Chapter 6 Responses to cellular injury
CELLULAR INJURY

Causative agents and processes
A wide range of possible agents or circumstances result in cellular injury (Fig. 6.1). These could be categorised according to the nature of the injurious agent, the cellular target, the pattern of cellular reaction or mode of cell death. The sequence of agent, target and mode will be uniform, but some injurious agents have variable effects depending on concentration, duration or other contributory influences such as co-existent disease. Some examples are given in Table 6.1. Major types of cellular injury include:
Table 6.1 Examples of causes of cellular injury and their mode of action
Example agent | Mode of action |
---|---|
Trauma (e.g. road traffic accident) | Mechanical disruption of tissue |
Carbon monoxide inhalation | Prevents oxygen transport |
Contact with strong acid | Coagulates tissue proteins |
Paracetamol overdose | Metabolites bind to liver cell proteins and lipoproteins |
Bacterial infections | Toxins and enzymes |
Ionising radiation (e.g. X-rays) | Damage to DNA |
Physical agents
Most physical agents cause passive cell destruction by gross membrane disruption or catastrophic functional impairment. Trauma and thermal injury cause cell death by disrupting cells and denaturing proteins, and also cause local vascular thrombosis with consequent tissue ischaemia or infarction (Ch. 8). Freezing damages cells mechanically because their membranes are perforated by ice crystals. Missile injury combines the effects of trauma and heat; much energy is dissipated into tissues around the track. Blast injuries are the result of shearing forces, where structures of differing density and mobility are moved with respect to one another; traumatic amputation is a gross example. Microwaves (wavelengths in the range from 1 mm to 1 m) cause thermal injury. Laser light falls into two broad categories: relatively low energy produces tissue heating, with coagulation for example; higher energy light breaks intramolecular bonds by a photochemical reaction, and effectively vaporises tissue. Ionising radiation is considered on p. 115.
Chemical and biological agents
Cells may be injured by contact with drugs and other chemicals; the latter may include enzymes and toxins secreted by micro-organisms. This category of agents can give rise to the full range of modes of death.
Infectious organisms
The mechanisms of tissue damage produced by infectious organisms are varied, but with many bacteria it is their metabolic products or secretions that are harmful (Ch. 3). Thus, the host cells receive a chemical insult that may be toxic to their metabolism or membrane integrity. The mode of cell death generally induces an acute inflammatory response, which may be damaging to adjacent cells; organisms that do this are called pyogenic. In contrast, bacterial endotoxin (lipopolysaccharide) induces apoptosis with different pathological consequences. Intracellular agents such as viruses often result in the physical rupture of infected cells, but with some viruses such as hepatitis B (Ch. 16) local tissue damage may result from host immune reactions. Therefore, the cellular response to injury caused by infections will depend on a combination of the damage inflicted directly by the agent and indirectly as a result of the host response to the agent.
Blockage of metabolic pathways
Loss of growth factor or hormonal influence
Many cells rely on growth factors for their survival. Typically, these bind to growth factor receptors spanning the plasma membrane, triggering an intracellular cascade, often via a tyrosine kinase. This pathway can fail or be blocked at many points including growth factor deficit, receptor loss or blockade, or tyrosine kinase inhibitor (e.g. imatinib) (Ch. 4); affected cells may undergo apoptosis. Similar consequences can follow hormone withdrawal, as either a physiological response or part of a disease process. If widespread in an organ, it will shrink (atrophy).
Ischaemia and reperfusion injury
Impaired blood flow (Ch. 8) causes inadequate oxygen delivery to cells. Mitochondrial production of ATP will cease, and anaerobic glycolysis will result in acidosis due to the accumulation of lactate. The acidosis promotes calcium influx. Cells in different organs vary widely in their vulnerability to oxygen deprivation; those with high metabolic activity such as cortical neurones and cardiac myocytes will be most affected.
Free radicals
Free radicals can be generated by two principal mechanisms:
The consequences of free radical formation include the following:
The clinicopathological events involving free radicals include:
Cells irreversibly damaged by free radicals are deleted, generally by apoptosis.
