Fig. 3.1
Microvascular proliferation: microvessels in red (factor VIII) with some dividing endothelial cells in blue (Ki67). Tumor cells (to the left) show high degree of proliferation (Ki67-positive nuclei)
In these early studies, there was no information on the importance of vascular proliferation for patient prognosis. In 2006, Stefansson et al. showed for the first time that vascular proliferation (i.e., proliferating microvessel density, pMVD; microvessel proliferation, MVP) was an independent prognostic factor, shown in endometrial cancer, and pMVD was superior to microvessel density by multivariate analysis [23]. The median vascular proliferation index (VPI), i.e., the percentage of microvessels, within hot spot areas, with evidence of proliferating endothelial cells by Ki67 staining, was 3.9%, with a range of 0–21% within the tumor tissue. Microvessel proliferation was found to be increased in cases with the presence of tumor necrosis and with high tumor stage (by FIGO categories). In the same study, vascular proliferation was an independent prognostic factor by multivariate analysis in addition to histologic grade, vascular invasion by tumor cells, and tumor stage.
In studies of breast cancer, using three independent cohorts including 499 patients, Arnes et al. found that median vascular proliferation ranged from 0.95% to 1.95% and was associated with estrogen receptor-negative tumors and reduced patient survival, whereas microvessel density was not significant [48]. It was further shown by Nalwoga et al., in 2 breast cancer cohorts including 431 cases, that vascular proliferation was significantly increased in estrogen receptor-negative cases and in tumors with a basal-like phenotype [49]. The mechanism for such a relationship in breast cancer is not known. It was shown that basal-like and triple-negative cancers were associated with VEGF expression [50], a key regulator of breast cancer angiogenesis [51], and VEGF-driven angiogenesis might contribute to the increased vascular proliferation that we found among basal-like tumors. Increased vascular proliferation in basal-like compared to luminal breast cancer was recently also shown by Kraby et al. [52].
It was reported in 2009 by Gravdal et al. that when combining Ki67 for endothelial proliferation with a marker of immature endothelium, nestin, the prognostic sensitivity was increased [53]. By studying prostate cancer, nestin/Ki67 co-expression, as a marker of vascular proliferation, was four- to fivefold higher in castration-resistant cancers and metastases compared with localized tumors and prostatic hyperplasias. Among localized cancers, high vascular proliferation was a strong and independent predictor of biochemical failure, clinical recurrence, and time to skeletal metastasis by multivariate analysis. In castration-resistant cancers, vascular proliferation was still associated with reduced patient survival. In breast cancer, by nestin/Ki67 co-expression, a median vascular proliferation of 2.7% was found by Krüger et al. [54]. There were significant associations with estrogen receptor-negative tumors as well as basal-like and triple-negative phenotypes. In this study, vascular proliferation was an independent predictor of death from breast cancer.
Interestingly, in a study by Haldorsen et al., microvascular proliferation in endometrial cancers was compared with imaging parameters obtained from preoperative dynamic contrast-enhanced magnetic resonance imaging (DCE-MRI) and diffusion-weighted imaging (DWI) to explore the relationship between these markers and their potential ability to identify patients with poor outcome [55]. Microvascular proliferation was found to be negatively correlated to tumor blood flow by MRI, possibly reflecting an abnormal and reduced functionality in newly formed tumor-associated vasculature. In this study, vascular proliferation was significantly associated with reduced patient survival.
In a study by Stefansson et al. in 2015, a 32-gene expression signature was found to separate tumors with high versus low microvascular proliferation [56]. This 32-gene signature was associated with high-grade tumor features and reduced survival by independent cohorts. Interestingly, copy number studies revealed a strong association between microvessel proliferation and 6p21 amplification. VEGF-A is known to be located in the 6p21 chromosomal region [57], and integrated analyses demonstrated significant associations between increased vascular proliferation and VEGF-A mRNA expression, pointing to a possible angiogenesis driver mechanism in endometrial cancer. In a previous study of endometrial cancer, VEGF-A was significantly associated with vascular proliferation and reduced patient survival [23].
