Learning Objectives
- Describe the global epidemiology of tuberculosis and HIV/AIDS
- Understand how tuberculosis is transmitted, diagnosed, and treated
- List the components of the DOTS strategy, the Stop TB Strategy, and the Global Plan to Stop TB
- Discuss the current challenges to controlling tuberculosis in resource-limited settings and the recent advances in diagnosis, treatment, and prevention of tuberculosis
- Describe the history, pathogenesis, diagnosis, and transmission of HIV/AIDS
- Describe the treatment of HIV/AIDS including the prevention and management of opportunistic infections
- Outline the policy and operational issues on treatment rollout, with particular reference to low- and middle-income countries
- Discuss HIV/AIDS prevention strategies and their potential effectiveness and limitations at different stages of the epidemic
- Know where to find additional resources for both tuberculosis and HIV/AIDS
Introduction
Tuberculosis (TB) and human immunodeficiency virus/acquired immunodeficiency syndrome (HIV/AIDS)—one a scourge nearly as old as humankind itself and the other a disease that emerged only 3 decades ago—are responsible for a significant burden of today’s global morbidity and mortality. When occurring separately, each is a challenge to cure or treat, but when occurring together they constitute a deadly pair. In many parts of the world, especially in many resource-limited settings, they are inextricably linked and have required a joint health sector programmatic response. Because of the large overlap that exists between both epidemics in the poorest parts of the globe, these diseases have been grouped in a single chapter. TB and HIV/AIDS are considered first separately and then together in this chapter.
TB is ubiquitous. Approximately a third of the world’s population—more than 2 billion people—is infected with Mycobacterium tuberculosis (MTB). Under ordinary circumstances, about 10% of people infected with MTB develop active TB disease during their lifetime. The World Health Organization (WHO) estimates there were 8.8 million new cases of TB in 2010, with roughly 65% of these cases reported to public health programs and the WHO.1 In the same year there were an estimated 1.5 million deaths due to TB (roughly a quarter of which were HIV-associated), making it the leading cause of death from a curable infectious disease.1,2
The vast majority of TB patients—over 80%—live in just 22 countries in the world. The list of 22 high-TB burden countries is dominated by the resource-limited countries of sub-Saharan Africa and Asia (Table 10-1) with India and China accounting for 40% of the notified cases in 2010. Strengthened TB control efforts and international investments have paid off. The absolute number of TB cases and TB incidence rates have been falling for at least the last 5 years (Figure 10-1).1 In the 2 decades between 1990 and 2010, significant decreases in global prevalence and mortality rates were observed. With approximately 75% of TB cases in resource-limited countries occurring among those in their most economically productive years (between the ages of 15 and 54), the human and economic toll on these countries has been devastating.
1. India |
2. China |
3. Indonesia |
4. Pakistan |
5. Bangladesh |
6. Philippines |
7. South Africa |
8. Democratic Republic of Congo |
9. Ethiopia |
10. Nigeria |
11. Vietnam |
12. Myanmar |
13. Russian Federation |
14. Thailand |
15. Afghanistan |
16. Kenya |
17. Mozambique |
18. Cambodia |
19. Brazil |
20. United Republic of Tanzania |
21. Uganda |
22. Zimbabwe |
The persistence of TB through the years is multifactorial, with key factors being a history of neglect by governments and poorly managed TB control programs, poverty, population growth, and migration. In the last 3 decades, the HIV epidemic has contributed to the rising number of TB cases in sub-Saharan Africa. HIV is a significant risk factor that increases the likelihood of progression from TB infection to disease from 10% over a lifetime to 10% each year. TB is the leading cause of death among HIV-infected individuals, accounting for about a quarter of AIDS deaths worldwide. In some of the worst affected countries in sub-Saharan Africa, over 70% of patients with acid-fast bacilli (AFB) smear-positive pulmonary TB are also infected with HIV.1
The emergence of significant levels of multidrug-resistant TB in some parts of the world has also hampered global efforts to control TB. Strains of TB that are resistant to standard (first-line) anti-TB medications have been documented in every country; however, certain hot spots in Asia and Eastern Europe harbor the greatest burden of drug-resistant TB. Confirming our worst fears, the emergence of TB strains resistant to nearly all anti-TB drugs, termed extensively drug-resistant TB (XDR-TB), has been reported in 83% of the countries that have tested for it.3,4
The global epidemiology of HIV infection is not so different from that of TB. Recurring themes include the heavy case burden borne by sub-Saharan Africa and the relationships between HIV infection and poverty, prior lack of access to effective prevention strategies and treatments, and the fact that HIV is also killing those in their most productive years, in many cases wiping out generations in the hardest hit countries.
About 34 million (31.4 to 35.9 million) people were living with HIV in 2011, more than ever before due to the effects of antiretroviral therapy, which prolongs life. AIDS resulted in the deaths of about 1.7 million (1.5 to 1.9 million) in 2011, and an additional 2.5 (2.2 to 2.8 million) people were newly infected in the same year.5 Sub-Saharan Africa remains the worst affected area, with approximately 23.5 million HIV-infected inhabitants, roughly two-thirds of the total global burden.5 However, the numbers of people infected with HIV in Eastern Europe and Central and East Asia have also grown in the past few years. The proportion of HIV-infected adults who are women continues to increase, reaching 50% globally and 58% in sub-Saharan Africa.5
Heterosexual sex is the primary means of HIV transmission worldwide, although in some regions injection drug use (often combined with an exchange of sex for drugs) is still a major route of transmission. Efforts to control HIV/AIDS were originally focused on preventing new infections, but in the past decade they have included the scale-up of care and treatment programs to provide life-prolonging antiretroviral therapy.
