ANATOMY & PHYSIOLOGY
The physiology of respiration and the anatomy of the chest wall are tightly linked. The chest wall is an airtight, expandable, cone-shaped cage. Normal ventilation occurs when expansion of the rib cage and simultaneous diaphragmatic excursion create negative intrathoracic pressure, allowing inward flow of air.
The function of the chest wall is made possible by its segmentally arranged anatomy. The ventral wall of the bony thorax extends from the suprasternal notch to the xiphoid, approximately 18 cm in the adult. It is formed by the manubrium, sternum, and xiphoid process. The remainder of the anterior wall and the lateral walls are formed by 12 ribs. The first seven pairs of ribs articulate directly with the sternum, the next three pairs connect to the lower border of the preceding rib, and the last two terminate in the wall of the abdomen. The sides of the chest wall consist of the upper 10 ribs, which slope obliquely downward from their posterior attachments. The posterior chest wall is formed by the twelve thoracic vertebrae, their transverse processes, and the 12 ribs (Figure 18–1). The upper ventral portion of the thoracic cage is covered by the clavicle and the subclavian vessels. Laterally, it is covered by the shoulder girdle and axillary nerves and vessels; dorsally, it is covered in part by the scapula.
The superior aperture of the thorax (also called either the thoracic inlet or the thoracic outlet) is a downwardly slanted 5- to 10-cm kidney-shaped opening bounded by the first costal cartilages and ribs laterally, the manubrium anteriorly, and the body of the first thoracic vertebra posteriorly. The inferior aperture of the thorax is bounded by the twelfth vertebra and ribs posteriorly and the cartilages of the seventh to tenth ribs and the xiphisternal joint anteriorly. It is much wider than the superior aperture and is occupied by the diaphragm.
The blood supply and innervation of the chest wall are via the intercostal vessels and nerves (Figures 18–2 and 18–3). The upper thorax also receives vessels and nerves from the cervical and axillary regions. The underside of the sternum’s blood supply derives from the internal thoracic artery branches, which anastomose with the intercostal vessels along the lateral aspect of the chest wall.
The entirety of the thoracic cavity is lined by a pleural membrane. The parietal pleura is the innermost lining of the chest wall and is divided into four parts: the cervical pleura (cupola or cupula), costal pleura, mediastinal pleura, and diaphragmatic pleura. The visceral pleura is a mesodermal layer investing the lungs and is continuous with the parietal pleura, joining it at the hilum of the lung. The potential pleural space is a capillary gap that normally contains only a few drops of serous fluid. However, this space may be enlarged when fluid (hydrothorax), blood (hemothorax), pus (pyothorax or empyema), lymphatic fluid (chylothorax), or air (pneumothorax) fills this potential cavity.
Ventilation is the process of moving gas through the conducting airways, to and from the alveoli, and occurs when elevation of the rib cage and descent of the diaphragm cause an increase in thoracic volume and generate negative intrathoracic pressure. In infants, the ribs have not yet assumed their oblique contour and ventilation depends on diaphragmatic breathing. Furthermore, accessory muscles of respiration contribute to the conformational change in the thoracic cage during periods of intense exercise or respiratory distress (Figure 18–4).
Expiration is mainly passive, resulting from elastic recoil of the lungs. An exception to this is deep breathing, when the abdominal musculature contracts, pulling the rib cage downward and simultaneously elevating the diaphragm by compressing the abdominal viscera against it.
The pleural cavity pressure is normally negative, owing to the opposing forces of elastic recoil of the lung and active expansion of the space by the chest wall. During quiet respiration, it varies from −15 cm H2O with inspiration to 2 cm H2O during expiration. Larger pressure changes (eg, −60 cm H2O during forced inspiration to +30 cm H2O during vigorous expiration) may occur with deep breathing. Because of gravity, pleural pressure at the apex is more negative when the body is upright and changes about 0.2 cm H2O per centimeter of vertical height.
The formation (transudation) and reabsorption of fluid within the pleural space depends on hydrostatic, colloid, and tissue pressures (the Starling equation) in addition to permeability of the pleural membrane. In health, fluid is formed by the parietal pleura and absorbed by the visceral pleura (Figure 18–5). Since systemic capillary hydrostatic pressure is 30 cm H2O, and intrapleural negative pressure averages –5 cm H2O there is a net hydrostatic pressure of 35 cm H2O. Additionally, the colloid osmotic pressure of the systemic capillaries is 34 cm H2O and an opposing 8 cm H2O of pleural space osmotic pressure. Thus, a net 26 cm H2O osmotic pressure draws fluid back into systemic capillaries. Since systemic hydrostatic pressure (35 cm H2O) exceeds osmotic capillary pressure (26 cm H2O) by 9 cm H2O, there is a 9 cm H2O net drive of fluid into the pleural space by systemic capillaries in the chest wall. Similar calculations for the visceral pleura involving the low-pressure pulmonary circulation will show that there is a resulting net drive of 10 cm H2O that attracts pleural fluid into pulmonary capillaries. Thus, there is normally a balance favoring neither loss nor gain of fluid in this space.
In health, pleural fluid is low in protein (<100 mg/dL). When it increases in disease to about 1 g/dL, the net colloid osmotic pressure of the visceral pleural capillaries is equaled and pleural fluid reabsorption becomes dependent on lymphatic drainage. Thus, abnormal amounts of pleural fluid may accumulate: (1) when hydrostatic pressure is increased, such as in heart failure; (2) when capillary permeability is increased, as in inflammatory or neoplastic disease; or (3) when colloid osmotic pressure is decreased.
The mediastinum is the compartment between the pleural cavities. It extends anteriorly from the suprasternal notch to the xiphoid process and posteriorly from the first to the eleventh thoracic vertebrae. Superiorly, fascial planes in the neck are in direct communication; inferiorly, the mediastinum is limited by the diaphragm. Apertures through the inferior extent of the mediastinum are traversed by the aorta, inferior vena cava, esophagus, and vagus nerve.