Vascular Maturation
The structural integrity and maturation status of blood vessels, i.e., the degree of coverage by cells like pericytes , have been reported [5, 58], and several factors are known to contribute to pericyte recruitment [59, 60]. Reduced maturation might contribute to the atypical structure of vessels in malignant tumors [25, 61]. Also, tumor-associated pericytes are often abnormal when present [62]. Vascular maturation, as estimated by pericyte coverage, appears to be a dynamic process. In prostate cancer, androgen ablation therapy may induce a downregulation of intra-tumoral VEGF followed by selective regression of immature tumor microvessels by apoptosis of endothelial cells not covered by pericytes [63]. These authors suggested that vessel maturation status of individual tumors might predict the efficiency of anti-VEGF tumor treatment. In 2001, Jain proposed that anti-angiogenic therapy might lead to improved maturation and normalization of the tumor vasculature, thereby increasing the efficacy of combined treatment including chemotherapy or radiation [64, 65]. In a clinical study, injection of anti-VEGF was followed by increased maturation of tumor-associated vessels [66], as has also been shown in experimental studies [67, 68]. It was shown that anti-VEGFR2 treatment creates a “normalization window ” of the vasculature for increased efficiency of additional radiation treatment by upregulation of angiopoietin-1 (Ang-1) and degradation of the basement membrane by MMP activation [69]. In a trial of preoperative bevacizumab followed by a combination of bevacizumab and chemotherapy in HER2-negative breast cancer, Tolaney et al. reported that the tumor response appeared to reflect vascular normalization, primarily in patients with high tumor microvessel density [44].
Data on human tumors are limited with respect to clinical correlates and outcome. In a previous study of lung cancer [70], better outcome was found for tumors with high vascular maturation. The mean vascular maturation index (VMI) was 46%, and high VMI was associated with low microvessel density and absence of nodal metastases. In contrast, a study of breast cancer showed no prognostic impact of VMI [71]. In both studies, the basement membrane antibody LH39 was used as a maturation marker. Eberhard et al. studied vascular maturation in six human tumor types and found a wide range in pericyte coverage index from 13% (glioblastoma) to 67% (breast cancer) [24], although no clinical or prognostic evaluation was presented. The authors concluded that differences between various tissues in vascular proliferation and maturation might be of importance for the suitability of anti-angiogenic treatment. In a study of endometrial cancer, Stefansson et al. showed that median pericyte coverage, as estimated by the α-SMA coverage index (SMAI), was 35%, and low SMAI was significantly associated with increased vascular invasion by tumor cells and impaired patient prognosis [23].
In a study of colorectal cancer, semiquantitative and digital-image-analysis-based scoring identified significant associations between low expression of perivascular PDGFR and shorter overall survival. Importantly, perivascular PDGFR-α and PDGFR-β remained independent factors for survival by multivariate analyses [72].
Glomeruloid Microvascular Proliferation
Although tumor vessels frequently have an abnormal structure, both architectural and cytologic atypia might be difficult to assess, and there is no consensus on how to report vascular morphology in a reproducible and efficient way. Some studies have suggested pattern-based angiogenesis markers, such as glomeruloid microvascular proliferations (GMPs) (Fig. 3.2). GMPs, also called “microvascular nests ” or “glomeruloid bodies,” are focal proliferative buddings of a mixture of vascular cells (primarily multilayered endothelial cells in addition to pericytes and macrophages) that at least superficially resemble renal glomeruli [73–76]. In standard tissue sections, GMPs generally consist of 15–100 cells; one or more vascular lumens are usually present, especially in more mature GMPs, but are not necessary.


Fig. 3.2
Glomeruloid microvascular proliferation (GMP) (red vessels, factor VIII), with a few dividing endothelial cells in blue (Ki67), and marked proliferation in tumor cells (Ki67)
GMPs represent a defining histologic feature of glioblastoma multiforme [73, 74] and have been associated with increasing aggressiveness of brain tumors [77, 78]. GMP-like patterns have also been sporadically reported in other tumors, including gastrointestinal carcinomas, thymomas, and different vascular tumors [75, 79–83]. However, until quite recently, human tumors have not been studied systematically.