Tuberculosis
TB is caused by the bacterium Mycobacterium tuberculosis. M. bovis, M. caprae, M. africanum, M. microti, M. pinnipedii, and M. canettii are very similar genetically to MTB, and together they comprise the MTB complex.6 Although any of the MTB complex organisms may cause TB, MTB is the most common, especially in the tropics. MTB is an obligate aerobic, non-spore-forming, nonmotile bacillus with large lipid content in its cell wall. MTB grows slowly, with a generation time of approximately 15 to 20 hours as compared with less than 1 hour for most common bacteria. MTB bacilli are referred to as AFB because of the ability of their lipid-rich cell walls to retain red carbolfuchsin stain even after decolorization with acid and alcohol during the Ziehl-Neelsen staining procedure.
MTB is transmitted when a person with active TB coughs, sneezes, or talks and expels MTB bacilli into the air. These respiratory secretions contain droplet nuclei that become aerosolized and can linger in a contained airspace for up to 8 hours. Droplet nuclei are tiny, generally only 5 μm to 10 μm in diameter, which allows them to be transported to the terminal air spaces when inhaled. Once deposited in the terminal air sacs, the MTB bacilli are taken up by alveolar macrophages. Through hematogenous or lymphatic spread or by direct extension, MTB bacteria may spread to virtually any organ in the body. In most cases, the bacteria are “walled off” by macrophages and other cells that contain the infection through granuloma formation. In some cases, particularly in children, initial infection with MTB can result in primary disease that has the hallmarks of hilar or mediastinal lymphadenopathy with or without a visible opacity, the “primary lesion,” in the lung. If successfully contained, the MTB will continue to grow very slowly over the person’s lifetime. This scenario is latent TB infection. Left untreated, MTB will stay dormant and never cause active disease in 90% of people who are otherwise healthy. If a person is HIV infected, this risk of developing active TB increases from a 10% lifetime risk to a 10% annual risk.
If the person’s immune system is not able to contain the MTB infection, the bacteria begin to multiply more rapidly, and the person will develop active TB. In addition to HIV infection, poor nutrition, diabetes mellitus, chronic renal failure, and certain medications (e.g., steroids) can result in varying degrees of immune suppression that will increase the likelihood of progression from latent TB infection to active TB disease. Pulmonary TB is the most common form of the disease, accounting for over 80% of cases (in populations without large numbers of HIV-infected individuals). Extrapulmonary TB can affect any organ other than the lungs, but most commonly affected are the pleura, lymph nodes, spine, genitourinary tract, nervous system, or abdomen. Extrapulmonary TB is more common in HIV-infected individuals and young children.
The classic symptoms of active pulmonary TB are a cough that persists for more than 2 weeks with sputum production and occasional hemoptysis, weight loss, and fevers with night sweats. Chest pain and fatigue are also commonly seen. Fever and weight loss are more common in TB patients who are also HIV-infected. On physical examination, weight loss and tachycardia (due to the fever) may be noted. Respiratory signs are variable and may include crackles, wheezes, or bronchial breathing; alternatively, breath sounds may be completely normal.
Any person presenting to a health care facility with a cough for 2 or more weeks in a TB-endemic country should be suspected of having TB and appropriately evaluated. The patient should submit three sputum samples for smear microscopy (and mycobacterial culture where available). Under ideal conditions (and in areas without a high HIV prevalence), sputum smear microscopy using Ziehl-Neelsen staining will identify approximately 65% of adult pulmonary TB cases.7 Identification of these cases is a high priority because they are the most infectious and therefore responsible for most instances of transmission of TB. Immuno- fluorescence using fluorochrome stain is becoming widely available and is the preferred smear microscopy method. MTB can be identified more quickly using this method because smears can be scanned under a lower magnification. Results of smear microscopy, regardless of the stain used, are recorded based on the number of TB bacilli observed on the slide, which reflects the severity of the disease and the infectiousness of the patient.
Laboratory culture of MTB, considered the gold standard or definitive test for MTB disease, can require up to 8 to 12 weeks using standard culture technique (e.g., solid media with Lowenstein-Jensen media) for MTB growth to be detected. Liquid media using automated equipment such as the BACTEC system (Beckton Dickinson, Sparks, MD) can detect MTB growth as early as 2 to 3 weeks after inoculation, but its expense has limited its availability in resource-limited settings. Because of the lag time to obtain results, mycobacterial culture is not helpful in making rapid individual diagnoses but can be useful in detecting paucibacillary disease in HIV-infected individuals. Although access to mycobacterial culture is increasing in TB-endemic countries, it is rarely available at laboratories outside of academic centers or the country’s national reference center(s).
