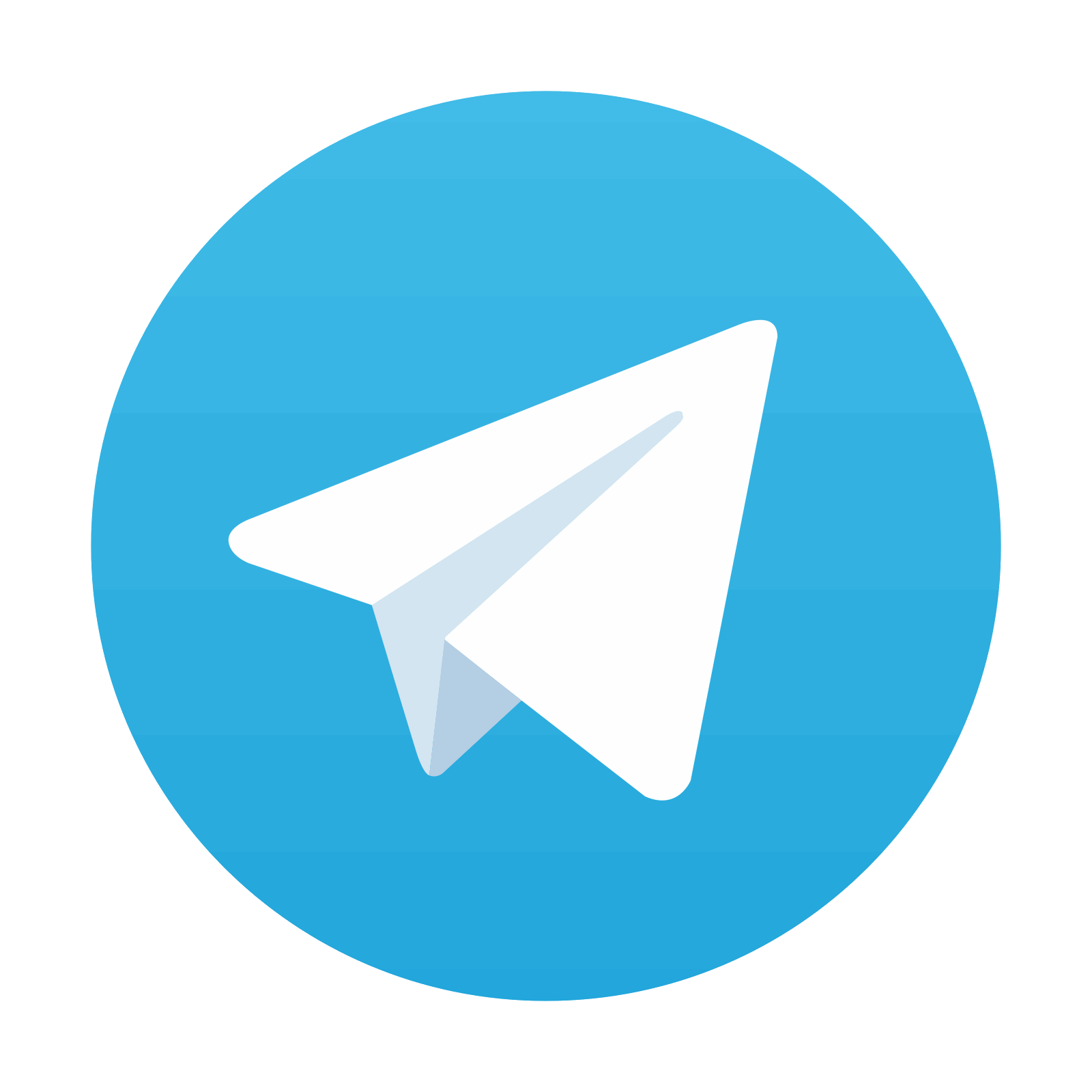
Stay updated, free articles. Join our Telegram channel

Full access? Get Clinical Tree
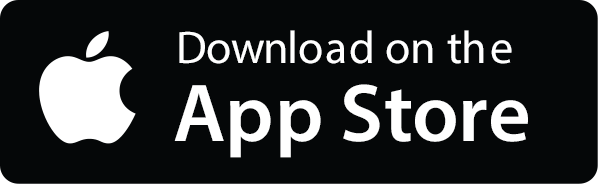
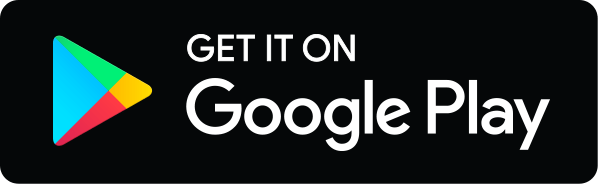