The mediastinum may be divided into a number of compartments in several ways. Classically, it may be divided into the superior, anterior, middle, and posterior compartments. The superior compartment extends above a line drawn from the fourth thoracic vertebrae to the sternomanubrial junction (Angle of Louis.) In the three-compartment Burkell classification (Figure 18–6), the anterior mediastinum contains the thymus gland, the lymph nodes, the ascending aorta and transverse aorta, the great vessels, and areolar tissue. The middle mediastinum contains the heart, the pericardium, the trachea, the hila of the lungs, the phrenic nerves, lymph nodes, and areolar tissue. The posterior mediastinum contains the sympathetic chains, the vagus nerves, the esophagus, the thoracic duct, lymph nodes, and the descending aorta.
Congenital abnormalities within the mediastinum are numerous. A defect in the anterior mediastinal pleura with communication of the right and left hemithorax is rare. This retrosternal part of the anterior mediastinum is normally thin, and overexpansion of one pleural space may cause “mediastinal herniation” or a bulge of mediastinal pleura toward the opposite side.
Displacements of the mediastinum occur from masses or from accumulations of air, fluid, blood, or chyle interfering with vital functions. Tracheal compression, vena caval obstruction, and esophageal obstructions cause clinical symptoms. The mediastinum can also be displaced laterally when pathologic processes of one hemithorax cause mediastinal shift. Fibrosis and lung volume loss can shift the mediastinum toward the affected side. Open pneumothorax and massive hemothorax shift the mediastinum away from the affected side. Open pneumothorax produces alternating paradoxic mediastinal shifts with respiration and will adversely affect ventilation. Acute mediastinal displacement may produce hypoxia or reduced venous return and cause dysrhythmias, hypotension, or cardiac arrest.
The fundamental unit of lung anatomy is a bronchopulmonary segment (Figure 18–7). The right lung has three lobes: upper, middle, and lower. The left lung consists of two lobes: upper and lower. On the left, the lingular segments of the upper lobe are the homolog of the right middle lobe. Two fissures of varying completeness separate the lobes on the right side. The major, or oblique, fissure divides the upper and middle lobes from the lower lobe. The minor, or horizontal, fissure separates the middle from the upper lobe. On the left side, the single oblique fissure separates the upper and lower lobes. The parenchymal anatomy can be seen by studying the sequential division of the bronchopulmonary tree. The trachea and main stem bronchi and their branches contain a posterior membranous area and are prevented from collapsing by horseshoe-shaped anterior segments of cartilage in their walls. The cartilaginous reinforcement of the airway gradually becomes less complete as the branches become smaller, and reinforcement ceases with bronchi of 1-2 mm. The bronchopulmonary segmental anatomy is designated by numbers (Boyden) or by name (Jackson and Huber). There are typically 18 bronchopulmonary segments (right upper 3, right middle 2, right lower 5, left upper 4, left lower 4) as shown in Figure 18–7. The segmental bronchial anatomy is most constant with the pulmonary vascular structures showing more variability.
The lungs have a dual blood supply: the pulmonary and the bronchial arterial systems. The pulmonary arteries transmit deoxygenated blood from the right ventricle for oxygenation. They closely accompany the bronchi. The bronchial arteries usually arise directly from the aorta or nearby intercostal arteries and are variable in number. They transmit oxygenated blood to the bronchial wall up to the level of the terminal bronchioles. The pulmonary veins travel in the interlobar septa and do not correspond to the distribution of the bronchi or the pulmonary arteries.
The lymphatics travel in intersegmental septa centrally as well as to the parenchymal surface to form subpleural networks. Drainage continues toward the hilum in channels that follow the bronchi and pulmonary arteries. The lymphatics eventually enter lymph nodes in the major fissures of the lungs, the hilum, and the paratracheal regions.
The direction of lymphatic drainage—irrespective of the primary site—is cephalad and ipsilateral, but contralateral flow may occur from any lobe. The usual sequence of lymphatic spread of pulmonary cancer is first to the regional parabronchial nodes and then to the ipsilateral paratracheal, subcarinal, scalene, or inferior deep cervical nodes. The lymphatics from the left lower lobe may be almost equally distributed to the left and right. From the left upper lobe, distribution is often to the anterior mediastinal group (A-P window and para-aortic lymph nodes).
DIAGNOSTIC STUDIES
Skin tests are used in the diagnosis of tuberculosis, histoplasmosis, and coccidioidomycosis. Tuberculin testing is usually done with purified protein derivative (PPD) injected intradermally. Intermediate-strength PPD should be used in patients who seem likely to have active disease. Induration of 10 mm or more at the injection site after 48-72 hours is positive and indicates either active or arrested disease. Because false-negative reactions are rare, a negative test fairly reliably rules out tuberculosis. Mumps antigen is usually placed on the opposite forearm to test for anergy. Skin tests for histoplasmosis and coccidioidomycosis are performed in a similar way, but skin tests for fungal infections are unreliable and serologic tests should be performed instead.
Indirect laryngoscopy is used to assess vocal cord mobility in patients suspected of having lung carcinoma when there has been a voice change. It should also be performed to search for an otherwise occult source for malignant cells in sputum or metastases in cervical lymph nodes.
Roentgenographic evidence of bronchial obstruction, unresolved pneumonia, foreign body, suspected carcinoma, hemoptysis, aspiration pneumonia, and lung abscess are only a few of the indications for bronchoscopy. Depending on the indication, either flexible or rigid bronchoscopy may be performed. Rigid bronchoscopy must be done under general anesthesia and is most often used for clearing major airways of bulky obstructing lesions such as tumors, foreign bodies, or blood clots. Tumor ablation may be done via multiple techniques, including the use of Nd:YAG laser.
Flexible bronchoscopy is a highly effective diagnostic and therapeutic tool. It can be performed under local and intravenous sedation. Washings are usually obtained for bacterial or fungal culture and cytologic examination. Visible lesions are biopsied directly and brush biopsies are obtained from specific bronchopulmonary segments. Occasionally, transcarinal needle biopsy of a subcarinal node is obtained.