In December 2010, the WHO endorsed the use of a new automated cartridge-based nucleic acid amplification assay, Xpert MTB/RIF.8 This technology has several distinct advantages over existing diagnostic tests. The assay simultaneously detects the presence of MTB and resistance to rifampin, requires less training and laboratory capacity than culture techniques, and provides a result much more quickly—in about 90 minutes versus weeks to months with current culture technology. A multi-country study involving 1,730 patients demonstrated that Xpert MTB/RIF is both sensitive and specific; it detected 98% of smear-positive and 73% of smear-negative TB cases, and it correctly identified 99% of non-TB cases.9 Its performance in detecting rifampin resistance is equally strong, with the Xpert MTB/RIF assay correctly identifying 98% of cases with rifampin-resistant bacteria.9 Widespread introduction of this test is expected to have a dramatic impact on TB disease control, especially in resource-limited settings with a high HIV prevalence and/or high rates of multidrug resistant TB (MDR-TB). It has been estimated that twice the number of patients with HIV-associated TB will be diagnosed with Xpert MTB/RIF implementation, and three-times as many MDR-TB patients.8 Roll-out and monitoring of this technology is now a main thrust of many national TB control programs and WHO. Cost (especially of the cartridges which are single use) has limited implementation in some settings but as of mid-2012, more than 700 instruments had been procured in 67 countries through concessional pricing.10
Chest radiography is often used to aid in the diagnosis of pulmonary TB when none or only one of the sputum smears examined is positive for AFB or to evaluate concomitant lung pathology in a patient with extrapulmonary TB. Chest x-rays are more helpful in diagnosing TB in children, who, due to their paucibacillary disease and insufficient tussive force for producing sputum, usually have AFB-negative sputum smears. In adults, a chest x-ray may show one or more infiltrates, particularly in the upper lung lobes, with or without cavitation, fibrosis, or retraction. However, no radiographic findings are absolutely diagnostic of pulmonary TB, and even a normal chest x-ray cannot rule out the diagnosis. This is especially true in HIV-infected individuals, where the chest x-ray appearance is often atypical (e.g., normal) in those with severe immunocompromise.
The tuberculin skin test (TST, also referred to as the PPD test because it is composed of purified protein derivative) is rarely used to diagnose TB in adults in resource-limited settings. The TST has both poor sensitivity and specificity for active TB disease (the TST has been shown to be negative in up to 25% of patients with culture-confirmed TB), and its interpretation is complicated in areas where HIV infection is prevalent (increasing the potential for false negative results) and where Bacille Calmette-Guérin (BCG) vaccine is used (increasing the potential for false positive results).
Published data on two commercial ex vivo interferon-gamma release assays (IGRAs)—the T.SPOT.TB (Oxford Immunotec) and QuantiFERON-TB Gold In-Tube (Cellestis/Qiagen)—suggest that these rapid tests have a higher specificity than TST for detecting TB.11,12 These tests detect T-cell responses to two MTB-specific antigens (ESAT-6 and CFP-10) and therefore, unlike the TST, they are not subject to false-positive results in BCG-vaccinated persons. Both tests require only a single blood draw for specimen collection. Evaluation of these tests has focused on their use in detecting latent infection rather than TB disease. The current US recommendation is that IGRAs can be used in place of TST in all situations but that IGRAs are preferred in persons who have been BCG vaccinated or who are in groups that historically have low rates of return for TST reading (e.g., homeless persons). The tuberculin skin test is preferred in young children because of the lack of consistent IGRA use data in this population. For all other groups, there is no preference. Their use is currently limited in the field setting by the need for relatively rapid specimen transport to a laboratory with the appropriate equipment and a trained technician. In addition, their current market-value costs are prohibitive for resource-limited countries.
In the absence of typical laboratory and radiographic findings, a small portion of patients will be diagnosed on the basis of the treating physician’s clinical judgment.
Extrapulmonary TB, representing approximately 20% of all TB cases, presents a greater diagnostic challenge. Without the benefit of the standard sputum smear test for AFB and because of the limited availability of mycobacterial culture, the initial diagnosis is often presumptive, based on clinical findings, and confirmed retrospectively by a positive response to anti-TB treatment. Where available, diagnosis may also rely on the results of specialized diagnostic tools such as ultrasound, biopsy, or aspiration. If extrapulmonary TB is suspected or confirmed, the patient should also be evaluated for pulmonary TB. Children younger than 2 years are at risk for developing serious disseminated forms of TB, specifically miliary or disseminated TB or TB meningitis.
Treatment of TB requires a multidrug regimen for at least 6 months. When taken without interruption, the standard regimen using first-line drugs will cure over 90% of patients with drug-susceptible TB. TB treatment is divided into two phases: the 2-month initial phase, when rapid bacterial killing occurs, and the 4-month continuation phase, when further sterilizing activity occurs. The two most effective drugs against TB in our arsenal are isoniazid and rifampin. Both bactericidal agents, they are used throughout TB treatment. Pyrazinamide, also bactericidal, is only active in an acid environment and therefore only effective during the 2-month initial phase of therapy. A fourth agent, ethambutol, is added to the regimen to prevent the emergence of drug resistance should the primary strain have underlying resistance to any of the other three drugs used.
Treatment regimens based on patient characteristics have been standardized by the WHO (Table 10-2). Different regimens are used for patients suspected of having drug-resistant TB (see “The Problem of Drug Resistance,” later in this chapter). In general, adults and children, regardless of their HIV status, are treated similarly. Whenever possible, fixed-dose combination tablets should be administered because their use will facilitate correct prescribing and patient adherence and simplify drug ordering and stock control. Anti-TB drugs can be obtained by countries at low or no cost from the Global Drug Facility, a program administered by the Stop TB Partnership in Geneva. A 6-month course of anti-TB therapy costs as little as US $30.
