Fig. 3.1
Schematic Representation of TRPV1 LTD. a Presynaptic TRPV1 LTD, while mediated at the presynaptic terminal depressing glutamate neurotransmitter release, requires formation of postsynaptic 12-HPETE in order to activate TRPV1. 12-HPETE is formed by the enzyme 12-lipoxygenase (12-LO) using arachidonic acid (AA) as a metabolic precursor, which is liberated subsequent to mGluR1 activation. Once TRPV1 is activated presynaptically, it results in activation of the protein phosphatase calcineurin, which through an unknown mechanism (?) inhibits neurotransmission (as illustrated with an ‘x’). b Postsynaptic LTD results from endocytosis of AMPA glutamate receptors. Postsynaptic mGluR5 and TRPV1 activation along with anandamide (AEA) formation are required for this LTD. While the exact pathway of AEA formation and its effect is not completely known, AEA usually is formed from the conversion of metabolic precursor N-arachidonoyl phosphatidylethanolamine (NAPE) phospholipase D, and once formed AEA likely targets TRPV1. TRPV1 then potentially results in the activation of calcineurin (?), which through clathrin-dependent mechanisms induces endocytosis of AMPA receptors. It should be noted that the exact location of TRPV1 in both cases is not specifically known as TRPV1 could be expressed by the plasma membrane or by intracellular membranes regulating internal calcium release
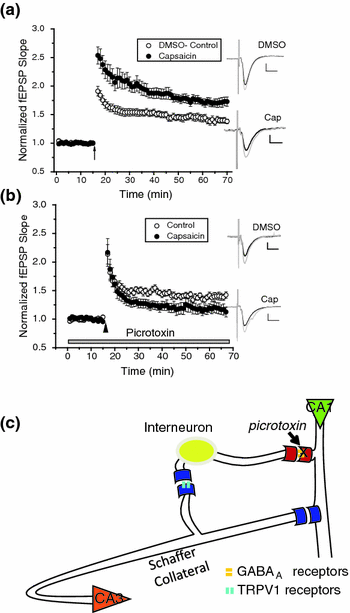
Fig. 3.2
TRPV1-induced enhancement of CA1 pyramidal cell long-term potentiation (LTP) is blocked by GABAA antagonist picrotoxin. a The TRPV1 agonist capsaicin (1 μM), significantly increased (p < 0.05; n = 14) theta burst (arrow)-induced LTP as compared to dimethyl sulfoxide (DMSO) vehicle control (0.1 % DMSO; n = 20), as measured using field excitatory postsynaptic potentials (fEPSPs). b Picrotoxin (100 μM) significantly blocked the capsaicin-induced enhancement of LTP (p < 0.05; n = 8) compared to control (n = 17). Insets: an average of 15 traces either just before (black) or 28–30 min after (gray) conditioning stimulus. Scale Bars: 0.25 mV, 10 ms. Figure 3.2 parts a and b were reprinted with permission from Bennion et al. ( 2011). c A schematic model representing the effect of picrotoxin in the hippocampal circuit. CA3 pyramidal cell axons form the Schaffer Collateral pathway innervate the CA1 subfield and synapse onto both CA1 pyramidal cells and CA1 inhibitory interneurons. Interneurons use GABA as an inhibitory neurotransmitter that binds to postsynaptic GABAA receptors, which mediate fast inhibition onto pyramidal cells. TRPV1 located presynaptically at the Schaffer Collateral synapse onto the interneurons induce LTD when activated (see Fig. 3.1a) and thus reduce inhibition from the interneurons to the CA1 pyramidal cells, known as disinhibition. In the presence of capsaicin to induce TRPV1 LTD, LTP of CA1 pyramidal cells is enhanced (see a this figure). In the presence of picrotoxin, which eliminates the effect of the interneurons from the hippocampal circuit, this capsaicin-induced enhancement of CA1 LTP is blocked (see b this figure); indicating TRPV1 acting through the interneuron pathway is required for enhanced CA1 pyramidal cell LTP
One interesting conundrum concerning the TRPV1-mediated LTD is that while normally presynaptic TRPV1 enhances glutamate neurotransmission, as TRPV1 is calcium-permeable and thus enhances presynaptic calcium and transmitter release levels, in this case TRPV1 depressed transmitter release. It turns out that TRPV-mediated LTD requires activation of the phosphatase calcineurin by TRPV1, in order to induce LTD. This data will be more fully addressed later on.