Bronchoscopically, 30%-50% of lung tumors are visible. Brushing, random biopsies, and sputum cytology may still yield a positive diagnosis of cancer or tuberculosis in the absence of a visible lesion. The yield is influenced by size, location, and histologic cell type of the lesion.
Additional techniques available during bronchoscopy include the use of endo-bronchial ultrasound (EBUS) and electromagnetic navigational bronchoscopy. EBUS enables visualization of masses and lymph nodes from the central airways, and facilitates fine needle aspiration of these lesions. Navigational bronchoscopy integrates use of a virtual bronchoscopy obtained from cross-sectional imaging (usually CT scan) and a tracking system based on three dimensional feedback from electromagnetic signals to allow access to lesions deep within the lung parenchyma.
Cervical mediastinoscopy remains a mainstay of evaluation of the mediastinum despite advances in imaging. Properly performed mediastinoscopy samples nodes from at least three stations, including ipsilateral and contralateral paratracheal levels 2 and 4 and subcarinal level 7. Cervical mediastinoscopy is performed through a 3- to 4-cm incision one fingerbreadth above the sternal notch. Dissection proceeds beneath the pretracheal fascia, allowing safe access to mediastinal nodes and avoiding major vascular structures. After palpation, the mediastinoscope can be inserted and nodes biopsied under direct vision. Unclear structures care aspirated prior to attempted biopsy.
Enlarged lymph nodes in the aorticopulmonary window are technically inaccessible by means of standard cervical mediastinoscopy. Extended cervical mediastinoscopy provides access to these aorticopulmonary window nodes. It is performed through the same neck incision as standard mediastinoscopy except the dissection is carried laterally beside the left carotid artery toward and then over the aorta into the aorticopulmonary space. Because of the surrounding structures, this procedure carries significant risks and in patients with dilated or calcific aortas or previous cardiac operations, is contraindicated.
In experienced hands, the complications of mediastinoscopy are minimal (< 1%-2%). Major bleeding complications requiring sternotomy or thoracotomy for repair are infrequent (1%-2%). Other possible complications include pneumothorax, recurrent nerve injury, infection, and esophageal injury.
Mediastinoscopy is almost invariably accurate in the diagnosis of sarcoidosis. It is also useful to diagnose tuberculosis, histoplasmosis, Castleman silicosis, metastatic carcinoma, lymphoma, and carcinoma of the esophagus. It should not be used in the investigation of primary mediastinal tumors, which should be approached by an incision permitting definitive excision.
Anterior mediastinotomy (the Chamberlain procedure) is used to sample nodes and biopsy tissue in the anterior mediastinum, most commonly in the aortopulmonary window. A small (3-4 cm) incision is made over the second or third interspace on the appropriate side of the lesion. Alternatively, the procedure can be performed with videoscopic guidance (video-assisted thoracoscopic surgery [VATS]). The mediastinum is approached through the interspace directly or after excising the costochondral cartilage using either the mediastinoscope or an open technique. Careful attention is paid to preserving the mammary vessels encountered in the dissection. The mediastinum is approached extrapleurally unless lesions specifically within the thorax—effusions, tumors invading the hilum or chest wall—need to be investigated. Furthermore, if additional access is required to facilitate the dissection or to treat a complication, the incision can be converted to a larger anterior thoracotomy.
Complications resulting from anterior mediastinotomy include bleeding, recurrent nerve injury, and infection. Major morbidity is less than 1%-2%.
Scalene lymph node biopsy has been largely replaced by mediastinoscopy although remains important particularly for evaluation of suspicious supracervical lymphadenopathy.
VATS plays an important role in the diagnosis and staging of thoracic malignancies as well as in the resection of isolated peripheral pulmonary nodules and bullous lung disease. Furthermore, it has been an advance in lung biopsy and pleurodesis procedures. Although some oncologic concerns persist, thoracoscopic procedures are the standard of care for many resections, although they have not entirely supplanted open resection. As instruments and techniques have evolved, complications from VATS procedures (persistent air leaks, hemorrhage, tumor seeding, etc) have decreased. Overall, major complication rates of 1%-2% are reported. Faster patient recovery, shorter hospital stays, decreased pain are major advantages of VATS, although long-term differences between videoscopic and formal thoracotomy using muscle-sparing incisions are yet to be demonstrated.
Biopsies of the pleura can be performed either using percutaneous needle techniques, VATS, or open surgical approaches. It is indicated when the cause of a pleural effusion cannot be determined by analysis of the fluid or when tuberculosis is suspected. A definitive diagnosis can be obtained in 60%-80% of cases of tuberculosis or cancer. The principal complication is pneumothorax. Five percent to 10% of biopsy specimens are inadequate for diagnosis. Biopsy of the pleura can be performed via videoscopic or open technique with minimal morbidity, providing the pathologist with a specimen superior to that of needle biopsy.
The most common indication for the use of transthoracic needle biopsy of the lung is the evaluation of a solitary pulmonary nodule. It may also be used to confirm the presence of metastatic disease. Lung biopsy may also be indicated in diffuse parenchymal disease and in some patients with localized lesions. Most commonly, lung biopsies are now performed under CT guidance. Complications following percutaneous needle biopsy include pneumothorax (5%-30%), hemothorax, hemoptysis, and air embolism. Pulmonary hypertension or cysts and bullae are contraindications. Several deaths have been reported. There is about a 60% chance of obtaining useful information. Additionally, there is controversy concerning the risks of spreading the tumor by needle biopsy in localized disease.
Thoracoscopy is the standard approach for open lung biopsy in patients who can tolerate single lung ventilation. Techniques for port placement vary, but all allow introduction of a stapler and operating thoracoscope. In addition to allowing smaller incisions, thoracoscopy allows for the visualization of multiple segments and taking multiple biopsies in diffuse diseases. For open biopsies a limited intercostal or anterior parasternal incision is used to remove a 3- to 4-cm wedge of lung tissue in diffuse parenchymal lung disease. The site of incision is selected for accessibility and potential diagnostic value. The incision is generally made at the fifth interspace on the right at the anterior axillary line to allow for access to all three lobes for biopsy. The middle lobe and lingula are selected in specific cases when pathology exists only in these areas, as they generally yield results of the poorest quality. Open lung biopsy is associated with a lower death rate, fewer complications, and greater diagnostic yield than needle biopsy. It is especially useful in critically ill, immunosuppressed patients for differentiation of infectious infiltrative lesions from neoplastic infiltrative lesions. Peripheral lesions are totally excised by wedge or segmental resection, and deeply placed lesions are removed by lobectomy in suitable candidates.