Failure of membrane integrity
Cell membrane damage is one of the consequences of complement activation (Ch. 9); some of the end products of the complement cascade have cytolytic activity. Another effector of cytolysis is perforin, a mediator of lymphocyte cytotoxicity that causes damage to the cell membrane of the target cells such as those infected by viruses. Incidental membrane tears or perforations can be repaired very quickly, so do not necessarily result in cell death.
DNA damage or loss
Damage to DNA results primarily from reactive oxygen species attack, for example following ionising radiation (p. 117). Damage may not be evident immediately; dividing cells are more susceptible. Cell populations that are constantly dividing (i.e. labile cells such as intestinal epithelium and haemopoietic cells) are soon affected by a dose of radiation sufficient to alter their DNA. Other cell populations may require a growth or metabolic stimulus before the DNA damage is revealed. Since non-lethal DNA damage may be inherited by daughter cells, a clone of transformed cells with abnormal growth characteristics may be formed; this is the process of neoplastic transformation that results in tumours (Ch. 11).
Mechanisms of cell death
There are two distinct mechanisms by which cells die: necrosis and apoptosis. However, there are also other cellular deaths combining features of both these processes. Discussion of cell death is further complicated by a lack of uniform nomenclature; some authors use the term necrosis to denote cell death by any cellular mechanism, but more often it is used to describe a specific mechanism. Although there are usually particular triggers for one process or another, there are some situations where apoptosis follows a lower dose or shorter duration of insult while necrosis occurs above that threshold. Mechanisms of cell death involve defined metabolic pathways. Consequently, cell death processes may be amenable to therapeutic interventions.
Necrosis

All these pathways eventually lead to rupture of the plasma membrane and spillage of cell contents, but this is not the end of the sequence. Some of the contents released are immunostimulatory, for example heat shock proteins and purine metabolites. These provoke the inflammatory response (Ch. 10), which paves the way for repair.
Several distinct morphological types of necrosis are recognised:
The type of tissue and nature of the causative agent determine the type of necrosis.
Coagulative necrosis
Coagulative necrosis is the commonest form of necrosis and can occur in most organs. Following devitalisation, the cells retain their outline as their proteins coagulate and metabolic activity ceases. The gross appearance will depend partly on the cause of cell death, and in particular on any vascular alteration such as dilatation or cessation of flow. Initially, the tissue texture will be normal or firm, but later it may become soft as a result of digestion by macrophages. This can have disastrous consequences in necrosis of the myocardium following infarction, as there is a risk of ventricular rupture (Ch. 13).
Microscopic examination of an area of necrosis shows a variable appearance depending on the duration. In the first few hours, there will be no discernable abnormality. Subsequently, there will be progressive loss of nuclear staining until it ceases to be haematoxyphilic; this is accompanied by loss of cytoplasmic detail (Fig. 6.2). The collagenous stroma is more resistant to dissolution. The result is that, histologically, the tissue retains a faint outline of its structure until such time as the damaged area is removed by phagocytosis (or sloughed off a surface), and is then repaired or regenerated. The presence of necrotic tissue usually evokes an inflammatory response; this is independent of the initiating cause of the necrosis.
Gangrene
Gangrene is necrosis with putrefaction of the tissues, sometimes as a result of the action of certain bacteria, notably clostridia. The affected tissues appear black because of the deposition of iron sulphide from degraded haemoglobin. Thus, ischaemic necrosis of the distal part of a limb may proceed to gangrene if complicated by an appropriate infection. As clostridia are very common in the bowel, intestinal necrosis is particularly liable to proceed to gangrene; it can occur as a complication of appendicitis, or incarceration of a hernia if the blood supply is impeded. These are examples of ‘wet’ gangrene. In contrast, ‘dry’ gangrene is usually seen in the toes, as a result of gradual arterial or small vessel obstruction in atherosclerosis or diabetes mellitus, respectively. In time, a line of demarcation develops between the gangrenous and adjacent viable tissues.
Fibrinoid necrosis
In the context of malignant hypertension (Ch. 13), arterioles are under such pressure that there is necrosis of the smooth muscle wall. This allows seepage of plasma into the media with consequent deposition of fibrin. The appearance is termed fibrinoid necrosis. With haematoxylin and eosin staining, the vessel wall is a homogeneous bright red. Fibrinoid necrosis is sometimes a misnomer because the element of necrosis is inconspicuous or absent. Nevertheless, the histological appearance is distinctive and its close resemblance to necrotic tissue perpetuates the name of this lesion.