Collectively, these data suggest that TRPV1 is normally involved in NMDA receptor-dependent LTP. Since TRPV1 plays a role in plasticity, TRPV1 could be used as a neuromodulator target in the effort to potentially enhance plasticity and therefore theoretically enhance memory formation.
3.3.2 Hippocampal Long-Term Depression
Recent studies examined TRPV1 modulation of various forms of hippocampal LTD following the demonstration of TRPV1 modulation of NMDA receptor-dependent LTP in CA1 pyramidal cells. Hippocampal LTD is a mechanism thought to depotentiate synapses that have undergone LTP and could be used to eliminate harmful memories or allow for new memory formation, among other potential options. These studies identified that TRPV1 is indeed likely involved in several types of hippocampal LTD, including (1) in the dentate gyrus, (2) mGluR-dependent CA1 LTD, and (3) NMDA-dependent CA1 LTD, which will be discussed in turn.
A novel postsynaptic LTD mediated by TRPV1 was identified in the dentate gyrus (Chavez et al. 2010). Dentate gyrus granule cells innervated by the medial perforant pathway underwent postsynaptic LTD via clathrin-dependent internalization of AMPA receptors in response to capsaicin application or a 1 Hz pairing protocol that was TRPV1-dependent. TRPV1-induced LTD in the dentate gyrus was confirmed using TRPV1−/− mice, supporting the study’s pharmacological data (see model in Fig. 3.1b). Interestingly, in contrast to CA1, where presynaptic TRPV1 activation required postsynaptic mGluR1 and 12-HPETE formation; in the dentate gyrus, postsynaptic TRPV1 activation required postsynaptic mGluR5 and anandamide production. Therefore, TRPV1-dependent LTD in the hippocampus can differ fairly dramatically depending on the hippocampal subfield. However, similar to CA1, dentate gyrus LTD was also calcineurin-dependent, suggesting calcineurin may play a key role in TRPV1 signaling in the hippocampus.
Another major type of hippocampal synaptic plasticity is mGluR-dependent LTD, in contrast to NMDA-dependent LTD. Regarding CA1 mGluR-dependent LTD, previous studies proposed that the initial acute depression of it was mediated by a cannabinoid receptor 1 (CB1)–independent endocannabinoid pathway (Rouach and Nicoll 2003), though what this endocannabinoid pathway could be, was not known. One report suggests this CB1-independent acute depression could be TRPV1 induced. In this case, initiating mGluR-dependent LTD using the type I mGluR agonist DHPG while examining the acute depression phase, the TRPV1 antagonist capsazepine actually caused an increase in the depression (Bennion et al. 2011). This implied a role for TRPV1, though capsaicin application caused no further change in the depression. Taken together, however, the fact that capsazepine caused an increase in depression suggests that when activated normally, TRPV1 mitigates some of the acute depression of mGluR-dependent LTD, and since capsaicin had no effect, TRPV1 is already probably close to being fully activated in response to DHPG application. Type I mGluRs activated by DHPG thus likely result in the production of a TRPV1 agonist, such as anandamide or 12-HPETE, to activate TRPV1. Thus when TRPV1 is blocked, the acute depression is even greater by eliminating the mitigating effect of TRPV1 on depressed glutamate neurotransmission. The TRPV1 involved in acute DHPG-induced depression is most likely present on CA1 pyramidal cells rather than the TRPV1 modulating interneuron activity, as the acute depression in the presence of capsazepine is not altered by the GABAA antagonist picrotoxin. While TRPV1 altered acute short-term depression, it was not involved in true LTD or long-lasting depression of duration greater than 15 min.
Regarding CA1 NMDA-dependent LTD, in one report, TRPV1 agonist capsaicin (1 mM) caused a reduction of LTD induced by 3 Hz stimulation, an effect that was eliminated by capsazepine (Li et al. 2008), suggesting that TRPV1 can modulate CA1 NMDA-dependent LTD. However, while this study enticingly suggests that TRPV1 is involved in CA1 LTD, the concentration of capsaicin used can have off-target effects, including increasing intracellular calcium in a TRPV1-indendent manner. A subsequent study using a lower, more TRPV1-specific concentration of capsaicin (1–10 μM) and 5 Hz stimulation noted no change in LTD (Bennion et al. 2011). While several variables differed between these two reports and could be the reason for the discrepancy, no conclusive evidence can be reached at this time regarding TRPV1 involvement in CA1 NMDA-dependent LTD.
