Thyroid Physiology
Peter Kopp
THYROID HORMONE SYNTHESIS AND SECRETION
Thyroid hormones are essential for normal growth and development, particularly of the central nervous system, and they play a key role in regulating metabolism. The synthesis of thyroid hormones requires a normally developed thyroid gland, a functioning hypothalamic-thyroid axis, an adequate nutritional iodine intake, and a series of regulated biochemical steps within thyroid follicular cells. Thyroid hormone action occurs predominantly through regulating two specific nuclear transcription factors, thyroid hormone receptor α and β (TRα and TRβ), which alter the expression of numerous genes throughout the organism. Depending on the gene, this transcriptional regulation can be positive (e.g., myosin heavy chain α) or negative (e.g., TSH [thyroid-stimulating hormone]). In addition, thyroid hormones can also exert nongenomic effects.
The Hypothalamic-Thyroid Axis
The thyroid is controlled by a classic hypothalamic-pituitary axis (Fig. 3.1).1 The hypothalamic tripeptide thyrotropin-releasing hormone (TRH) stimulates the production and secretion of the glycoprotein hormone TSH in the pituitary. TSH is formed of an α and a β subunit; the α subunit is common to the glycoproteins TSH, follicle-stimulating hormone, luteinizing hormone, and human chorionic gonadotropin.
On thyroid follicular cells, TSH binds to the TSH receptor, a G protein-coupled transmembrane receptor, which is expressed at the basolateral membrane (Fig. 3.2).2 TSH binding results in stimulation of cell growth, cell differentiation, and thyroid hormone synthesis. Activation of the TSH receptor leads primarily to coupling with the stimulatory G protein Gsα and subsequent activation of adenylyl cyclase. This, in turn, leads to an increase in intracellular cyclic AMP, phosphorylation of protein kinase A, and activation of numerous cytosolic and nuclear target proteins. At high doses of TSH, the TSH receptor also couples to Gq/11 and thereby activates the phospholipase C-dependent inositol phosphate Ca2+/diacylglycerol pathway. This results in an increase in hydrogen peroxide (H2O2) generation and iodination.
After synthesis of the thyroid hormones, thyroxine (T4) and triiodothyronine (T3) (Fig. 3.3), a process that is discussed in more detail below, they are secreted into the bloodstream. In the serum, most T4 and T3 are protein bound to thyroxine-binding globulin (TBG), transthyretin (TTR), and albumin, and only a minute fraction is present as free hormone.3 Thyroid hormones enter peripheral cells, a process that is at least partially mediated by amino acid channels.4 In the cytosol, T4 is either 5′-deiodinated by deiodinase I or II, resulting in formation of the more active T3, or is inactivated to rT3 by deiodination of the inner ring (Fig. 3.3).5 Thyroid hormone action is primarily mediated by two nuclear receptors, TRα and TRβ, which regulate the transcription of numerous genes.6 In addition, several nongenomic actions are mediated by thyroid hormones. T3 exerts a negative feedback on TRH and TSH secretion (Fig. 3.1).
Thyroid Hormone Synthesis and Its Abnormalities
Thyroid hormone synthesis requires several biochemical steps within thyroid follicular cells and the follicular lumen, the functional unit of the thyroid.7,8,9 Iodide is actively transported into thyroid follicular cells by the sodium-iodide symporter (NIS) at the basolateral membrane (Fig. 3.4).10 This transport requires an electrochemical gradient, which is generated by the Na, K-ATPase. Iodide then reaches the apical membrane. At the apical membrane, iodide is released into the follicular lumen. This efflux occurs, at least in part, through the anion transporter pendrin (PDS/SLC26A4).11 Once iodide reaches the follicular lumen, it is oxidized by the enzyme thyroperoxidase (TPO).7 The oxidation of iodide by TPO requires the presence of H2O2. H2O2 production is catalyzed by the dual oxidase 2 (DUOX2), an enzyme that requires a specific maturation factor, DUOXA2, to reach the apical membrane.12,13 The follicular lumen is filled with colloid, which consists largely of thyroglobulin (TG).12 TG serves as matrix for the synthesis of T4 and T3. In a first step, TPO iodinates selected tyrosyl residues on TG, thereby generating monoiodotyrosine (MIT) and diiodotyrosine (DIT) (organification reaction). In a second step, iodotyrosines are coupled by TPO to form T4 and T3 (coupling reaction). To release thyroid hormones, TG is internalized into the follicular cell by micro- or macropinocytosis and digested in lysosomes. T4 (˜80%) and T3 (˜20%) are subsequently released into the blood stream, in part through the thyroid hormone-transporting channel monocarboxylate transporter 8 (MCT8).13 To recycle iodide, which is a scarce micronutrient in many parts of the world,14,15 MIT and DIT are deiodinated by a recently cloned iodotyrosine dehalogenase, DEHAL1.16 After deiodination, the released iodide is recycled into the follicular lumen and reused for hormone synthesis (Fig. 3.4).
