7 Trauma and multiple injury
Trauma epidemiology
However, experience of trauma cannot be extrapolated from other countries to predict events or outcomes. For example, in the UK, RTAs, falls and interpersonal violence account for the majority of major trauma (Fig. 7.1). Fewer than 1 in 10 patients with major trauma have penetrating injury, and this is usually caused by knives. In the USA, approximately 20% of the population owns a gun, and RTA deaths are matched by firearm injuries. Staggeringly, having a gun in the home increases the risk of homicide three-fold, and that of suicide five-fold; and for 15–24-year-olds, these figures increase by a factor of 10. A recent UK study found that the total number of homicides over a 2-year period for a population of 0.8 million was similar to that seen in a single day among the same-sized population of many American cities. In so-called developed countries, annually, 1 person in 50 will be involved in an RTA. Of these, 1% will die, 10% will need hospital treatment and 25% will be temporarily disabled.
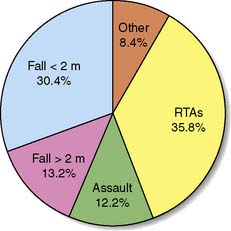
Fig. 7.1 Relative proportions of causes of trauma deaths.
(Data kindly provided by the Scottish Trauma Audit Group 1992–1997.)
Injury biomechanics and accident prevention
where E = energy transfer, T = time, V = volume of tissue, and C = tissue factors (a constant).
These physical principles underpin strategies of accident prevention and protection. Obviously, reducing the chance of direct contact helps; separating pedestrians and traffic is the single most important factor in reducing pedestrian injury rates. This is illustrated by the fact that, in the US, less than 2% of traffic fatalities are pedestrians, whereas in the UK they account for 36% of the total. In a similar fashion, the central reservation barriers on motorways dramatically reduce the chances of high-speed head-on collisions (Fig. 7.2).
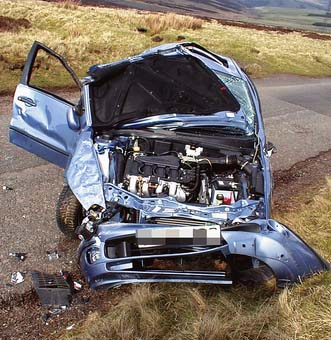
Fig. 7.2 Car involved in a high-speed, head-on collision, showing the magnitude of structural damage.
Contact factors can be minimized by vehicle design: crumple zones, energy-absorbing materials, preventing the ejection of passengers from the vehicle and reducing intrusion into the passenger compartment. For the occupants, seatbelts, airbags, collapsible steering columns and soft fascia compartments enable contact deceleration to take place over a longer time period, reducing the potential for injury (Fig. 7.3). Properly used, seatbelts reduce the risk of death/serious injury by 45%. Airbags further reduce the risk of death by 10% for belted drivers, and by 20% for unbelted front-seat passengers, but may not provide protection from side-impact events, or if the vehicle rolls over.
Summary Box 7.1 Accident features associated with major trauma
Wounds
Classification and production
• Abrasions or grazes. These are caused by the tangential application of blunt force. Dirt is often ingrained in the surface layers of skin, with the risk of short-term infection and, if untreated, later permanent ‘tattooing’. The abrasion’s site and nature may give useful clues as to the direction and magnitude of injury forces.
• Contusions, ecchymoses or bruises. These result from blunt force disrupting superficial capillaries. The overlying skin is intact. When small blood vessels are involved, a large collection of blood (haematoma) may develop. It is impossible to tell the age of a bruise accurately by its colour, but if it is yellow (caused by breakdown of haemoglobin pigment to biliverdin and bilirubin), the bruise is likely to be at least 18 hours old.
• Lacerations. Blunt forces tear, shear or crush skin and soft tissues, producing lacerations. The wound edges are irregular and often abraded, contused and crushed, as are the surrounding tissues.
• Incised wounds or ‘cuts’. These are produced by sharp edges, such as knives or glass shards, and have characteristically clean edges with clear margins. The greatest dimension of an incised wound is its length (cf. puncture wound).
• Puncture wounds. Sharp points or edges produce puncture wounds, in which the greatest dimension is the depth. When the wound pierces a body cavity, it is ‘penetrating’; if it passes through a viscus, it is ‘perforating’.