In animal studies, Dvorak and coworkers induced the formation of “glomeruloid bodies” from preexisting microvessels in mouse skin, through the injection of an adenoviral vector expressing VEGF-A164, indicating that the formation of GMP might represent a VEGF-A-dependent and dysregulated angiogenic response [84]. The formation of new blood vessels through several steps, each with a distinctive morphology, was described in detail; these include mother vessels (MOV) , glomeruloid microvascular proliferation (GMP), and arteriovenous malformations (AVM) [25, 75, 76, 85]. The GMP phenotype was dependent on the continued presence of VEGF-A164, and as VEGF-A164 expression declined, GMPs underwent apoptosis and progressively devolved into smaller, more normal-appearing microvessels [76]. Thus, the GMP generated in this model required exogenous VEGF-A164 for their maintenance as well as for their generation. This finding is likely relevant to GMP in human tumors. All of the tumor types known to form GMP also express VEGF-A. Another human parallel appears to be the POEMS syndrome , where increased VEGF-A levels are associated with glomeruloid vascular proliferations in the skin, i.e., glomeruloid hemangioma [79].
In a study by Straume et al. in 2002 of more than 700 human cancers (breast, endometrial, prostate, melanoma), approximately 20% of the cases were considered GMP positive (range 13–23%). The presence of GMP was significantly related to poor prognosis [27], and this has been confirmed in studies of non-small cell lung cancer [86] and pancreatic cancer [87]. This angiogenic phenotype was found to be a better predictor of outcome than microvessel density [16].
In the series of nodular melanomas, 23% were GMP positive, and the presence of GMP was significantly associated with aggressive tumor features like increasing lesion thickness (a.m. Breslow) and ulceration. In survival analysis, GMP was an independent prognostic factor along with Clark’s level of tumor invasion and ulceration, and GMP was of greater value in this regard than microvessel density. To extend these studies, the presence of GMP in relation to the expression of several different angiogenic factors and their receptors in melanoma was evaluated [88]. GMP was associated with increased endothelial cell expression of VEGF receptor-1 (FLT-1), VEGF receptor-2 (KDR), and neuropilin-1. The expression of VEGF-A protein in tumor or endothelial cells was not associated with the presence of GMP, whereas VEGF-A expression was significantly stronger in GMP endothelium compared with non-GMP endothelium within the tumors. There was a significant association between the lack of Tie-2 expression in tumor-associated endothelial cells and the presence of GMP, whereas there was no association with the expression of angiopoietin-1 (Ang-1) [88]. Taken together, these findings indicate that increased expression of VEGF receptors on the endothelium in melanomas was associated with presence of GMP, whereas the opposite was found for Tie-2, a receptor that has been linked to vessel maturation [10]. Expression of bFGF was decreased in GMP endothelium, and this has been associated with a less mature vasculature [27].
In the initial study [27], 17% of breast carcinomas were GMP positive, and the presence of GMP was related to the ductal histotype, high grade, estrogen receptor negativity, and HER2 expression. Regarding prognosis, GMP was found to be an independent prognostic indicator by multivariate analysis, providing additional information beyond basic variables such as tumor size, histologic grade, and lymph node metastases. Notably, GMP was not correlated with microvessel density which was not prognostic in this patient cohort. These findings indicate that GMP may provide a novel prognostic marker, indicative of a more aggressive vascular phenotype.
Further studies on breast cancer indicated that GMP is associated with multiple markers of aggressive tumors like estrogen receptor negativity and a basal-like phenotype [89], and the GMP vascular phenotype has been associated with the presence of BRCA1 germline mutations and p53 alterations [90]. BRCA1-related breast cancers have a distinct profile on microarray analysis [91] and also a characteristic spectrum of TP53 mutations [92]. Our data suggest that BRCA1 mutations might induce a genetic profile of which GMP is an important manifestation and part of the tumor phenotype. Of relevance, BRCA1 protein has been associated with inhibition of VEGF transcription and secretion in breast cancer cells these.
We previously found a significant association between GMP and pathologic expression of p53 protein [90], whereas p53 overexpression was not associated with increased microvessel density. The relationship between p53 and angiogenesis could involve several different mechanisms: (1) p53 is known to suppress the expression of VEGF [94] and interacts with the transcription factor Sp1 [95]; (2) p53 degrades hypoxia-inducible factor-1 [96]; (3) p53 downregulates the expression of bFGF-binding protein [97]; and (4) p53 upregulates thrombospondin-1 expression [98].