Collectively, while TRPV1 involvement in CA1 NMDA-dependent LTD is debatable, TRPV1 is likely involved in short-term acute depression of mGluR-dependent LTD, and strong evidence supports it mediating a novel form of LTD in the CA1 and dentate gyrus.
3.3.3 Plasticity in Other CNS Regions
After TRPV1-dependent synaptic plasticity was reported in the hippocampus, TRPV1-dependent types of plasticity were identified in other brain regions as well. One such example is in the superior colliculus, which is a classic model used to examine the synaptic refinement or pruning that occurs in the developing visual system via mechanisms such as LTD, which can cause a weakening or loss of synapses. High-frequency stimulus-induced LTD of the superior colliculus, as measured using field excitatory postsynaptic potentials, was blocked with TRPV1 antagonists and mimicked using TRPV1 agonists (Maione et al. 2009). The developmental timing of the ability to induce LTD also coincided with temporal TRPV1 expression as measured using TRPV1 antibodies. TRPV1 receptor expression and TRPV1-dependent LTD were both present in young animals but absent in adults. Juvenile TRPV1 expression occurs in GABAergic cells of the superior colliculus and on glutamatergic retinal inputs to the superior colliculus where TRPV1 is likely presynaptically expressed in the latter, similar to glutamatergic input to CA1 stratum radiatum interneurons. It appears that this plasticity modulates the GABA pathway to induce LTD as GABA receptor blockers eliminated this LTD. In summary, TRPV1 could be developmentally involved in the CNS by regulating synaptic pruning, the elimination of incorrect and unnecessary synapses.
In addition to the superior colliculus, the amygdala, which is part of the limbic system along with the hippocampus, has also altered plasticity in the presence of capsaicin (Zschenderlein et al. 2011). Interestingly, TRPV1-induced alteration of amygdalar LTP was dependent on the type of anesthetic used on the animal, with ether producing decreased LTP and isoflurane producing increased LTP. These results were confirmed using TRPV1−/− mice. In addition, in the presence of ether, the TRPV1 agonist N-oleoyldopamine also depressed amygdala lateral nucleus LTP (Kulisch and Albrecht 2013). In this case stress was also examined, and research demonstrated that TRPV1 activation by N-oleoyldopamine inhibited stress-induced depression of LTP by forced swim test. The exact mechanism of this TRPV1 pathway and how TRPV1 altered amygdala LTP was not completely determined, though nitric oxide synthase was required. Along with modulation of amygdala LTP, TRPV1 was also required for a type of amygdala LTD. In the extended amygdala, neurons from the bed nucleus of the stria terminalis exhibit TRPV1-dependent LTD (Puente et al. 2011). This postsynaptic form of plasticity required the activation of postsynaptic TRPV1 via anandamide, which was formed as a result of postsynaptic mGluR5 stimulation, very similar to TRPV1-dependent LTD in the dentate gyrus (Chavez et al. 2010).
The midbrain also demonstrates plasticity via TRPV1 in both the nucleus accumbens and the substantia nigra. The nucleus accumbens is especially important for reward and reward-dependent learning, which can be modulated by the drugs of abuse that alter normal plasticity, leading to addictive states. Addiction results in behaviors and actions that are carried out inspite of the negative consequences. In medium spiny neurons of the nucleus accumbens, mGluR5-induced endocannabinoid production is required for postsynaptic TRPV1-mediated LTD initiated by either low-frequency stimulus or the type I mGluR agonist DHPG (Grueter et al. 2010). This LTD required activation of both mGluR5 and TRPV1 receptors as demonstrated using various antagonists and TRPV1−/− mice. TRPV1 induced postsynaptic AMPA receptor endocytosis, again similar to TRPV1-LTD in the hippocampus and amygdala. Interestingly, the injection of cocaine 24 h prior to the physiological experiments altered this plasticity by blocking it. This suggests that TRPV1 plays a role synaptically and behaviorally in the reward pathways of the brain and could be involved in addiction at the nucleus accumbens level. In this study TRPV1−/− mice also demonstrated enhanced locomotion in response to cocaine injection, supporting a role for TRPV1 in motor pathways. Finally, dopamine release in the nucleus accumbens can also be enhanced by TRPV1 activation of dopamine neurons in the ventral tegmental area via glutamate, which activates the nucleus accumbens following noxious stimulation (Marinelli et al. 2005). This further supports a role for TRPV1 in the midbrain reward pathways.