Sputum cytology can be valuable for detecting lung cancer. Specimens are obtained by deep coughing or by abrasion with a brush, or bronchial washings are obtained by either bronchoscopic or percutaneous transtracheal washing techniques. Specimens should be collected in the morning and delivered to the laboratory promptly. Centrifugation or filtration can be used to concentrate the cellular elements.
In primary lung cancer, sputum cytology is positive in 30%-60% of cases. Repeated sputum examination improves the diagnostic return. Examination of the first bronchoscopic washing material yields a diagnosis in 60% of cases. Postbronchoscopy sputum analysis should always be made at 6-12 and 24 hours, as findings may be positive at these times when previous tests were negative. Cytologic analysis using immunohistochemistry to molecular markers (cytokeratins, hnRNP, etc) has improved accuracy and sensitivity and the ability to detect premalignant lesions.
Computed tomography (CT) is a cornerstone of evaluation of chest pathology. CT scanning is critical in the staging of carcinoma, and has value in defining the extent of metastatic disease.
Although the major value of magnetic resonance imaging (MRI) in the thorax has been in cardiovascular imaging, it can also show invasion of lung cancer into the chest wall, vertebrae, and spinal cord as well as mediastinal structures. MRI has a particular niche in the evaluation of superior sulcus (Pancoast) tumors to establish involvement of the brachial plexus, subclavian vessel, or bony chest wall.
Positron emission tomography (PET) is an important tool in staging and workup of the cancer patient. PET scanners are widely available. PET scanning may identify unsuspected regional or distant disease in up to 20%-30% of patients with lung cancer or esophageal cancer compared with conventional imaging methods (CT, bone scan). PET scanning is more accurate than CT scan in detection of cancer spread to mediastinal lymph nodes. Because of the high negative predictive value of PET scanning, a negative PET scan in the mediastinum permits direct progression to thoracotomy. The presence of a positive PET scan in the mediastinum mandates either mediastinoscopy or, more recently, endoscopic evaluation of mediastinal lymph nodes because of false-positive PET scan results.
The combined PET/CT is highly accurate (> 90%), but by itself it has a 10%-20% false-positive rate in the mediastinum. Therefore, interpretations of PET results must be accepted with caution and must be confirmed by surgical staging when inconsistent with the overall clinical picture.
DISEASES OF THE CHEST WALL
A lung hernia results from a defect in the chest wall may be congenital or acquired as the result of trauma or a surgical operation. Most lung hernias are thoracic in location, but cervical (defects of Sibson fascia) or diaphragmatic herniation may occur occasionally. Lung hernias may present as a tender subcutaneous mass that enlarges with cough or Valsalva. Other than this mass, they are usually asymptomatic. Once diagnosed, these hernias should be repaired, with repair of the skeletal defect and prosthetic mesh reinforcement. This may be done open or with VATS techniques.
Infections of the chest wall or pleural spaces may pose complex challenges in medical management. Infections that appear to involve only the skin and soft tissues may actually represent outward extensions of deeper infection of the ribs, cartilage, sternum, or even the pleural space (empyema necessitatis). Inadequate drainage of superficial infection can lead to inward extension into the pleural space, causing empyema.
Subpectoral abscess is caused by suppurative adenitis of the axillary lymph nodes, rib or pleural infection, or posterior extension of a breast abscess. It may also occur as a complication of chest wall surgery (eg, mastectomy, pacemaker placement). Symptoms include erythema, induration of the pectoral region, and obliteration of the normal infraclavicular depression and may progress to systemic sepsis. Shoulder movement is painful. Organisms most commonly involved include hemolytic streptococci and Staphylococcus aureus. Treatment involves incisional drainage along the lateral border of the pectoralis major muscle and administration of systemic antibiotics.
Subscapular abscess may arise from osteomyelitis of the scapula but most commonly follows thoracic operations such as thoracotomy or thoracoplasty. Winging of the scapula or paravertebral induration of the trapezius muscle is usually present. A pleural communication is suggested if a cough impulse is present or if the size of the mass varies with position or direct pressure. The diagnosis is established by needle aspiration. Open drainage is indicated for pyogenic infections not involving the pleural space. Tubercular lesions should be treated by chemotherapy and needle aspiration, if possible.
In the past, osteomyelitis of the ribs was often caused by typhoid fever and tuberculosis. Except in children, hematogenous osteomyelitis is a rare problem today. Thoracotomy incisions may result in osteomyelitis.
Infection of the sternum most commonly follows median sternotomy incisions, particularly in diabetics. It presents as a postoperative wound infection or mediastinitis with drainage, fever, leukocytosis, and instability of the sternal closure. Treatment consists of control of systemic sepsis with appropriate antibiotics, open drainage, resection of the involved sternum, and reconstruction of the defect with pectoralis muscle, serratus muscle, or omental coverage. Occasionally, sternal osteomyelitis will be due to tuberculosis.
Costal cartilage infections are relatively unresponsive to antibiotic therapy. Once devascularized, perichondral tissue necroses and acts as a foreign body to perpetuate the infection and favor sinus tract formation. The most common cause is direct extension of other surgical infections (eg, wound infection, subphrenic abscess). Surgical division of costal cartilages, as in a thoracoabdominal incision, may predispose to cartilage infection postoperatively if local sepsis develops. A wide variety of organisms have been implicated.
Erythema and induration with fluctuance and often spontaneous drainage can occur. The course can be fulminant or may be indolent over months or years, with periodic exacerbations. Associated osteomyelitis of the sternum, ribs, or clavicle may occur.