In a study of locally advanced breast cancer, treated with standard chemotherapy, Akslen et al. found that the presence of GMP, occurring in 21% of the cases, was significantly associated with high-grade tumors and TP53 mutations in addition to the basal-like and HER2-positive subtypes of breast cancer as defined by gene expression data [15]. The GMP phenotype was significantly associated with the lack of treatment response and progressive disease, indicating a potential predictive value. In these tumors, GMP was also correlated to a gene expression signature for tumor hypoxia response, pointing to a possible mechanistic relationship. In a study of metastatic melanoma, GMP in primary tumors (25%) or metastatic tissue (12%) did not predict the response to bevacizumab monotherapy, although limited tissue from metastatic lesions could decrease sensitivity [99].
In endometrial cancer, GMPs were found to be significantly associated with increasing histologic grade, diffusely invasive growth pattern, presence of necrosis, vascular invasion, deep myometrial invasion, and high clinical stage [23]. This study also indicated an association between GMP formation and increased vascular proliferation, by factor VIII/Ki67 co-expression. The findings provide further evidence that GMP is an angiogenic marker of high-grade and aggressive tumors.
In prostate cancer, GMP was present in 13% of cases [27] and was associated with high preoperative levels of serum PSA. The GMP phenotype was an independent predictor of time to biochemical failure as determined by multivariate analysis.
In other tumor types, GMP was a significant prognostic factor in a study of non-small cell lung cancer [86]. A total of 25% of these tumors were GMP positive, and the frequency of GMP was not associated with basic factors such as histologic grade or clinical stage. Similar to our findings [27], there was no association between GMP status and microvessel density in these lung cancers. There was no correlation between VEGF-A expression and the frequency of GMP, although this phenotype was more often seen in Ang-1-positive tumors. Multivariate analysis indicated that GMP was a significant and independent prognostic factor, whereas microvessel density was not. Taken together, these data support the initial observation that GMP might be a novel and significant tissue-based angiogenesis marker for potential clinical use.
Other Vascular Patterns
There has been some additional focus on architectural patterns of angiogenesis in malignant tumors [100]. It seems that qualitative features, rather than quantitative metrics of microvessel density and other markers, may provide some prognostic relevance in certain tumor types, like glioblastomas of the brain, and ocular melanomas. Some studies have focused on the distribution pattern of microvessels within tumors. The EDVIN concept (“edge versus inner”) suggests that comparing vessel counts at the edge of the tumor with the inner area might give a better picture of the angiogenic activity and patient survival. The prognostic value of EDVIN was shown in studies of breast and colorectal cancers [101].
Quantification of vascular pattern by image analysis has shown increased prognostic impact by the use of syntactic structure analysis [102]. Studies of pheochromocytomas, which are highly vascular tumors of the adrenal medulla, have shown that complex and irregular vascular patterns are associated with malignant behavior [103].
Vascular Immunomarkers
Can certain vascular immunomarkers discriminate between endothelial cells in benign tissues and “activated” tumor-associated endothelium? If so, these markers could be applied in tumor imaging and therapeutic targeting, in addition to response prediction and prognostication. This field is very promising but not well developed, and it is not the primary topic of this review. Chi et al. reported expression differences between endothelial cells from various sites of the vascular system [104]. Also, various proteins are differentially expressed in tumor-associated endothelium [105, 106], and such endothelial markers might provide “zip codes” or “maps” for homing of antitumor peptides like LyP1 [107]. St. Croix et al. showed multiple novel antigens being expressed selectively in tumor endothelium from colorectal cancers, some of them associated with the cell membrane (TEM1, TEM7, TEM8) or extracellular matrix [108]. In the same setting, studies from our team indicate that when using the marker nestin for immature endothelium, in addition to Ki67 as a proliferation marker, enhanced and significant prognostic information can be obtained from tissue sections [53, 54].
Pan-endothelial markers , such as von Willebrand factor (factor VIII), CD31, and CD34, are frequently used to visualize endothelial cells by immunohistochemistry when estimating microvessel density. Some reports suggest that CD105/endoglin, a TGF-β receptor involved in vascular development and remodeling, might be suitable as a marker of active angiogenesis in malignant tumors, as well as a therapeutic target on tumor-associated vessels [109]. Microvessel density by CD105 was superior and independent as a prognostic factor in breast cancer [110]. Similar results were presented for lung cancer [111] and prostate cancer [112], whereas no advantage of CD105 was found in studies of endometrial cancer [113] and malignant melanoma [114].