The Sodium-Iodide Symporter
Thyroid follicular cells concentrate iodide up to 40-fold compared with the serum iodide concentrations.17 This active transport is mediated by NIS.10,18 NIS transports two sodium ions and one iodide ion into the cell. This sodium-dependent transport requires a sodium gradient, which is generated by the Na+/K+-ATPase.10,17,19
The human NIS cDNA was cloned shortly after isolation of the rat NIS cDNA.18,20 NIS belongs to the solute carrier family 5 (sodium/glucose cotransport family) and is also designated as SLC5A. The solute carriers of this family are dependent on an electrochemical sodium gradient as the driving force for solute transport. The human NIS gene is located on chromosome 19p13 and consists of 15 exons.21 The encoded protein consists of 643 amino acids, is thought to have 13 transmembrane domains, and undergoes glycosylation.22,23 The amino-terminus is located outside the cell and the carboxy-terminus inside the cytosol.22,23
![]() FIGURE 3.1. The hypothalamic-pituitary-thyroid axis. TRH, thyrotropin-releasing hormone; TSH, thyroid-stimulating hormone; T4, thyroxine; T3, triiodothyronine. |
NIS has a Km for iodide of ˜36 µM.18,24 Electrophysiologic studies revealed that NIS is electrogenic because of the influx of two sodium cations and one iodide anion.25 NIS is blocked competitively by several anions, in particular perchlorate and thiocyanate.26,27 Perchlorate is also actively transported by NIS,28,29 but the transport is electroneutral.25,29 Perchlorate has been used as an antithyroid drug in the treatment of hyperthyroidism in the past but is no longer used for this indication because it can rarely induce aplastic anemia. Although there is concern that contamination of water supplies with perchlorate may result in thyroid dysfunction, this does not seem to be common but may also depend on an adequate iodine intake.30
Perchlorate is also used diagnostically in the perchlorate discharge test. The perchlorate test is performed to determine the extent of iodide organification.27 Under normal conditions, iodide is organified very rapidly on tyrosyl residues of TG after crossing the basolateral and the apical membrane (Fig. 3.4). Any intrathyroidal iodide that has not been incorporated into TG is released into the bloodstream, but as long as NIS is not blocked, it will again be transported into the cell. In contrast, all iodide that is released cannot be transported back into thyroid follicular cells if NIS is inhibited by perchlorate. In the perchlorate test, radioactive iodide is administered to the patient and the counts accumulating in the thyroid are measured at frequent intervals (uptake). Then, 1 g of KClO4 or NaClO4 is administered, and the amount of intrathyroidal radioiodine is continually measured. In individuals with normal iodide organification, there is no decrease in intrathyroidal counts after the administration of perchlorate because the iodide has been organified into TG. In contrast, a loss of ≥ 10% of thyroidal counts indicates an organification defect. In a partial organification defect (PIOD), some of the iodide is organified. A PIOD can be associated with congenital defects with abnormal efflux of iodide into the follicular lumen such as in Pendred syndrome, defective H2O2 generation owing to DUOX and DUOXA2 defects, dysfunction of TPO, or a thyroiditis.31,32,33 In the case of a total organification defect (TIOD), for example, in patients with complete inactivation of TPO, there is no meaningful organification and the radioiodine is completely released from the gland.
TSH stimulates iodide uptake in thyroid follicular cells through various mechanisms. It upregulates NIS mRNA and protein expression in vivo and in vitro22,34,35,36 and prolongs the half-life of
NIS protein.37 TSH also leads to increased insertion and retention of NIS in the plasma membrane.10 Iodide accumulation and organification is also directly regulated by iodide.38,39,40 NIS mRNA expression is decreased after exposure to moderate and high doses of iodide.36,41 In addition, iodide may increase NIS protein turnover and induce a decrease in NIS activity.41,42,43,44 High doses of iodide block thyroid hormone synthesis acutely through inhibition of the organification process (Wolff-Chaikoff effect).38 This inhibition is transient and requires a high intracellular iodide concentration.38,39 Because of the inhibition of NIS expression, the intracellular concentrations gradually decrease and the inhibitory effect dissipates.45
NIS protein.37 TSH also leads to increased insertion and retention of NIS in the plasma membrane.10 Iodide accumulation and organification is also directly regulated by iodide.38,39,40 NIS mRNA expression is decreased after exposure to moderate and high doses of iodide.36,41 In addition, iodide may increase NIS protein turnover and induce a decrease in NIS activity.41,42,43,44 High doses of iodide block thyroid hormone synthesis acutely through inhibition of the organification process (Wolff-Chaikoff effect).38 This inhibition is transient and requires a high intracellular iodide concentration.38,39 Because of the inhibition of NIS expression, the intracellular concentrations gradually decrease and the inhibitory effect dissipates.45
Inactivating homozygous or compound heterozygous mutations of the NIS gene have been identified in individuals with hypothyroidism associated with impaired iodide uptake.10 Many of these patients have a diffuse or nodular goiter, a very low or no uptake of radioiodine, and a decreased saliva/serum radioiodine ratio.