Standard Regimens for New TB Patients | Intensive Phase Treatment | Continuation Phase |
---|---|---|
New patients with presumed, or known, to have drug-susceptible TB | 2 months of HRZEa | 4 months of HR |
New patients in settings where the level of isoniazid resistance among new TB cases is high and isoniazid susceptibility testing is not done (or results are not available) before the continuation phase begins | 2 months of HRZEa | 4 months of HRE |
aWHO no longer recommends omission of ethambutol during the intensive phase of treatment for patients with non-cavitary, smear-negative pulmonary or extrapulmonary TB who are known to be HIV-negative. In tuberculous meningitis, ethambutol should be replaced by streptomycin. H = isoniazid, R = rifampicin, Z = pyrazinamide, E = ethambutol, S = streptomycin. Daily dosing during both the intensive and continuation phases is considered optimal but three times weekly during the continuation phase is an acceptable alternative for any new TB patient receiving directly observed therapy. Three times per week therapy during both the intensive and continuation phases is an acceptable alternative if the patient is receiving directly observed therapy and is not living with HIV or living in an HIV-prevalent setting. | ||
Standard Regimens for Previously Treated Patients (depending on the availability of routine drug susceptibility testing (DST) to guide the therapy of individual retreatment patients) | ||
DST | Likelihood of MDR (patient registration groupa) | |
Routinely available for previously treated patients | High (failureb) | Medium or low (relapse, default) |
Rapid molecular-based method | DST results available in 1–2 days confirm or exclude MDR to guide the choice of regimen | |
Conventional method | While awaiting DST resultsc: Empirical MDR regimen Regimen should be modified once DST results are available. | 2HRZES/HRZE/5HRE Regimen should be modified once DST results are available. |
None (interim) | Empirical MDR regimen Regimen should be modified once DST results or DRS datad are available. | 2HRZES/HRZE/5HRE for full course of treatment. Regimen should be modified once DST results or DRS data are available. |
Monitoring patients on therapy to ensure a therapeutic response is achieved is crucial. Patients with positive AFB-sputum smears or mycobacterial cultures should have follow-up sputum smears and cultures examined after the initial phase of therapy and again toward and at the end of therapy. New monitoring recommendations for patients who have a positive Xpert MTB/RIF test are being developed but will likely include similar follow-up.
The BCG vaccine, a live bacterial vaccine first used in 1921, is currently given to about 100 million children each year.13 Determination of its efficacy in preventing various forms of TB has yielded variable and inconsistent results. In a meta-analysis of efficacy studies, BCG vaccine was found to have an overall 51% protective effect against all forms of TB, a 64% protective effect against TB meningitis, and a 78% protective effect against disseminated TB.14 However, this analysis did not exclude studies with “mycobacteria-experienced” patients (i.e., patients who have or may have had prior mycobacterial infection). In mycobacteria-naïve patients (i.e., those without prior mycobacterial infection, such as newborns), BCG efficacy is much higher, at 80%.15
Currently, the WHO recommends administering a single dose of BCG vaccine at birth to all infants living in areas where TB is highly endemic as well as to infants and children at particular risk of exposure to TB in countries with low endemicity.13 Furthermore, BCG vaccination should to be given at birth to all infants regardless of HIV exposure, especially considering the high endemicity of TB in populations with high HIV prevalence. Only those known to be HIV-infected or with severe immunosuppression from another cause should not receive BCG vaccine due to the risk of developing severe or fatal disseminated BCG disease.16 There are no data to support BCG revaccination or booster inoculations.13 BCG’s effectiveness in protecting infants and young children from life-threatening forms of TB suggests that it may be playing a role in preventing even higher rates of TB globally, but it is clearly an insufficient measure to eradicate TB globally.7
The goals of TB control are to reduce morbidity and mortality from the disease until TB no longer poses a threat to the public’s health. To achieve this, it is necessary to ensure the accurate and timely diagnosis and successful treatment of each patient with active TB disease, particularly those capable of transmitting the disease. This will prevent both further transmission to uninfected individuals and the emergence of drug-resistant TB strains.
Historically, attempts to treat TB were highly ineffective and could take several years. Some improvements in lessening the TB burden in industrialized countries were achieved through better nutrition, less crowded living conditions (which decreased the likelihood of close contact to and shared airspace with others with infectious TB), and the sanatoria movement (which removed infectious TB patients from the community); however, TB was still associated with a 50% mortality rate. In the 1950s, tuberculosis treatment was revolutionized by the development of new drugs that, given in combination, cured tuberculosis and eliminated the need for lengthy hospitalizations. However, despite the availability of effective treatment, tuberculosis remained a public health problem.
Years after the development of anti-TB drugs, it became apparent how difficult it is for patients to complete their full course of therapy. The practice of directly observed therapy (DOT), in which a trained individual watches patients take each dose of their medicines, was developed to address this problem. By ensuring regularity and completion of treatment, patients whose treatment is observed have been shown in some settings to have decreased mortality from tuberculosis compared with those whose treatment is not observed.17 In addition, the discovery of the highly effective drug rifampin made it possible to cure the majority of TB cases in 6 to 9 months. The new rifampin-containing regimen became known as short-course therapy. These two dramatic breakthroughs in TB treatment formed the basis of the DOTS approach: directly observed therapy, short course.