VEGF and its receptors may be present on tumor cells and vessels and might represent targets for imaging and treatment [115]. It was shown that activated microvessel density (aMVD) , as estimated by VEGF/KDR staining on endothelial cells, was highest in the tumor periphery and superior to standard microvessel density (sMVD) as a prognostic factor evaluated by multivariate survival analysis of non-small cell lung cancer [116].
Expression of bFGF on tumor-associated endothelial cells was inversely associated with lymph node metastases and pathological stage of non-small cell lung cancer [117]. Similar findings, together with a prognostic role, have been found for prostate cancer [118] and malignant melanoma [119]. These findings further support the diversity of tumor-associated vessels.
Other angiogenesis markers have been explored, like the expression of tumor-specific endothelial (TEM) antigens [120–122]. Expression of certain integrins, like αvβ3, has been associated with tumor vasculature [123], and this marker might also be applied for imaging [124] and treatment strategies [125]. The main challenge will be to validate such proteins in future studies. Whether simple histology-based tissue markers will prove effective in comparison with other classes of angiogenic markers, like circulating endothelial cells, remains to be studied. Taken together, the study of vascular markers is important for our understanding of tumor-associated angiogenesis, vascular imaging techniques, and the development of therapeutic modalities. Whether gene expression signatures might capture the complexity of malignant tumors and better reflect their angiogenesis capacity remains to be studied in detail.
Markers of Vascular Invasion
One important hallmark of cancer progression is the ability of tumor cells to migrate into vascular channels, i.e., blood vessels or lymphatic vasculature, as an early step of metastatic spread [126]. In breast tumors, vascular invasion is usually considered to be lymphatic vessel involvement (LVI) more often than blood vessel invasion (BVI) [29], but there are few studies in this field. Vascular invasion , as observed on standard tissue sections, is associated with increased risk of tumor recurrence, metastasis, and death from disease [29, 127]. Lymphatic invasion is particularly important as a prognostic factor in early stage breast cancer [128, 129]. Gujam et al. highlighted that immunohistochemistry discriminates better between BVI and LVI, and this distinction improves the prognostic value of vascular invasion compared to standard sections [130].
A potentially different impact of blood vessel invasion as compared with lymphatic involvement has not been well established, for example, in relation to the molecular subtypes of breast cancer. This might be due to the lack of firm criteria to separate blood vessel and lymphatic invasion. Usually, CD31 staining for blood vessel endothelium and D2-40 for lymphatic vessels are applied, although overlapping staining patterns exist. Still, D2-40 expression is considered to be specific for lymphatic endothelium. In a study by Klingen et al., blood vessel invasion, present in 15% of the cases, showed strong associations with non-luminal tumors such as the basal-like, triple-negative, and HER2-positive subgroups [30]. In survival analysis, BVI was significantly associated with recurrence-free and breast cancer-specific survival, whereas LVI was not. When adjusting for basic factors, BVI was an independent prognostic marker, indicating that this feature might be recorded in breast cancer diagnostics, although more studies need to confirm these findings. Development of even more specific markers for blood vessels would be desirable in a routine setting to identify patients at a higher risk for early systemic spread. The potential use of such diagnostic approaches for improved therapy among cases with blood vessel invasion should be considered.
We previously reported that basal-like breast cancers appear to have increased angiogenesis with more microvessel proliferation and higher frequency of the glomeruloid microvascular pattern (GMP) when compared with other breast cancer subtypes [48, 49]. These findings suggest a possible relationship between increased angiogenesis and blood vessel invasion among basal-like breast cancers. The relationships between vascular proliferation, immature vessels, and vascular invasion have also been shown in endometrial cancer [23].
Notably, studies of disseminated tumor cells from the bone marrow, as well as expression profiles of primary tumor cells, suggest that hematogenous spread is often an early event in tumor progression [131]. Early systemic dissemination of breast cancer cells is associated with a specific expression signature, and the molecular pathways associated with primary hematogenous spread and lymphatic dissemination appear to be different [132]. The present data suggest that blood vessel invasion by tumor cells is strongly associated with aggressive tumor subtypes (basal-like, triple negative, HER2 positive). Blood vessel invasion has also been related to interval breast cancer presentation compared with screen-detected tumors [30]. Based on such findings, it might be of practical importance to examine the presence of blood vessel invasion in breast cancers.