Pendrin as Mediator of Iodide Efflux at the Apical Membrane
At the apical membrane, iodide is transported into the follicular lumen (Fig. 3.4). This is facilitated by an electrochemical gradient because the interior of the follicle is negatively charged. TSH rapidly stimulates iodide efflux into the follicular lumen, although leaving efflux in the basal direction unchanged.46,47,48 On the basis of electrophysiologic studies performed with inverted plasma membrane vesicles, iodide efflux is thought to be mediated by two apical iodide channels with different affinities for the anion (Km ˜70 µM and ˜33 mM).49 The demonstration of iodide transport by the anion channel PDS/SLC26A4, together with the clinical phenotype in patients with Pendred syndrome (see below), suggests that PDS/SLC26A4 could correspond to one of the channels.11,50,51,52,53 Pendred syndrome is an autosomal recessive disorder caused by biallelic mutations in the PDS/SLC26A gene and defined by sensorineural deafness, goiter, and impaired iodide organification.54,55,56
The PDS/SLC26A4 gene encompasses 21 exons and contains an open reading frame of 2,343 base pairs (bp).50 The solute carrier family 26 contains several anion transporters and the motor protein of the outer hair cells, prestin (SLC26A5).57,58,59 The genes encoding PDS/SLC26A4, DRA/SLC26A3 (downregulated in adenoma/congenital chloride diarrhea), and prestin (SCLC26A5) are located in proximity on chromosome 7q21-31 and have a very similar genomic structure suggesting a common ancestral gene. PDS/SLC26A4 is a hydrophobic membrane protein consisting of 780 amino acids with 12 putative transmembrane domains.11,50,60 PDS/SLC26A4 contains a so-called STAS (sulfate transporter and anti-sigma factor antagonist) domain in its intracellular carboxyterminus.61 It has been suggested that the STAS domain of SLC26 members can interact with the regulatory domain of cystic fibrosis transmembrane conductance regulator in some epithelial cells,62,63,64 but a clear functional role for this interaction has not been demonstrated.