However, short-course therapy administered under direct observation was still not enough to control tuberculosis; an even more comprehensive approach was needed. Developed by Dr. Karel Styblo of the International Union Against Tuberculosis and Lung Diseases (IUATLD) based on his work in Africa in the 1980s, the DOTS strategy was launched in 1994 and quickly became the internationally accepted approach to tuberculosis control. Endorsed by the WHO, the DOTS strategy is a five-pronged comprehensive approach that builds on the administration of therapy under direct observation (Table 10-3).
The five components of the DOTS strategy are as follows: |
1. Sustained political commitment |
2. Case detection by quality-assured sputum smear microscopy |
3. Treatment of TB cases with standard short-course chemotherapy regimens under proper case-management conditions, including direct observation of treatment |
4. Uninterrupted supply of quality-assured anti-TB drugs |
5. Recording and reporting system enabling outcome assessment and management of program effectiveness |
The DOTS strategy helped to turn the tide in TB control in the last two decades. However, as the TB epidemic evolved, the DOTS strategy no longer addressed the most pressing issues affecting tuberculosis control: namely, tuberculosis control in HIV-endemic settings and treatment of multidrug-resistant (MDR) TB. In addition, the DOTS strategy has been criticized for placing too much emphasis on treating smear-positive patients, leaving a sizable proportion of patients (i.e., AFB-smear-negative patients, children, and patients with extrapulmonary disease) as a low priority, and therefore there was little support for the detection of smear-negative cases.18 Similarly, sole reliance on the 100-year-old technique of smear microscopy provided little impetus for investment in newer and more sensitive and specific diagnostic measures. Lastly, DOT, once a cornerstone of TB treatment, has also been challenged. Some studies have shown that similar cure rates can be achieved when treatment is self-administered, countering the dogma that DOT is essential to TB control.19,20 Programs rolling out antiretrovirals in resource-limited settings are demonstrating that patients can take long courses of therapy (lifelong in the case of antiretrovirals) with appropriate support and education but without the need for direct observation. Whereas DOT in some settings is arranged to support patients, it has been criticized as punitive and burdensome in other settings. It is becoming clear that no one-size-fits-all approach can be accepted in every situation.
Based on the growing understanding of TB control needs, additional policy framework and clinical guideline documents were developed to provide guidance to TB control programs that build on the DOTS strategy.
Launched on World TB Day in 2006, the Global Plan to Stop TB 2006–2015 provides a road map for accelerated progress toward controlling TB with the goal of significantly reducing the global burden by 2015 (Table 10-4).21 The cost of implementing this plan—US$56 billion—is the largest sum requested for TB control to date. The 2006 Global Plan was updated in 2010.22 The foundation of the plan is the Stop TB Strategy (Table 10-5).23 This new six-point strategy builds on the successes of DOTS while also explicitly addressing the key challenges facing TB such as TB/HIV co-disease, drug-resistant TB, and TB in vulnerable populations. The Stop TB strategy also recognizes the need and includes a call for universal access to high-quality TB care, further TB advocacy to support the development of new tools to prevent, detect, and treat TB, and empowerment of TB patients.
Vision: A TB-free world |
Goal: To dramatically reduce the global burden of TB by 2015 in line with the Millennium Development Goals (MDGs) and the Stop TB Partnership targets |
Objectives |
|
Targets
|
1. Pursue high-quality DOTS expansion and enhancement
|
2. Address TB-HIV, MDR-TB, and the needs of poor and vulnerable populations
|
3. Contribute to health system strengthening based on primary health care
|
4. Engage all care providers
|
5. Empower people with TB and communities through partnership
|
6. Enable and promote research
|
Setting and evaluating success toward agreed on goals has been an essential component of modern TB control. At the heart of the DOTS strategy is the evaluation of patient outcomes to assess the effectiveness of TB control activities. This process—referred to as cohort analysis—allows the determination of, among other outcomes, the treatment success rate of a cohort of patients who started treatment in the same quarter or year. In 1991, the World Health Assembly set the goals of 70% case detection and 85% treatment success as country-level targets to be achieved by 2000, then postponed until 2005.
More recently, the Millennium Development Goals set targets in 2000 for TB control: to reverse the rise in TB incidence globally by 2015 and to halve the 1990 prevalence and death rates in most regions by 2015. Both sets of targets are the benchmarks by which National Tuberculosis Programs (NTPs) now measure their overall success. Furthermore, the Global Plan to Stop TB, as mentioned previously, has set ambitious goals beginning with a vision of a TB-free world (Table 10-4).
Drug-resistant TB is wholly a human-made phenomenon. With TB, the public health consequences of inadequate or incomplete treatment are worse than with no treatment. Drug-resistant strains emerge through spontaneous mutation of the bacilli and will be selected as the dominant strain whenever inappropriate therapy (e.g., therapy with a single agent) is used. Single-drug exposure can occur due to improper prescribing, irregular drug supply, incorrect administration, or poor drug quality. Today, we are paying the price for poor TB control efforts in the past. The rapidly rising rates of drug-resistant TB in many countries are due in large part to previous poor treatment adherence—specifically, a failure of patients to take drugs consistently and/or to complete the prescribed course of treatment. Treatment of drug-resistant TB is more complicated than treatment of drug-susceptible TB; specifically, it is longer, less effective, and vastly more expensive. Consequently, the very ability of public health systems to control TB is threatened by the rising rates of drug-resistant cases.