It has been suggested that the basal-like phenotype of breast cancer may be related to non-lymphatic spread [133], and findings indicate a reduced risk of axillary lymphatic spread in triple-negative breast cancer [134]. Although the presence of metastases in axillary lymph nodes predicts development of distant metastases, 20–30% of patients with node-negative breast cancer develop metastatic spread at distant sites [135]. As mentioned, early systemic dissemination of breast cancer cells is associated with a specific gene expression signature [132].
In a large study of endometrial cancer, 18% of the tumors showed blood vessel invasion, whereas 31% of the tumors revealed lymphatic involvement [28]. Both BVI and LVI were associated with features such as high histologic grade and diffuse tumor growth. Patients without vascular invasion had the best prognosis and those with BVI (with or without LVI) had the worst outcome, whereas patients with LVI had an intermediate survival by univariate analysis. Both BVI and LVI had independent prognostic importance. Such findings support the biological significance of vascular spread through the hematogenic and lymphatic routes in endometrial cancer. The significant correlation found with clinical phenotype indicates that these markers may be relevant for patient management.
In further studies of endometrial cancer, certain gene expression patterns were associated with vascular invasion by tumor cells as examined on standard sections [136]. Thus, a vascular invasion signature of 18 genes was significantly associated with patient survival and clinicopathologic phenotype. Vascular involvement was related to gene sets for epithelial-mesenchymal transition, wound response, endothelial cells, and vascular endothelial growth factor (VEGF) activity. Further, expression of collagen 8 and MMP3 was associated with vascular invasion, and ANGPTL4 and IL-8 showed a relationship to patient survival. These findings indicate that vascular involvement within primary tumors is associated with gene expression profiles related to angiogenesis and epithelial-mesenchymal transition. This 18-gene expression signature was furthermore studied in multiple cohorts of breast cancer and found to associate with aggressive features like high tumor grade, hormone receptor negativity, HER2 positivity, a basal-like phenotype, reduced patient survival, and response to neoadjuvant chemotherapy [137]. The 18-gene vascular invasion signature was associated with several other gene expression profiles related to vascular biology and tumor progression, including the Oncotype DX breast cancer recurrence signature. Taken together, the findings indicate that markers for vascular invasion by tumor cells in the primary tumor, including gene expression patterns, might provide information that indicate an increased risk of metastatic spread.
Concluding Remarks
It has become increasingly evident that some malignant tumors can be treated by attacking their blood supply. At the same time, both experimental and clinical data have demonstrated that tumor-associated angiogenesis is more complex than reflected simply by the number of microvessels on tissue sections. In the era of targeted therapy, companion biomarkers are becoming crucial to increase treatment efficacy by defining subgroups of patients with high probability of response to the treatment [13, 16], similar to the role of HER2 in breast cancer management. Whereas this is a “hallmark of tailored treatment,” such markers have not yet been successfully established in the field of anti-angiogenesis therapy. In the case of anti-VEGF regimens, there is no simple relationship between the presence of the target (VEGF) and treatment response [13], and no reliable association with the “endpoint” of angiogenic stimulation, i.e., microvessel density, has been found. At the same time, there is a relative lack of translational studies of human tumors, and tissue-based angiogenesis markers should therefore be further studied and validated. Markers reflecting the angiogenic response in primary tumors, such as vascular proliferation and vascular maturation status, need to be examined across different tumor types to increase the evidence of their potential utility, especially as predictive factors. The presence of glomeruloid microvascular proliferation (GMP), reflecting some of the increased irregularity and complexity of tumor-associated angiogenesis and a marker of VEGF-driven angiogenesis, should be considered. Furthermore, a refined immunophenotypic profiling of the tumor vasculature might improve the basis and indications for novel imaging techniques and treatment targets. Complementary systemic biomarkers, such as circulating endothelial progenitor cells, are likely to gain increased importance. Different markers might be combined into profiles to obtain a balance between high-technology methods and simpler cost-effective techniques.