Gunshot wounds
Gunshot wound data (Fig. 7.4) highlight the gulf between UK and US practice. In the US, deaths from gunshot wounds are the fourth leading cause of years of potential life lost before the age of 65. Guns are used in over 60% of suicides and 70% of all homicides. Non-fatal gunshot wounds outnumber fatal ones two- to three-fold.
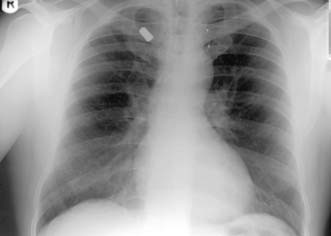
Fig. 7.4 Chest X-ray showing a bullet to the right of the upper mediastinum.
(Courtesy of Miss Kate Wilson.)
Shotgun events are relatively more common in the UK than handgun or rifle injuries (http://www.crimeandjustice. org.uk/). The muzzle velocity of these weapons is relatively high, but dissipation of the shot and air resistance on the pellets quickly decrease their velocity and limit the wounding potential (Fig. 7.5). These weapons are lethal at close range but, unless ‘choked’, are relatively less wounding at greater distances, where they tend to cause superficial injury to skin and subcutaneous tissues.
Falls
where g = gravitational constant (9.8 m/s) and h = height fallen in meters
The body’s position during landing affects the contact area and the propagation of energy since, if the same force is dissipated over a larger area, there is less force per unit area and hence less damage (Figs 7.6 and 7.7). Feet-first falls involve a relatively small area of contact, but deceleration forces can be reduced by flexing the knees and hips. Regardless of the position on landing, however, for falls > 5 m there is a high incidence of deceleration injuries to intrathoracic and intra-abdominal structures, particularly where these are relatively immobile or tethered: for example, the aortic root and the mesenteric arteries. Overall, falls on to an unyielding surface from 15–20 m are associated with a greater than 50% mortality. Nevertheless, bizarre descriptions of survival from high falls do exist. There are well documented accounts of individuals surviving after falling from aircraft onto trees and deep snow. Clothing may also play a role in slowing the rate of fall: in 1885, Miss Sarah Ann Henley survived after a fall from the Clifton suspension bridge (∼︀75 m) as her hooped crinolines acted as a parachute and the tide was out so that the landing surface was thick mud.
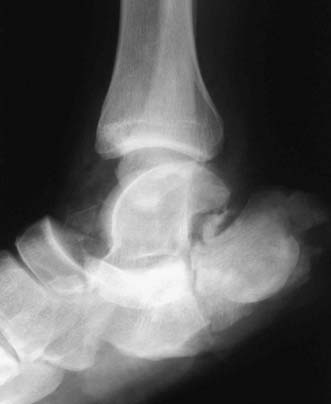
Fig. 7.6 Lateral view of ankle and grossly comminuted fracture of calcaneum, involving the subtalar joint.
The mechanism of injury was a fall on to the feet from 15 metres.
Injury severity assessment
The second type of classification is physiological. The best-known physiological scoring system is the Glasgow Coma Scale (Table 7.1), which is used to assess the neurological state of injured patients objectively, and which also has prognostic value. The Glasgow Coma score (GCS), in conjunction with two other physiological recordings, systolic blood pressure and respiratory rate, can be used to produce the ‘Revised Trauma Score’. Although widely used, physiological scoring systems have intrinsic problems. Some patients with severe injury may not be identified initially, usually because the assessment has been performed before detectable physiological compromise has had time to occur. The system may also overestimate injury severity if physiological changes occur (due, for example, to alcohol) that are not reflected in the measured parameters, or which modify these factors. In addition, no allowance is made for factors such as comorbid features e.g. underlying cardiac or pulmonary disease or medications.
Eyes open | |
4 | |
3 | |
2 | |
1 | |
Best motor response | |
To verbal command | |
6 | |
To painful stimulus | |
5 | |
4 | |
3 | |
2 | |
1 | |
Best verbal response | |
5 | |
4 | |
3 | |
2 | |
![]() Stay updated, free articles. Join our Telegram channel![]() Full access? Get Clinical Tree![]() ![]() ![]() |