As a note, it is worth mentioning that a form of TRPV-like mediated LTD is exhibited in leeches, an invertebrate. This LTD occurs at nociceptive synapses and is dependent on presynaptic TRPV-like receptors activated by postsynaptically produced endocannabinoids and is also calcineurin-dependent (Yuan and Burrell 2010, 2012). This type of plasticity is extremely similar to presynaptic TRPV1-mediated LTD in hippocampal interneurons (Gibson et al. 2008) and is also dependent on presynaptic protein synthesis and postsynaptic synthesis of mRNA and protein (Yuan and Burrell 2013). While the requirement of transcription and translation in mammalian CNS TRPV1 plasticity has yet to be investigated, it is likely similar to leeches as many forms of plasticity are dependent on protein synthesis.
In summary, there is now substantial data supporting the role of TRPV1 in synaptic plasticity in divergent brain regions, including acting as a mediator of LTD and a modulator of LTP. Therefore, TRPV1 has the potential to be a widespread regulator of adaptive brain function via synaptic alterations in brain areas required for learning and memory, visual development, and the reward pathways, including drug-induced alterations in reward plasticity. The involvement of TRPV1 in synaptic plasticity is summarized in Table 3.1.
Table 3.1
TRPV1 involvement in the modulation and mediation of mammalian CNS synaptic plasticity
Plasticity type | Location | TRPV1- pre or post | Effect | mGluR | eCB | Induction | Signal molecule | Reference | |
---|---|---|---|---|---|---|---|---|---|
Modulate | LTP | Hippocampal CA1 pyramidal cells | Enhanced LTP | HFS (100 Hz) | ND | Li et al. (2008) | |||
Hippocampal CA1 pyramidal cells | Postsynaptic likely | Enhanced LTP mediated by disinhibition via interneurons | Theta burst, HFS | ND | Bennion et al. (2011) | ||||
Hippocampal CA1 pyramidal cells | Reduced LTP in TRPV1−/− | HFS (100 Hz) | ND | Marsch et al. (2007) | |||||
Hippocampal CA1 pyramidal cells | Reduced LTP in TRPV1−/− rescued to WT with GABA antagonist | HFS (100 Hz) | ND | Brown et al. (2013) | |||||
Amygdala, lateral nucleus | Pre and Postsynaptic, alters glutamate | Depressed LTP in ether, enhanced LTP in isoflurane | Anandamide likely | HFS (100 Hz), capsaicin | Nitric Oxide Synthase | Zschenderlein et al.(2011) | |||
Amygdala, lateral nucleus | Pre and Postsynaptic, alters glutamate | Depressed LTP (ether), blocked stress-induced impairment of LTP | N-oleoyldopamine | HFS (100 Hz), w/w out N-oleoyldopamine | Kulisch and Albrecht (2013) | ||||
LTD | Hippocampal CA1 pyramidal cells | Reduction in LTD | LFS (3 Hz) | ND | Li et al. (2008 ) | ||||
Hippocampal CA1 pyramidal cells | Postsynaptic likely | No change in LTD | LFS (5 Hz) | ND | Bennion et. al. (2011) | ||||
mGluR-LTD | Hippocampal CA1 pyramidal cells | Postsynaptic likely | Mitigate acute depression, no effect on LTD | Type I mGluRs | DHPG | ND | Bennion et al. (2011) | ||
Mediate | LTD | Hippocampal CA1 interneurons | Presynaptic | Depressed glutamate release | mGluR1 | 12-HPETE | HFS, TRPV1 agonists | Calcineurin, Ca2+ | |
Superior colliculus | Presynaptic likely | Induce LTD, GABA system involved | ND | Anandamide or 12-HPETE possibly | HFS (50 Hz), TRPV1 agonist | ND | Maione et al. (2009) | ||
Hippocampal dentate gyrus | Postsynaptic | AMPA receptor endocytosis | mGluR5 | Anandamide | LFS, TRPV1 agonists | Calcineurin, Ca2+ | Chavez et al. (2010) | ||
Nucleus accumbens- MSNs | Postsynaptic | AMPA receptor endocytosis | mGluR5 | ND | LFS (3 Hz); Capsaicin | Ca2+ | Grueter et al. (2010) | ||
Amygdala, stria terminalis | Postsynaptic | ND | mGluR5 | Anandamide | LFS (10 Hz) | Ca2+ | Puente et al. (2011) |
3.4 Endogenous TRPV1 Activity
3.4.1 Endogenous CNS TRPV1 Ligands