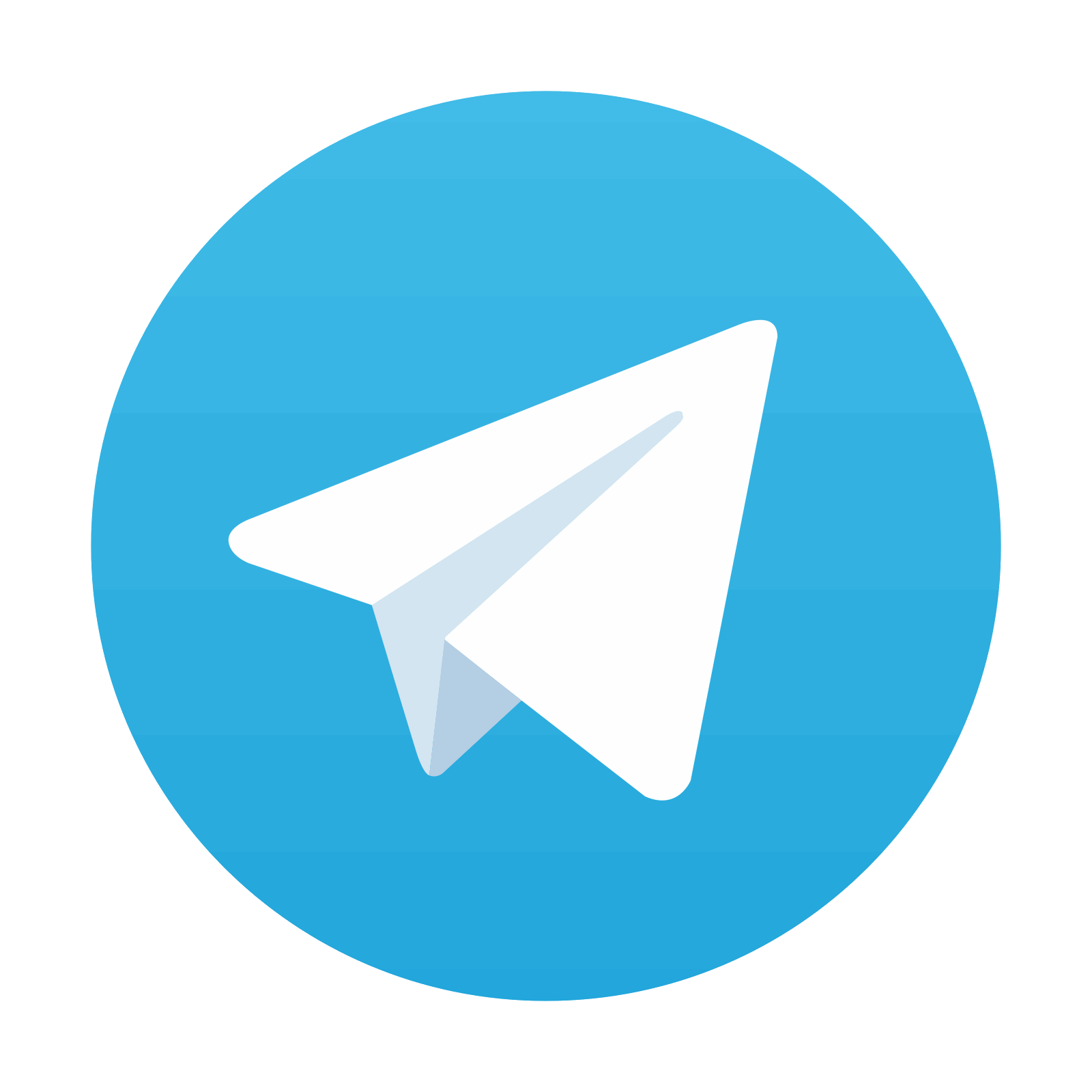
Stay updated, free articles. Join our Telegram channel

Full access? Get Clinical Tree
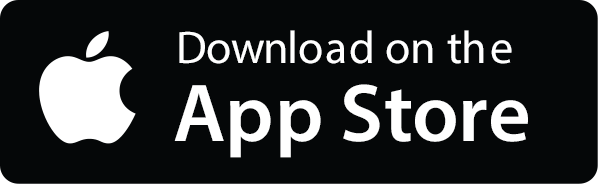
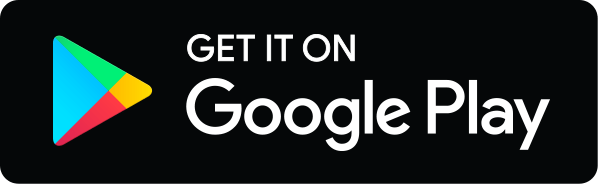