MDR-TB is defined as MTB resistant to at least isoniazid and rifampin—our two most powerful anti-TB drugs. Treatment of MDR-TB requires treatment with second-line drugs, which are generally from the following classes: aminoglycosides, polypeptides, fluoroquinolones, and carbothioamides; the category also includes cycloserine and para-aminosalicylic acid. Regimens to treat MDR-TB strains should contain at least four drugs to which the organism is known (or highly likely) to be susceptible. A typical initial phase for MDR-TB treatment may contain five or more drugs including an injectable agent that is given for a minimum of 6 months. Under ideal program conditions, MDR-TB cure rates can approach 70%. In general, second-line drugs are less effective and more toxic than first-line drugs. When rifampin can no longer be used because of resistance, treatment must be extended to 18 to 24 months to achieve cure. In addition, treatment of MDR-TB can be 100 times more costly than treatment of drug-susceptible TB.
The WHO estimates there were 290,000 new cases of MDR-TB among the notified TB patients in 2010.1 Only 16% of these, or 46,000, were identified and started on treatment.1 The most recent report of the WHO/IUATLD Global Project on Anti-Tuberculosis Drug Resistance Surveillance evaluated drug-resistant TB data collected from 93 settings between 2002 and 2007.24 As was shown in the three previous surveys, MDR-TB was found in all regions of the world. Based on information compiled throughout the global project (using data from 114 countries and two Special Administrative Regions of China), it is estimated that MDR-TB represents 5% of all TB cases. In addition, patients who had received some treatment for TB in the past were more likely to have drug-resistant TB (an estimated 15% of retreatment cases are MDR-TB), and they have resistance to more drugs than previously untreated patients.24 With a few exceptions, the MDR-TB burden in most countries is stable or declining.
In response to the growing problem of drug-resistant TB, the DOTS-Plus strategy was created in 1998.25 Because the first priority in controlling MDR-TB is preventing its further emergence, a solid DOTS-based program must be in place before instituting DOTS-Plus. However, in MDR-TB hot spots such as Estonia, Latvia, and certain areas of Russia and China, where rates of MDR-TB have already reached high levels, additional measures, including the treatment and cure of resistant cases using second-line drugs, are needed to control MDR-TB. DOTS-Plus includes the designation of specialized treatment centers for MDR-TB, special clinical guidelines for management of MDR-TB patients with second-line drugs, and actions to make second-line drugs available for such treatment. Similar to the Global Drug Facility provision of first-line drugs, the Green Light Committee was established to provide quality-assured second-line drugs at reduced costs to carefully monitored programs.
DOTS-Plus has been shown to be cost effective in a variety of settings, and reasonably high cure rates (approximately 70%) have been achieved.26 By September 2005, just 6 years after its launch, over 10,000 patients with MDR-TB had received treatment through 35 programs in roughly 29 countries. In recognition of the success of DOTS-Plus projects worldwide, the WHO published updated guidelines in 2006 on the management of MDR-TB.26 Compiled by a team of international experts; these guidelines build on the extensive data collected over the years and represent the best available knowledge on the management of MDR-TB. In compliance with the new Global Plan to Stop Tuberculosis to treat all patients with TB, this document represents a significant shift in policy toward MDR-TB management: rather than a luxury reserved for programs with appropriate resources or for those experiencing the highest levels of drug resistance, management of MDR-TB is now an integral activity of an NTP.
In 2006, the emergence of a highly resistant strain of MTB associated with a high mortality rate was described in a report of 53 mostly HIV-infected patients from KwaZulu-Natal, South Africa.27 This new strain—termed XDR-TB—is defined as MTB resistant to isoniazid, rifampin, and to two additional drug classes (fluoroquinolones and either aminoglycosides or capreomycin). Results from a survey of supranational reference laboratories conducted from 2000 to 2004 revealed that 20% of isolates were MDR-TB and 2% were XDR-TB. Population data from industrialized nations, Eastern Europe and Russia, and the Republic of Korea showed that 7%, 14%, and 15% of their reported MDR-TB cases, respectively, were XDR-TB.28 XDR-TB poses a significant obstacle to global TB control; if allowed to propagate, it could result in an epidemic of potentially untreatable TB.
In 2009, clinicians in Iran described their experience with 15 patients with TB that was resistant to all anti-TB medications tested.29 In 2011, a report of four patients in India was also published and garnered significant media attention.30 In both reports, the respective authors referred to these cases as “totally drug resistant TB,” or TDR-TB. Subsequently, the WHO issued a statement that it does not recognize the term totally drug resistant TB because it has not been clearly defined and limitations of current in vitro drug susceptibility testing (DST) for second-line drugs do not allow us to confirm MTB strains as totally drug resistant.31 In contrast, MDR-TB has been well studied, DST methods and interpretation of results outlined, and a consensus reached on its definition. At this time it is unknown how in vitro DST results for the remaining second-line drugs will correlate with clinical response in a patient. Consequently, the WHO advises against using these results to guide individual patient care.
TB control activities must be considered in the context of the country’s health system. If ignored, these factors may thwart efforts to control the disease; however, if leveraged appropriately, they can contribute to the goals of the NTP.
In many countries, particularly in Asia, a substantial private health care sector exists and is the source of care for a sizable proportion of TB patients. If the role played by the private sector is not recognized by the NTP and the opportunity for collaborating with private practitioners is not seized, many patients will be treated outside of the auspices of the NTP without any assurances of quality or adherence to international guidelines for diagnosing and treating TB. As a result, diagnosis may be delayed, which can have an adverse outcome for the patient and will allow continued transmission of TB in the community.