Apoptosis
Apoptosis is quite different from necrosis (Table 6.2); indeed, it includes suppression of necrosis. It is an energy-dependent process for deletion of unwanted individual cells. Apoptosis is involved in morphogenesis (Ch. 5), and is the mechanism for controlling organ size. Unwanted or defective cells also undergo apoptosis; thus, lymphocyte proliferation in germinal centres and the thymus is followed by apoptosis of unwanted cells. Factors controlling apoptosis include substances outside the cell and internal metabolic pathways:
Table 6.2 Comparison of cell death by apoptosis and necrosis
Feature | Apoptosis | Necrosis |
---|---|---|
Induction | May be induced by physiological or pathological stimuli | Invariably due to pathological injury |
Extent | Single cells | Cell groups |
Biochemical events | Energy-dependent fragmentation of DNA by endogenous endonucleases | Energy failure |
Impairment or cessation of ion homeostasis | ||
Lysosomes intact | Lysosomes leak lytic enzymes | |
Cell membrane integrity | Maintained | Lost |
Morphology | Cell shrinkage and fragmentation to form apoptotic bodies with dense chromatin | Cell swelling and lysis |
Inflammatory response | None | Usual |
Fate of dead cells | Ingested (phagocytosed) by neighbouring cells | Ingested (phagocytosed) by neutrophil polymorphs and macrophages |
Outcome | Cell elimination | Defence, and preparation for repair |
Exposure to inducers or withdrawal of inhibitors acts via the bcl-2 protein family, which then inhibit or activate the death pathway, resulting in activation of initiator and executioner caspases. Alternatively, activation of the plasma membrane receptor Fas (CD95) by its ligand bypasses bcl-2 to activate other caspases (Fig. 6.3). These result in degradation of the cytoskeletal framework, fragmentation of DNA and loss of mitochondrial function. The nucleus shrinks (pyknosis) and fragments (karyorrhexis). The cell shrinks, retaining an intact plasma membrane (Fig. 6.4), but alteration of this membrane rapidly induces phagocytosis. Dead cells not phagocytosed fragment into smaller membrane-bound apoptotic bodies. There is no inflammatory reaction to apoptotic cells, probably because the cell membrane is intact. Various diseases are associated with reduced or increased apoptosis.

Fig. 6.4 Apoptosis. Histology of skin from a case of graft-versus-host disease (Ch. 9) in which there is individual cell death (arrowed) in the epidermis as a result of immune injury.
Reduced apoptosis
The product of the p53 gene checks the integrity of the genome before mitosis; defective cells are switched to apoptosis instead. In contrast, bcl-2 protein inhibits apoptosis. Therefore, loss of p53 function or excess bcl-2 expression may result in failure of initiation of apoptosis with resulting cell accumulation; both these defects are important in neoplasia (Ch. 11). Autoimmune disease (Ch. 9) might reflect failure of induction of apoptosis in lymphoid cells directed against host antigens; in systemic lupus erythematosus, alterations are reported in the Fas lymphocyte receptor. Some viruses enhance their survival by inhibiting apoptosis of cells they infect, and latent infection by Epstein–Barr virus upregulates bcl-2.
Increased apoptosis
Diseases in which increased apoptosis is probably important include acquired immune deficiency syndrome (AIDS), neurodegenerative disorders and anaemia of chronic disorders (Ch. 23). In AIDS, human immunodeficiency virus proteins may activate CD4 on uninfected T-helper lymphocytes, inducing apoptosis with resulting immunodepletion. Apoptosis is the usual mode of cell death in exposure to ionising radiation (p. 117).