The treatment of choice includes resection of the involved cartilage and adjacent involved bony structures. Recurrence is due to underestimation of the extent of disease and inadequate resection.
Chest wall reconstruction may be necessary following trauma, surgical resection, or infection resulting in destruction of chest wall structures. Rigid reconstruction of the chest wall is generally recommended for defects greater than 5 cm, although posterior resections covered by the scapula will not necessarily need rigid mesh reconstruction. Advances in the use of musculocutaneous flaps and the supportive use of methyl methacrylate and Marlex mesh to produce solidity below these muscular flaps have facilitated repairs. In massive chest wall defects, vascularization of the area is essential and can be accomplished by use of omental flaps as well as pectoralis, latissimus dorsi, and rectus flaps. Microsurgical techniques for repair of such defects have greatly expanded the ability of plastic surgeons to deal with extensive resectional and infective processes.
Tietze syndrome is a painful, nonsuppurative inflammation of the costochondral cartilages and is of unknown cause. Recent evidence suggests that costochondritis may represent a manifestation of seronegative rheumatic disease. Local swelling and tenderness are the only symptoms; they usually disappear without therapy. The syndrome may recur.
Several reports have suggested the use of bone scintigraphy and chest CT for diagnosis of infected costochondritis. Bone scanning was effective in localizing and identifying inflamed costochondral junctions. Treatment is symptomatic and may include analgesics (NSAIDs) and local or systemic corticosteroids. When symptoms persist longer than 3 weeks and tumefaction suggests neoplasm, excision of the involved cartilage may be indicated and is usually curative.
Mondor disease consists of localized thrombophlebitis of the anterolateral chest wall. It is more prominent in women than in men and occasionally follows mastectomy. There are few symptoms other than the presence of a localized tender, cordlike structure in the subcutaneous tissues of the abdomen, thorax, or axilla. The disease is self-limited and does not pose a risk of thromboembolism. The possibility of an infective origin or stasis of the interrupted venous return due to neoplasm must be ruled out.
Chest wall tumors may be simulated by enlarged costal cartilages, chest wall infection, fractures, rickets, scurvy, hyperparathyroidism, and other conditions. Most commonly, chest wall lesions present as a mass with localized or referred pain; less than 25% are asymptomatic. Approximately 60% of all chest wall masses prove to be malignant. Lesions arise from one of the three components of the chest wall, including soft tissues (eg, muscle, nerve, fascia), bone, and cartilage.
The majority of tumors arise from either bone or cartilage. Rib involvement is more common than sternal presentation. Chest CT offers the most information for diagnosis and staging. Chest wall sarcomas are associated with pulmonary metastasis. Simple chest x-rays may initially identify a mass, especially if it is calcified. Bone scans should be obtained in all cases.
Initial diagnosis is obtained by limited incisional biopsy (transverse) if the mass is large (> 4 cm). Smaller lesions are excised en bloc, ensuring negative margins, with full knowledge that a malignancy is present in many cases. Classic teaching has been to perform en bloc wide local excisions with immediate reconstruction for all lesions at initial presentations. Progress with adjuvant multimodality therapy, however, for tumors such as rhabdomyosarcomas and Ewing sarcoma supports the use of initial limited biopsy for tissue diagnosis to guide treatment planning.
Lipomas are the most common benign tumors of the chest wall. Occasionally, they are very large and lobulated, and they may have dumbbell-shaped extensions that indent the endothoracic fascia beneath the sternum through an intercostal space. They may communicate with a large mediastinal or supraclavicular component.
These may arise from intercostal or superficial nerves. Solitary neurofibromas are most common, followed by neurolemmomas.
Hemangiomas of the thoracic wall are usually painful and occur in children. Tumors may be isolated or may involve other tissues (eg, lung), as in Rendu-Osler-Weber syndrome.
This rare lesion is seen most often in children. It may have poorly defined borders that make complete excision difficult.
Roughly 50% of all chest wall masses are sarcomas, yet overall they represent only a small percentage (5%) of all malignant soft tissue sarcomas. Survival is determined by the histologic grade, the completeness of resection, and the presence and development of metastases (synchronous or metachronous). Low-grade tumors have 5-year and 10-year survivals approaching 90% and 82%, respectively. With high-grade lesions, however, 5-year survival rates are only 30%-50%. The development of metastasis greatly reduces the chances of survival.
Treatment is directed at a complete resection with emphasis on achieving negative margins (1-2 cm). En bloc resection techniques include raising skin flaps and reconstruction with soft tissue flaps, Marlex mesh, and methyl methacrylate to correct chest wall deformity and prevent paradoxic chest movement.
There are many histologic subtypes of soft tissue sarcoma. Typically, low-grade sarcomas include desmoids or liposarcomas with low-grade features. Next most frequently seen are malignant fibrosarcoma, rhabdomyosarcoma, and malignant fibrous histiocytoma, which are usually high-grade lesions.
Individual histologic subtype is not by itself a significant prognostic variable, but histologic grade is significant. Metastases—either synchronous or metachronous—are most commonly to the lungs (75%) and should be resected if negative margins can be achieved and adequate lung function preserved. Therapy for low-grade lesions should consist of a complete resection. Incompletely resected lesions should be treated with external beam radiation therapy. High-grade lesions should be resected and patients enrolled in clinical trials evaluating the efficacy of systemic adjuvant chemotherapy. Postoperative radiotherapy is often helpful in the setting of close margins or tumor spillage.
Fibrosarcoma is the most common primary soft tissue cancer of the chest wall. It occurs most frequently in young adults. Treatment is neoadjuvant chemotherapy followed by resection. A subtype of these tumors includes neurofibrosarcomas, which involve the thoracic wall almost twice as often as other parts of the body. Also referred to as malignant peripheral nerve sheath tumors or malignant schwannomas, they often occur in patients with neurofibromatosis and usually originate from intercostal nerves.
Malignant fibrous histiocytoma has a bimodal distribution, with peaks between 20 and 30 years of age and between 50 and 60. Although they are the most common soft tissue sarcoma of adults, they rarely rise from the chest wall. Treatment is neoadjuvant chemotherapy followed by resection, followed by additional adjuvant chemotherapy.