Increasingly, NTP leadership is acknowledging the potential for private sector involvement and building collaborative relationships with private practitioners to support their involvement in NTP activities. A few countries, notably the Philippines and India, have established sophisticated public-private partnership schemes that outline different levels of engagement and collaboration—from simple referral mechanisms to full participation in the diagnostic and treatment activities supervised by the NTP. In most of sub-Saharan Africa, public-private collaborations are newer ventures. This area of involvement is constantly evolving as both the public and private sectors explore potential collaborations based on their specific country and health system context.
Health care access is an issue not only in rural areas, where health outposts may be understaffed or nonexistent, but also in urban areas, where overcrowded health facilities are unable to handle the large patient volume. In response, programs that train and use community health workers to extend the health care system’s reach have been created. Beginning with the WHO-coordinated Community TB Care in Africa project in 1996, the experience and results of numerous models of community-based care have been shared among policymakers, NTPs, community-based organizations, and nongovernmental organizations (NGOs). In rural villages as well as urban slums, together these agencies have contributed to the development of innovative methods of providing patient-centered care and patient- and community-level education as well as contributing to the identification of TB patients—all of which allow the NTP to expand the availability of services that are reflective of the community’s needs. It is essential that all community-based efforts be designed and executed in close collaboration with the NTP.
Most resource-limited countries are experiencing some degree of health care worker shortage. The shortage is most severe in the countries of sub-Saharan Africa, where limited resources significantly contribute to the shortage. Because of poor working conditions, unlivable wages, and concerns about health worker safety, many local physicians and nurses emigrate and seek employment in Europe and the United States, resulting in the well-described brain drain phenomenon. Fueling this problem is active recruitment by these high-income countries to fill some of their own gaps in health care personnel. With only one physician or less per 100,000 population in high-TB burden countries such as Ethiopia, Tanzania, and Uganda, these countries fall short of the WHO-recommended minimum of 20 physicians per 100,000 population.32 In addition, many health care workers who remain in-country are tempted away from clinical care delivery to work for one of the numerous international NGOs in their country (often for a higher salary) to serve in nonclinical management or public health roles. This internal brain drain further compromises the country’s health care delivery capacity. A response to the shortage of health care workers is outside the scope of NTP activities and requires a systematic and sustained directive led by the Ministry of Health involving all health care partners, to which the NTP can contribute through appropriate capacity building and the provision of incentives for retaining TB control clinical staff.
Both poverty and stigma challenge the success of TB control. TB is both caused by and a cause of poverty. On average, a person with TB loses a third of his or her annual income. Additionally, the poor are overrepresented among TB patients. The stigma associated with TB can be powerful and is only worsened in countries where TB is closely linked to HIV/AIDS. Patients with TB have been ostracized from their families and communities, and being witness to this behavior can lead people with a protracted cough to delay seeking care.
Efforts to address both the stigma of TB and its relationship to poverty have been undertaken by governments, NTPs, the WHO, and NGOs. Addressing poverty in TB control requires a multifaceted approach across several sectors to address economic impoverishment and the accompanying vulnerabilities and marginalization. The health system must be willing to undertake a pro-poor and equity-based approach to address the special needs of the most disadvantaged groups.33 This approach, coupled with public education activities to address stigma (such as media campaigns and the use of celebrity TB survivors, which have been successful in some countries), is needed to address these significant challenges to TB control efforts.
Like HIV, diabetes increases one’s risk for progressing from latent TB infection to TB disease. Another similarity is the negative feedback one disease has on the other, with TB worsening glycemic control and diabetes worsening the clinical course of TB. The prevalence of diabetes in some TB-endemic communities, especially in some Asian countries, is making diabetes the most common risk factor for developing TB disease. As has occurred with HIV-associated TB, a coordinated response supporting collaboration between TB and diabetes services and providers is essential. Mechanisms for collaboration and policy development for patients with TB and diabetes are being developed based on the models for integrated TB and HIV care, and bidirectional screening for both diseases is recommended. The WHO and the Union Against Tuberculosis and Lung Disease have published guidance for a collaborative framework for the management and control of TB and diabetes to begin to address this emerging issue.34
Both sputum smear microscopy and the TST, the two 100-year-old TB diagnostic tests most commonly used in resource-limited settings, lack the sensitivity and specificity desirable in a diagnostic test. Mycobacterial culture, albeit a more sensitive test, is still an imperfect tool because of the delay in the availability of results. And although the introduction of Xpert MTB/RIF holds promise to increase TB case detection and retention dramatically (due to the short turnaround time to results), cost is likely to limit its use; currently, it is reserved for high-risk patients, namely HIV-infected and those suspected of having drug-resistant TB in specialized urban centers. The ideal TB diagnostic test would be a simple and accurate point-of-care (POC) technology that could be used at remote health centers without the need for sophisticated laboratory expertise or equipment.