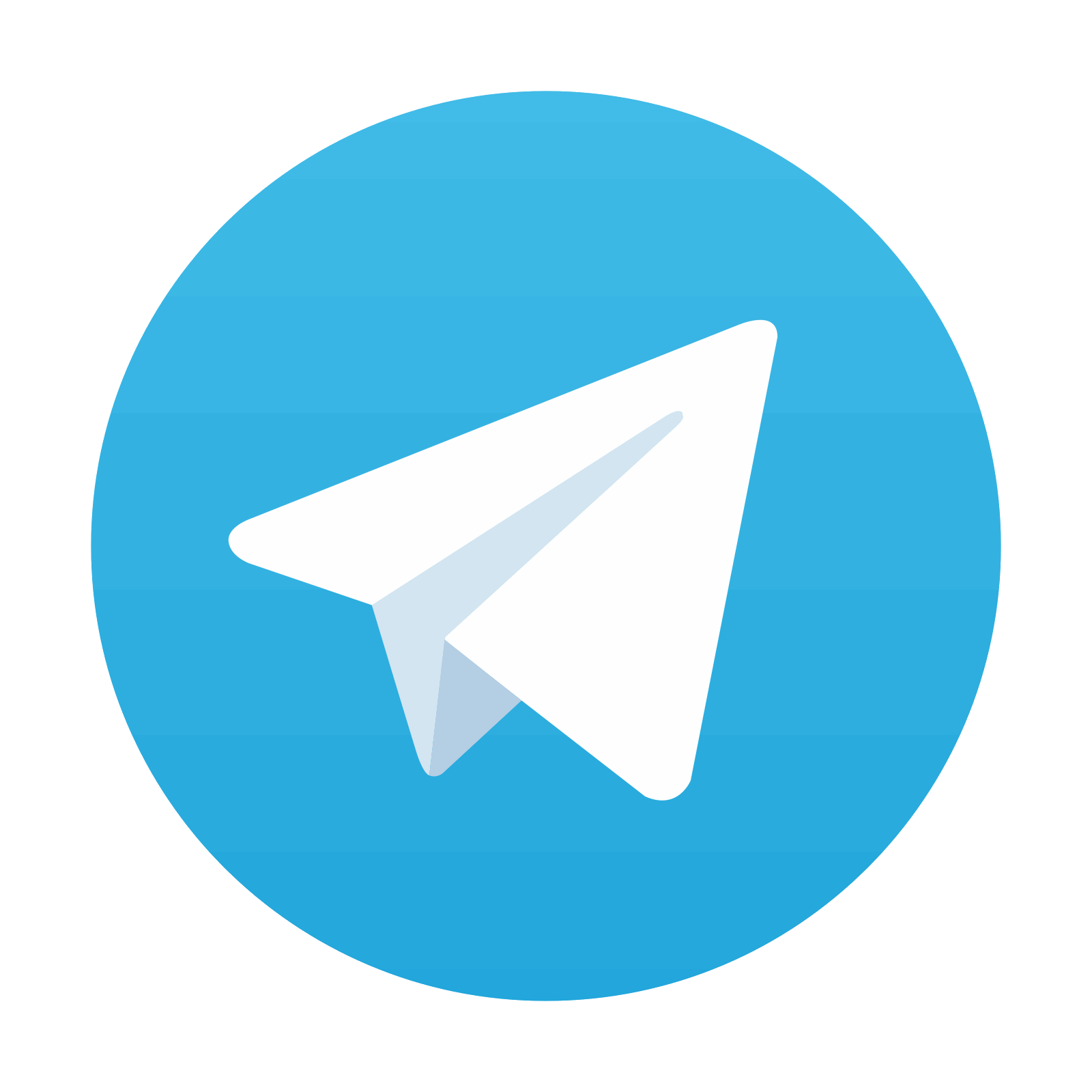
Stay updated, free articles. Join our Telegram channel

Full access? Get Clinical Tree
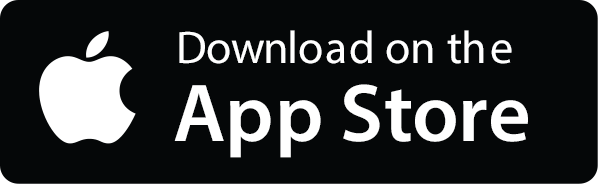
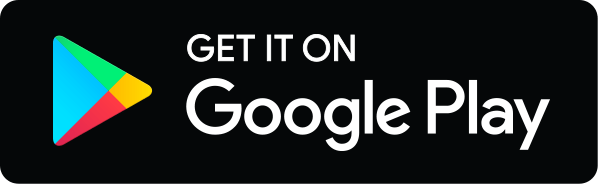