As changes in pH and temperature are relatively small and likely insufficient to activate TRPV1 in the CNS, lipid-based ligands are the most likely endogenous activators. Various fatty acid derivatives that activate TRPV1 are endogenously produced in the CNS. These ligands are thought not to be released by standard vesicle chemical neurotransmission mechanisms but are locally acting signal molecules, functioning in paracrine/autocrine or intracellular messenger fashion. Endogenously produced TRPV1 CNS ligands include several C18 N-acylethanolamines, such as endovanilloid N-oleoylethanolamine (Movahed et al. 2005), N-arachidonoyl-dopamine, 12-HPETE, and anandamide (Tóth et al. 2009). These all bind TRPV1 in the hippocampus (Ahern 2003; Al-Hayani et al. 2001; Gibson et al. 2008; Huang et al. 2002). 12-HPETE is also produced in the hippocampus by stimulation protocols used for synaptic plasticity (Feinmark et al. 2003). Therefore, theoretically these all could be involved in the induction of TRPV1 plasticity or TRPV1-induced behavior in vivo. For example, as activation of endogenous anandamide (Al-Hayani et al. 2001) or N-arachidonoyl-dopamine (NADA) (Huang et al. 2002) in the hippocampus both increase paired-pulse depression of population spikes, and 12-HPETE mediates CA1 stratum radiatum interneuron LTD (Gibson et al. 2008), hippocampal TRPV1 could likely influence synaptic plasticity in vivo/in vitro. Enzymes that form anandamide and 12-HPETE are respectively N-acyl-phosphatidylethanolamine-specific phospholipase D (NAPE-PLD) (Di Marzo et al. 1994) and 12-lipoxygenase (Hwang et al. 2000). mRNA for these enzymes are expressed by both hippocampal pyramidal cells and inhibitory interneurons as demonstrated using RT-PCR (Merrill et al. 2012), suggesting that both cells express enzymes to produce TRPV1 ligands and thus modulate hippocampal TRPV1 activity. That interneurons can produce endocannabinoids in addition to pyramidal cells was also recently demonstrated electrophysiologically (Péterfi et al. 2012). Immunocytochemical localization of TRPV1 alongside biosynthesis or biodegradation enzymes of TRPV1 endogenous ligands in the hippocampus and cerebellum support an endogenous role of TRPV1 in the brain (Cristino et al. 2008). Involvement of TRPV1 in normal, in vivo/in vitro hippocampal function is also supported by the fact that TRPV1−/− mice show reduced CA1 LTP as compared to littermate controls (Marsch et al. 2007). In addition to the hippocampus, in the superior colliculus, TRPV1 expression was co-localized in young animals (P14-28) to NAPE-PLD and 12-lipoxygenase (Maione et al. 2009). The demonstration of TRPV1 expression near the enzymes that produce TRPV1 endovanilloid ligands illustrates that all the components necessary for TRPV1 to be endogenously active in vivo present in specific regions. It also strongly suggests that TRPV1 could be used as a target of pharmaceutical agents that alter TRPV1 activity in order to achieve a particular benefit.
3.4.2 Tonic Activation
Similar to the PNS, where tonic activation of TRPV1 seems to be especially important for pain and inflammatory conditions (Premkumar and Abooj 2013), endogenously produced ligands also appear to tonically activate CNS TRPV1, where it alters basal neurotransmission. For example, in the hippocampus, where the previously described TRPV1-mediated interneuron LTD occurs in response to mGluR1 activation followed by 12-HPETE formation, there is actually an increase in glutamate neurotransmission in response to mGluR1 antagonists, suggesting that the interneurons were tonically inhibited by TRPV1 via mGluR1 (see Fig. 3.3). In other brain regions TRPV1 is also tonically activated, such as in the substantia nigra, in response to tonic anandamide release (Marinelli et al. 2003), and in the brain stem, where TRPV1 antagonists altered increased activity in the rostral ventromedial medulla (Starowicz et al. 2007). While the significance of tonic action of CNS TRPV1 is currently unknown, the cannabinoid receptor CB1 is also often tonically activated (Cachope 2012) and it could be a shared property of cannabinoid/endovanilloid receptors.