PDS/SLC26A4 can mediate chloride and iodide uptake in Xenopus oocytes in a sodium-independent manner.51 In thyroid cells, PDS/SLC26A4 is expressed at the apical membrane,60,65 a finding suggesting that it could have a role in iodide transport into the follicle.57,60 Functional studies in transfected cells confirmed that PDS/SLC26A4 can indeed mediate iodide efflux.52,53 More definitive evidence for PDS/SLC26A4-mediated apical iodide efflux was obtained in a model system with polarized Madin-Darby canine kidney cells expressing NIS and PDS/SLC26A4.11 Although TSH does not regulate the expression of PDS/SLC26A4, it rapidly upregulates membrane insertion, which results in increased efflux of iodide from thyroid cells.66
TG, the Matrix for Thyroid Hormone Synthesis
TG serves as the matrix for the synthesis of thyroxine (T4) and triiodothyronine (T3) and for the storage of thyroid hormone.12
TG is encoded by a very large gene that consists of 48 exons.68,69,70 It is located on chromosome 8q24.2-8q24.3.71,72,73 The TG promoter is regulated by the transcription factors TTF1 (NKX2.1), TTF2 (FOXE2), and PAX8.74,75 The open reading frame consists of 8,307 bp encoding a protein of 2,768 amino acids.76,77 Alternative splicing generates various transcripts, and the TG gene contains numerous single-nucleotide polymorphisms.78
TG is encoded by a very large gene that consists of 48 exons.68,69,70 It is located on chromosome 8q24.2-8q24.3.71,72,73 The TG promoter is regulated by the transcription factors TTF1 (NKX2.1), TTF2 (FOXE2), and PAX8.74,75 The open reading frame consists of 8,307 bp encoding a protein of 2,768 amino acids.76,77 Alternative splicing generates various transcripts, and the TG gene contains numerous single-nucleotide polymorphisms.78
TG is secreted as dimer and is heavily glycosylated.79,80,81 Other secondary modifications of TG include sulfation and phosphorylation.82,83,84 The TG protein has four major regions based on internal homology,85,86,87 and the carboxy-terminal part of the TG monomer shares remarkable homology with acetylcholinesterase.87,88 These structural characteristics suggest that the TG gene emerged from the fusion of two ancestral genes.89
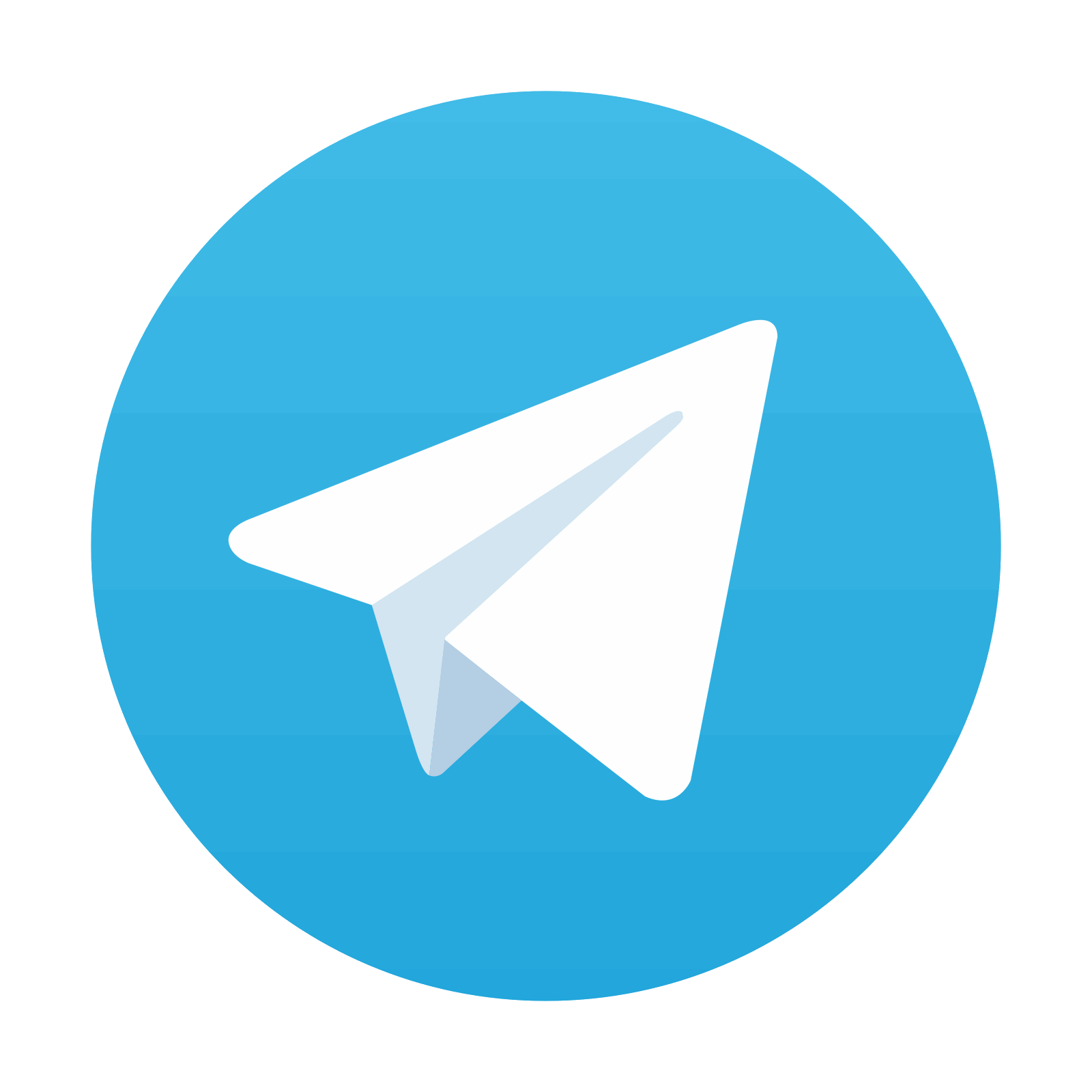
Stay updated, free articles. Join our Telegram channel

Full access? Get Clinical Tree
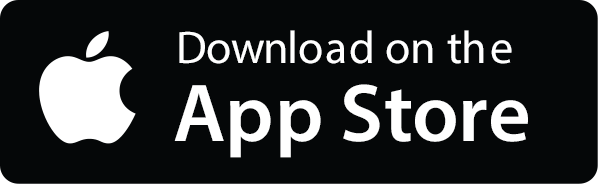
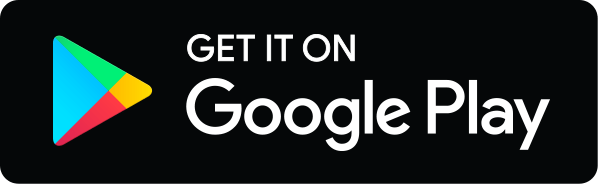