Rhabdomyosarcoma is an uncommon tumor in adults but is the second most common chest wall tumor in children. Treatment is neoadjuvant chemoradiation, surgical resection, then ongoing chemotherapy and radiation. These tumors are aggressive and often unresectable.
These tumors account for approximately one-third of all primary cancers of the chest wall. They occur more often in men.
The combined frequency of these three cartilaginous tumors is about 30%-45% of all benign skeletal tumors. Cartilaginous tumors are usually single and occur with equal frequency in males and females between childhood and the fourth decade. The tumors are usually painless and tend to occur anteriorly along the costal margin or in the parasternal area. Wide local excision is curative.
Fibrous dysplasia (bone cyst, osteofibroma, fibrous osteoma, and fibrosis ossificans) accounts for a third or more of benign skeletal tumors of the chest wall. This cystic bone tumor can occur in any portion of the skeletal system, but approximately half involve the ribs. The differential diagnosis includes cystic bone lesions associated with hyperparathyroidism. The tumor is usually single and may be related to previous trauma. Some patients experience swelling, tenderness, or vague discomfort, but the lesion is usually silent and is detected on routine chest x-ray. Treatment consists of local excision.
Eosinophilic granuloma may occur in the clavicle, the scapula, or (rarely) the sternum. Coexisting infiltrates of the lung are often present. This condition often represents a more benign form of Langerhans cell histiocytosis or Hand–Schüller–Christian disease. Fever, malaise, leukocytosis, eosinophilia, or bone pain may be present. Rib involvement presents as a swelling with cortical bone destruction and periosteal new growth. The clinical picture can resemble osteomyelitis or Ewing sarcoma. When the disease is localized, excision will result in cure.
Cavernous hemangioma of the ribs presents as a painful mass in infancy or childhood. The tumor appears on chest x-ray either as multiple radiolucent areas or as a single trabeculated cyst.
Fibromas, lipomas, osteomas, and aneurysmal bone cysts are all relatively rare lesions of the chest wall. The diagnosis is established after excisional biopsy.
Chondrosarcomas are the most common primary malignant tumor of the chest wall (20%-40%). They most commonly develop from the costochondral junctions of the first four ribs but can involve the sternum. About 15%-20% of all skeletal chondrosarcomas occur in the ribs or sternum. Most appear in patients 20-40 years of age. Local involvement of pleura, adjacent ribs, muscle, diaphragm, or other soft tissue may develop. Pain is uncommon and most patients complain only of the mass. Chest x-ray shows destroyed cortical bone, usually with diffuse mottled calcification, and the border of the tumor is indistinct. Successful treatment necessitates wide local excision and en bloc resection to achieve negative margins. Incomplete excision carries a significantly worse prognosis. Overall survival, as in all soft tissue sarcomas, is heavily dependent on the histologic grade. Completely resected low-grade chondrosarcoma has a 60%-80% 5-year survival rate. Patients with high-grade lesions who subsequently develop distant metastasis have only 20%-30% 5-year survival.
Although a complete resection can often be curative, local recurrence portends future metastatic disease and poor survival. Therefore, even in the setting of large tumors (> 15–20 cm), resection should be considered even if it necessitates removal of more than eight ribs. With epidural pain control and immediate reconstruction techniques, most patients will do well. Despite large chest wall resections, most patients can be immediately extubated and will not suffer drastic changes in pulmonary function or chest wall dynamics.
Ewing sarcoma is a small, round cell-type tumor which most commonly occurs in a single rib. It accounts for 10%-15% of all primary chest wall tumors. Presentation as a primary chest lesion is not common (< 15%). Typically, Ewing sarcoma presents as a large, warm, painful soft tissue mass usually associated with pleural effusion. Systemic symptoms such as fever, malaise, and weight loss are common. Radiologic studies demonstrate the classic “onion skin” appearance caused by widening and sclerosis of the cortex as multiple layers of new bone are produced.
Diagnosis can usually be made by fine-needle aspirate or incisional biopsy. Histologically, these tumors are unique and consist of broad sheets of small polyhedral cells with pale cytoplasm and small hyperchromatic nuclei. They stain periodic acid-Schiff-positive.
Ewing sarcoma is commonly a disease of childhood and adolescence. The most important prognostic indicator for survival is development of distant metastases. Current therapy consists of neoadjuvant chemotherapy (including cyclophosphamide, dactinomycin, doxorubicin, and vincristine) surgical resection if the tumor is well demarcated and can be completely resected. Radiotherapy may be indicated as postoperative adjuvant treatment, or in the case of tumors that are not completely resectable. Overall, 5-year survivals range from 15% to 60%. Long-term survivals (10 years) are achievable in patients who do not develop metastases.
Osteosarcoma occurs in the second and third decades, and 60% of cases occur in men. It is more aggressive than chondrosarcoma. X-ray findings consist of bone destruction and recalcification at right angles to the bony cortex, which gives the characteristic “sunburst” appearance. Osteogenic sarcoma most commonly presents as an extremity lesion, with only a small percentage of cases being chest wall primaries. Overall, less than 5% of all osteogenic sarcomas arise in the chest wall. Osteogenic sarcoma occurs in the second to fourth decades of life, half-again more commonly in men than in women. It is associated with environmental triggers, as well as with mutations in the retinoblastoma gene (RB1, 500-1000x risk) and Li-Fraumeni syndrome (p53, 15x risk). Typically, they are more aggressive tumors with a propensity for early metastasis to lung and bone.
Osteogenic sarcoma differs from chondrosarcoma in that it is usually sensitive to chemotherapy. They are treated with neoadjuvant chemotherapy followed by surgical resection. Even with neoadjuvant treatment, however overall 5-year survival after complete resection and postoperative chemotherapy may only be 15%.