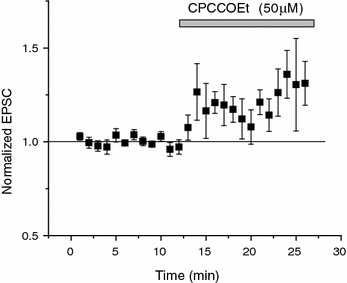
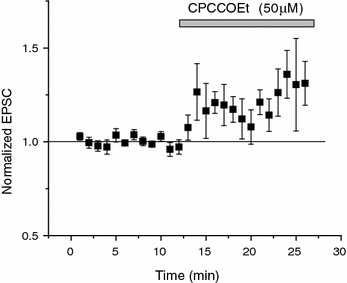
Fig. 3.3
TRPV1 is likely tonically activated in CA1 causing basal depression of neurotransmitter release onto hippocampal stratum radiatum interneurons. The metabotropic glutamate receptor 1 (mGluR1) antagonist CPCCOEt significantly increases (p < 0.05; n = 7) excitatory postsynaptic currents (EPSCs). EPSCs increased by 27.6 ± 3.8 % (STE) at 10–15 min after drug application. As mGluR1 is required to synthesize 12-HPETE to induce LTD via TRPV1 (Gibson et al. 2008), this suggests there is tonic activation of mGluR1 and thus TRPV1 stimulation under basal conditions in vitro, which is uncovered by blocking mGluR1
3.5 TRPV1 Signaling in CNS
TRPV1 can be expressed both presynaptically and postsynaptically, and depending on the specific synapse, it can either increase or decrease neurotransmission. Therefore, TRPV1 may have various signaling roles and mechanisms that are unique in different brain regions. As stated previously, presynaptic TRPV1 normally enhances glutamate neurotransmission by increasing presynaptic calcium (Musella et al. 2009; Marinelli et al. 2003; Marinelli et al. 2007; Doyle et al. 2002). However, presynaptic TRPV1 can also depress neurotransmission, as in hippocampal LTD (Gibson et al. 2008). While initially contradictory, it is possible that in this case calcium entry via TRPV1 could be activating a calcium-sensitive pathway to depress transmitter release. Supportive of this was evidence that blockade of the protein phosphatase calcineurin, also known as protein phosphatase 3/protein phosphatase 2B, eliminated both high-frequency stimulus-induced and TRPV1 agonist-induced LTD in interneurons (Jensen and Edwards 2012). Thus TRPV1 mediated this LTD or depression of neurotransmission via activation of a calcineurin-dependent pathway (see Fig. 3.1a). Supportive of this are the many studies that have linked calcineurin activity to synaptic plasticity, including presynaptic plasticity (Heifets et al. 2008; Zeng et al. 2001). In addition, a link between TRPV1 and calcineurin in depressing neurotransmission was identified in dorsal root ganglion cells where TRPV1-mediated Ca2+ currents activated calcineurin, causing reduced neurotransmitter release (Wu et al. 2005). In this study dephosphorylation via calcineurin had the dual effect of both decreasing activity of voltage-gated calcium channels in the presynaptic terminal and endocytosing them. It is possible that a similar mechanism mediates depression of neurotransmission via calcineurin in the hippocampus as well. These data also suggest that TRPV1 is closely coupled with calcineurin in the presynaptic terminal. While the exact location of TRPV1 is not known, as it could be expressed in the plasma membrane or on intracellular membranes, TRPV1 activates calcineurin that likely depresses voltage-gated calcium channels and reduces neurotransmission overall at the synapse. Another possibility is that TRPV1 mediates activation of a kinase such as PKA, which alters the vesicle release machinery of the cell through RIM1α, as has been reported for CB1 plasticity (Marinelli et al. 2007). This seems less likely based on our unpublished data using the PKA/kinase antagonist staurosporine that did not block LTD. In regions such as the superior colliculus, presynaptic TRPV1 may be functioning in a similar fashion, though to date the presynaptic TRPV1 signaling pathway is not known in other brain regions.