Recent efforts on advancing TB diagnostics offer hope. Scientific research in this area has been renewed by the contributions from disciplines such as molecular biology, nanotechnology, immunology, and genomics. This work is shifting diagnostic development from the biologic to the molecular level.35 Use of specimens other than sputum that are easier to collect (e.g., urine or stool) are being explored. Accessibility, affordability, and sensitivity are the three key characteristics that will determine the impact of future diagnostics for TB.35
A faster and simpler therapy for TB is essential to achieve successful TB control globally. Sequencing of the MTB genome in 1998 led to advanced efforts to find novel ways to combat this pathogen. Recent public-private collaborations, led by the Global Alliance for TB Drug Development, have ensured swift movement of potential compounds through the development pipeline. New compounds, such as the diarylquinoline, bedaquiline,36 recently approved by the Food and Drug Administration, and delamanid OPC-67683 hold promise for effective, shorter TB treatment with no evidence of cross-resistance.37,38 Specifically, the addition of delamanid to standard therapy was shown in a randomized controlled trial to improve sputum culture conversion at 2 months in patients with MDR-TB.38 In addition, results from a 2012 phase 2 clinical trial conducted in South Africa demonstrated excellent early bactericidal activity of a new combination therapy using the new TB drug candidate PA-824 plus moxifloxacin and pyrazinamide.39
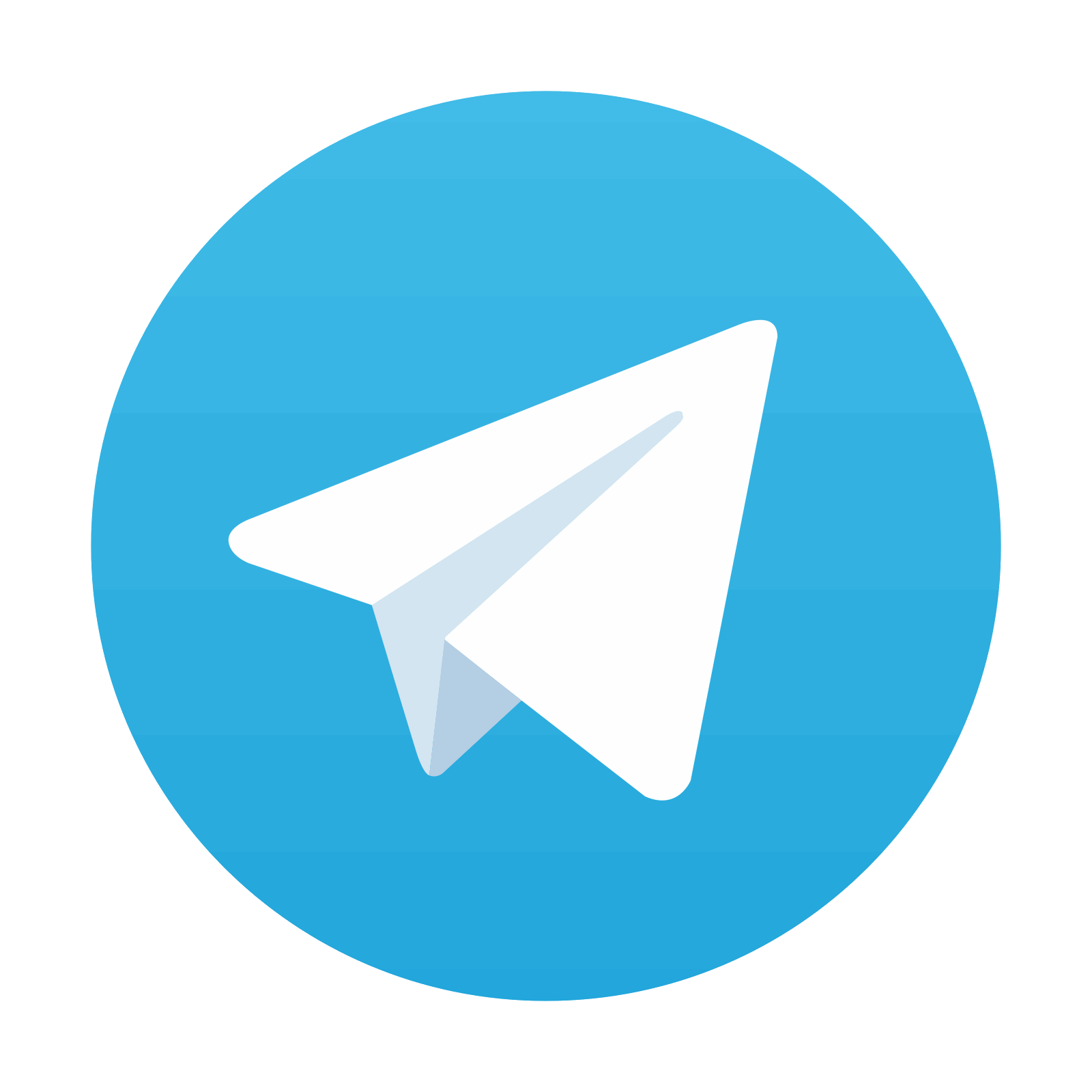
Stay updated, free articles. Join our Telegram channel

Full access? Get Clinical Tree
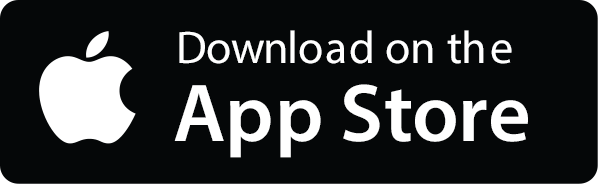
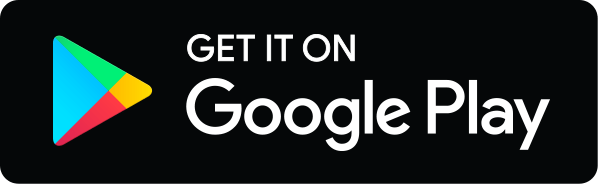