Solitary plasmacytomas of the chest wall are comparatively rare lesions. They constitute 5%-20% of all chest wall tumors. Radiologically, they present as classic “punched-out” lytic lesions without evidence of new bone formation. They are more common in men than in women and typically present in the fifth to seventh decades of life. Over 75% of the time, solitary chest wall plasmacytomas are associated with diffuse multiple myeloma. Survival is based on the development of systemic disease. Local control and relief of pain are achieved with radiation therapy (usually 3000-4600 cGy). Once systemic disease is diagnosed, treatment consists of chemotherapy. Overall, 5-year and 10-year survivals for solitary plasmacytomas of the chest wall are 35%-40% and 15%-20%, respectively. Typical median survivals after treatment with radiation therapy and chemotherapy average 56 months.
Metastases to bones of the thorax are often multiple and are usually from tumors of the kidney, thyroid, lung, breast, prostate, stomach, uterus, or colon. Renal cell and thyroid malignancies have a high propensity for metastasizing to the sternum. Occasionally, they present as a pulsatile mass due to the excessive vascularity of the metastasis. An aneurysm of the ascending thoracic aorta, while rare, must be considered in the differential diagnosis and ruled out prior to attempts at excisional biopsy. Involvement by direct extension occurs in carcinoma of the breast and lung. Primary lung cancer with direct extension to chest wall without nodal involvement (T3 N0) carries a reasonable 5-year survival (40%-50%) when treated with radical en bloc resection. Lung metastasis with direct chest wall extension should be treated with radical en bloc resection of the chest wall and underlying lung.
DISEASES OF THE PLEURA
Diseases of the pleura may be benign or malignant and may represent primary pleural processes, localized extrapleural diseases, or systemic illnesses. The most common pleural problem is the presence of air (pneumothorax) within the pleural space. Pleural effusions—accumulations of fluid—result from benign sterile fluid, malignant fluid, pus, chyle, or blood. Primary pleural tumors are uncommon, but involvement of the pleura with metastatic cancer is common.
The most common symptoms of pleural disease are pain and dyspnea. The pain is sharp, and it is characteristically worsened by respiratory movements, often inhibiting inspiration. Pleural pain is mediated through somatic intercostal nerves of the chest wall (cervical and costal pleura) and through the phrenic nerve (diaphragmatic and mediastinal pleura), causing chest wall or back pain and pain referred to the shoulder, respectively. The visceral pleura contains only sympathetic and parasympathetic nerve fibers and therefore is insensate; however, extension of visceral processes to involve the parietal pleura can produce typical pleuritic chest pain.
Pleural effusion is the presence of fluid within the pleural space. More specific terminology may be used when the nature of the fluid is known. Hydrothorax is a collection of serous (most often transudative but also exudative) fluid, while pus in the pleural cavity is referred to as a pyothorax or empyema. Additional terms are used for blood (hemothorax) and chyle (chylothorax). Abnormal pleural fluid accumulates as a result of one or more of the following mechanisms: (1) increase in the pulmonary vascular hydrostatic pressure (congestive heart failure, mitral stenosis); (2) decrease in the vascular colloid oncotic pressure (hypoproteinemia); (3) increase in the capillary permeability due to inflammation (eg, pneumonia, pancreatitis, sepsis); (4) decrease in the intrapleural pressure (atelectasis); (5) decrease in the lymphatic drainage (carcinomatosis); (6) transdiaphragmatic movement of abdominal fluid through lymphatics or physical defects (ascites, pancreatic pseudocyst rupture); and (7) rupture of a vascular or lymphatic structure (traumatic injury).
Decreased respiratory excursion, diminished breath sounds, dullness to percussion, a pleural friction rub, and local tenderness are signs that indicate the presence of pleural effusion. With long-standing and advanced disease, contraction of the hemithorax with narrowed intercostal spaces and localized bulging, swelling, or redness may occur. Chest radiographs demonstrate varying degrees of opacification of the ipsilateral hemithorax. Accumulation of 300-500 mL fluid causes blunting of the costophrenic angle on x-ray. If the entire hemithorax is opacified, 2000-2500 mL may be present. The mediastinum may be shifted to the contralateral side in the presence of a large effusion, or it may remain in the midline—particularly if proximal bronchial obstruction results in lobar or total lung atelectasis, if the mediastinum is fixed from fibrosis or tumor infiltration, if the ipsilateral lung is infiltrated with tumor, or if malignant mesothelioma is present. CT scanning may be required to evaluate complex, loculated, or recurrent pleural fluid collections. Interventional radiology services are useful for loculated pleural effusions that may be managed by percutaneous drain placement under CT guidance.
Generally, serous effusions are separated into two broad categories—transudates and exudates—based on the physical and cellular characteristics of the pleural fluid. Identification of the specific type of effusion aids in determination of the cause and most often depends on examination of at least 20 mL of fluid obtained by thoracentesis. Basic tests should include total protein, lactate dehydrogenase (LDH), total and differential cell counts, glucose, pH, cytology, and Gram stain with culture. Furthermore, simultaneous serum total protein, LDH, and glucose should be measured. Effusions with total protein content less than 3 g/dL (or a fluid-serum ratio lower than 0.5), an LDH level less than 200 units/dL (or a fluid-serum ratio < 0.6), and a specific gravity below 1.016 represent transudates, while all other effusions are classified as exudates. The results of these basic tests frequently allow the underlying pathologic process to be elucidated (Table 18–1).