Interestingly, postsynaptic TRPV1 also mediates a signal cascade triggered by calcineurin in the dentate gyrus (Chavez et al. 2010) (see Fig. 3.1b). Therefore, calcineurin is a potential common downstream target of TRPV1 and is likely closely associated with TRPV1 by a protein binding domain such as AKAP (Schnizler et al. 2008) in a type of supramolecular signaling complex. This would allow calcineurin activation by calcium, which is specifically entering the synapse terminal via TRPV1, similar to sensory neurons (Schnizler et al. 2008). Finally, calcineurin desensitizes TRPV1 (Mohapatra and Nau 2005), suggesting this could serve as a kind of negative feedback system as well; however, this has yet to be investigated specifically in the CNS. While much is known about TRPV1 in the PNS (or through expression systems, e.g., HEK cells), including its reversible phosphorylation via PKA/PKC and calcineurin, along with factors involved in sensitization, desensitization, and TRPV1 activation (Cortright and Szallasi 2004; Mohapatra and Nau 2005; Zhang et al. 2007; Mandadi et al. 2004; Woo et al. 2008), much less is known regarding signaling in the CNS, which remains to be fully explored.
3.6 TRPV1 in CNS Behavior
3.6.1 Stress and Anxiety
In the CNS, TRPV1 seems especially relevant in stress- and anxiety-related behaviors. TRPV1 has recently been reviewed as an important stress-response protein in the CNS (Ho et al. 2012). TRPV1−/− mice exhibit several behavioral changes, including reduced anxiety, fear conditioning (amygdala-dependent), and stress sensitization (Marsch et al. 2007; Santos et al. 2008), while wild-type mice infused with capsaicin exhibit anxiety-like behaviors (Hakimizadeh et al. 2012). TRPV1−/− mice had less anxiety in elevated maze, and light-dark tests and less freezing response following fear conditioning in tone and stress sensitization compared to wild-type littermates (Marsch et al. 2007). Similarly, following the infusion of TRPV1 antagonists in the ventral hippocampus or by intraperitoneal injection, rats and mice exhibit reduced anxiety in the elevated plus-maze test (Santos et al. 2008; Kasckow et al. 2004; Micale et al. 2008). Microinjection of the CB1/TRPV1 agonist anandamide into the prefrontal cortex suggests that TRPV1 plays a role in anxiety, with CB1 activation being more anxiolytic and TRPV1 activation being more anxiogenic (Rubino et al. 2008). In this study, higher dose injections of the nonhydrolysable anandamide analog methanandamide produced an anxiogenic effect, which was blocked by TRPV1 antagonist capsazepine. URB597, the selective inhibitor of fatty acid amide hydrolase, the anandamide degrading enzyme, which induces increased endogenous anandamide, was somewhat anxiogenic as well. These data indicate a role for TRPV1 in anxiety in various brain regions.
Regarding stressful events, TRPV1 activation proved to enhance spatial memory retrieval in response to stress (Li et al. 2008). While TRPV1 agonist capsaicin application by itself, either via intrahippocampal or intragastric infusion, did not enhance memory as measured using a Morris water maze behavioral assay, capsaicin did eliminate the deficits in spatial memory associated with stress from forced swim. This suggests that TRPV1 may play a protective role on short-term memory following stress. Similar findings were also demonstrated in the lateral nucleus of the amygdala where TRPV1 agonists eliminate stress-induced decreases in LTP (Kulisch and Albrecht 2013). Therefore, TRPV1 appears to play a significant role in anxiety and stress in the CNS.
3.6.2 Alcohol Consumption
As TRPV1 is also activated by ethanol, it has the potential to induce CNS ethanol-mediated behaviors. Indeed, TRPV1−/− mice had a higher preference for and consumption of ethanol (Blednov and Harris 2009) than wild-type mice. In addition, TRPV1−/− mice had a faster recovery from ethanol-induced motor incoordination and loss of righting reflex than wild-type mice. Injection of TRPV1 antagonist into wild-type mice resulted in the same phenotype as the TRPV1−/− mice. Decreased ethanol avoidance, associated with no change in consumption of sweet and bitter, was also noted by others (Ellingson et al. 2009), supporting this general find. It should be highlighted that while ethanol consumption in TRPV1−/− mice could still be mediated by lack of TRPV1 in the PNS trigeminal nerve in the oral cavity, effects on motor coordination are likely mediated by TRPV1 in the midbrain.
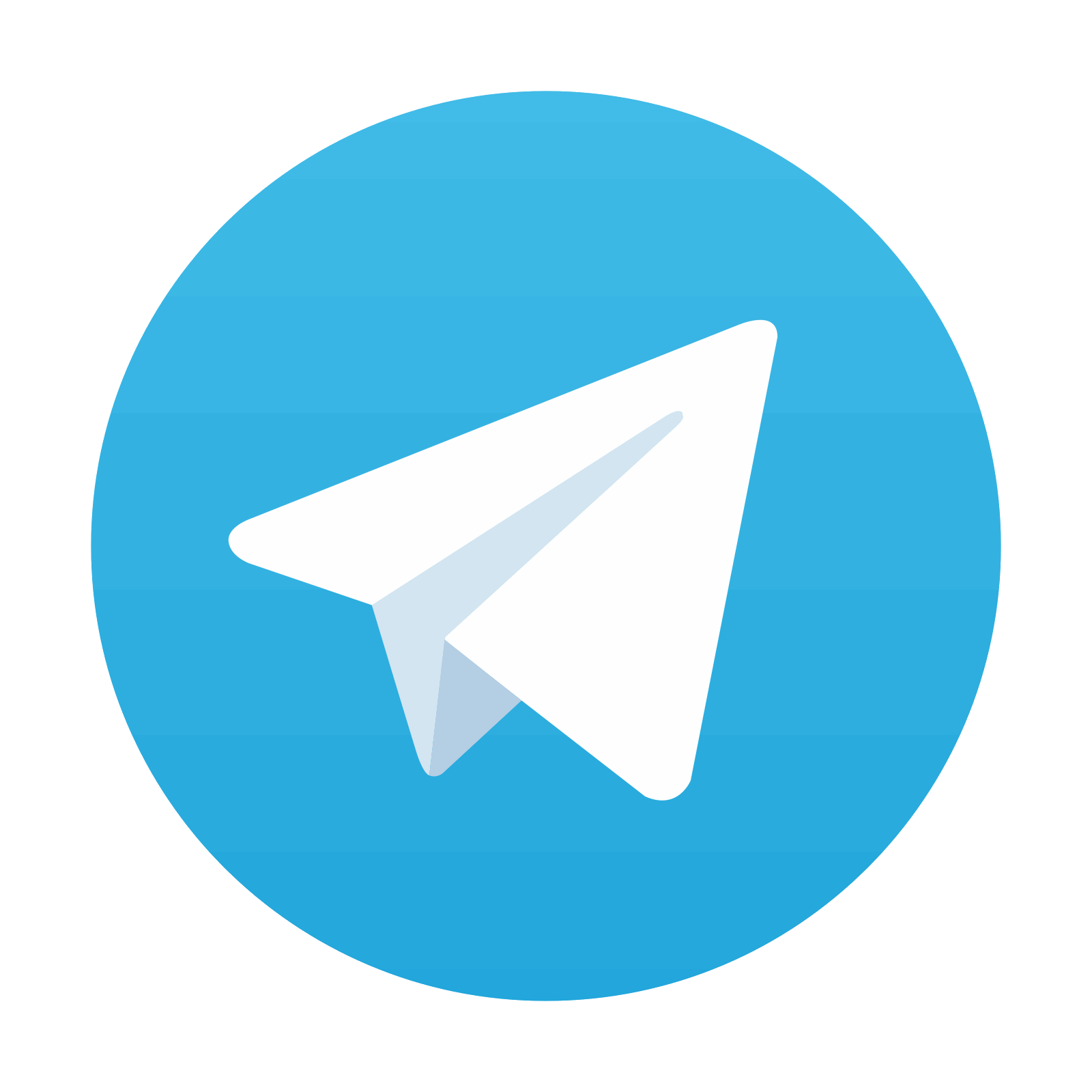
Stay updated, free articles. Join our Telegram channel

Full access? Get Clinical Tree
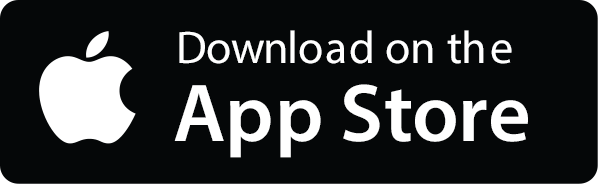
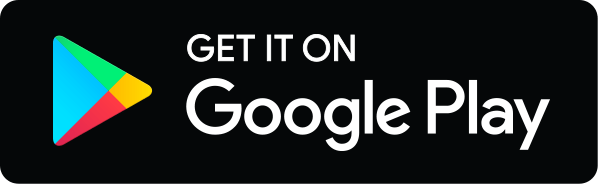