Tuberculosis | Cancer | Congestive Heart Failure | Pneumonia and Other Nontuberculous Infections | Rheumatoid Arthritis and Collagen Disease | Pulmonary Embolism | |
---|---|---|---|---|---|---|
Clinical context | Younger patient with history of exposure to tuberculosis. | Older patient in poor general health. | Presence of congestive heart failure. | Presence of respiratory infection. | History of joint involvement; subcutaneous nodules. | Postoperative immobilized, or venous disease. |
Gross appearance | Usually serous; often sanguineous. | Often sanguineous. | Serous. | Serous. | Turbid or yellow-green. | Often sanguineous. |
Microscopic examination | May be positive for acid-fast bacilli; cholesterol crystals. | Cytology positive in 50%. | — | May be positive for bacilli. | — | — |
Cell count | Few have > 10,000 erythrocytes; most have > 1000 leukocytes, mostly lymphocytes. | Two-thirds bloody; 40% >1000 leukocytes, mostly lymphocytes. | Few have > 10,000 erythrocytes or > 1000 leukocytes. | Polymorphonu-clears predominate. | Lymphocytes predominate. | Erythrocytes predominate. |
Culture | May have positive pleural effusion; few have positive sputum or gastric washings. | — | — | May be positive. | — | — |
Specific gravity | Most > 1.016. | Most > 1.016. | Most > 1.016. | > 1.016. | > 1.016. | > 1.016. |
Protein | 90% 3 g/dL or more. | 90% 3 g/dL or more. | 75% > 3 g/dL. | 3 g/dL or more. | 3 g/dL or more. | 3 g/dL or more. |
Sugar | 60% < 60 mg/dL. | Rarely < 60 mg/dL. | — | Occasionally < 60 mg/dL. | 5–17 g/dL (rheumatoid arthritis). | — |
Other | No mesothelial cells on cytology. Tuberculin test usually positive. Pleural biopsy positive. | If hemorrhagic fluid, 65% will be due to tumor; tends to recur after removal. | Right-sided in 55%–70%. | Associated with infiltrate on x-ray. | Rapid clotting time; LE cell or rheumatoid factor may be present. | Source of emboli may be noted. |
Other exudates: spgr > 1.016 Fungal infection: Exposure in endemic area. Source fluid. Microscopy and culture may be positive for fungi. Protein 3 g/dL or more. Skin and serologic tests may be helpful. Trauma: Serosanguineous fluid. Protein 3 g/dL or more. Chylothorax: History of injury or cancer. Chylous fluid with no protein but with fat droplets. |
More than 25% of all pleural effusions are secondary to cancer, and 35% of patients with lung cancer, 23% of patients with breast cancer, and 10% of patients with lymphoma develop malignant pleural effusions during the course of their disease. Approximately 10% of malignant effusions are secondary to primary pleural tumors, mostly mesothelioma. The mechanism is primarily through lymphatic obstruction in either the peripheral lung or central lymph node channels of the mediastinum. Malignant pleural effusions can be serous, serosanguineous, or bloody and are diagnosed primarily by demonstrating malignant cells in the fluid. Cytologic confirmation is successful 50%, 65%, and 70% of the time after one, two, or three thoracenteses, respectively. Closed pleural biopsy alone is successful in only 50% of cases, but coupled with thoracentesis it can increase the diagnostic yield to 80%. Thoracoscopy with direct pleural biopsy, however, is successful in 97% of patients and should be considered in any patient with a suspicious effusion after two negative thoracenteses.
Treatment of malignant effusions is strictly palliative: Most patients die within 3-6 months of developing a malignant pleural effusion, so prompt diagnosis and therapy are essential. The goals of treatment are lung reexpansion and pleural symphysis. This is most readily accomplished with placement of a chest tube (2028F) and closed-tube drainage for 24-48 hours. Generally, no more than 1 L is allowed to drain initially. Subsequently, 200-500 mL is allowed to drain every 1-2 hours until the effusion is fully drained. This controlled draining avoids the rare complication of reexpansion pulmonary edema. Once full lung expansion is obtained pleurodesis should be performed with an appropriate agent before loculations have formed. Different chemical, radioactive, and infectious agents have been used in the past with varying success rates, but most commonly used are talc (87%-100% insufflation; 83%-100% slurry), or doxycycline. Finally, mechanical pleurectomy without chemical instillation can control pleural effusions in over 99% of patients, but this requires an operative procedure. Talc is inexpensive, highly effective, and easily administered either as a powder insufflated into the open chest or as a slurry instilled through a chest tube.
Complications following pleurodesis include pneumothorax, loculated hydrothorax, fever, infection (empyema), acute respiratory distress syndrome (particularly following bilateral simultaneous pleurodesis, which for this reason alone are contraindicated), and recurrence. Problems are uncommon, and most patients can have their chest tubes removed within 48-72 hours following talc pleurodesis.
Pleural effusions are common findings in patients with moderate to severe congestive heart failure. Heart failure may be secondary to ischemia, valvular heart disease, viral myocarditis, congenital heart disease, and other less common lesions. The effusion may be bilateral or unilateral. When unilateral, the right hemithorax is most often affected. Fluid frequently involves the interlobar fissures (most commonly the minor fissure on the right) and can form localized collections simulating mass lesions known as “pseudotumors.” Other cardiovascular causes of pleural effusions include constrictive pericarditis and pulmonary venous obstruction.
Hydronephrosis, nephrotic syndrome, and acute glomerulonephritis are on occasion associated with pleural effusions. Rupture of the collecting system into the pleural space can also produce a hydrothorax. In this latter case, the pleural fluid creatinine will be elevated (fluid-serum creatinine ratio significantly > 1.0).
Moderate to severe pancreatitis is associated with a pleural effusion that characteristically occurs on the left and contains fluid with an amylase concentration substantially higher than that in the serum. Rarely pseudocysts of the capsule of the pancreas may communicate with the pleural space, resulting in high-volume pleural effusions.
Approximately 5% of patients with cirrhosis and ascites will develop a pleural effusion. In contrast to pancreatitis, nearly all of these effusions occur on the right side.
Pulmonary thromboemboli are sometimes accompanied by a pleural effusion. These effusions are typically serosanguineous and small, but they may be frankly bloody and massive. Characteristic x-ray findings are almost always present in the lung. Since the fluid is usually reabsorbed in a short period of time, drainage is seldom necessary.
Pyothorax (empyema thoracis) is the accumulation of pus within the pleural cavity. The pus is usually thick, creamy, and malodorous. If empyema occurs in the setting of underlying suppurative lung disease (ie, pneumonia, lung abscess, or bronchiectasis), it is referred to as a parapneumonic empyema (60% of cases). Other causes of thoracic empyema are surgery (20%), trauma (10%), esophageal rupture, other chest wall or mediastinal infections, bronchopleural fistula, extension of a subphrenic or hepatic abscess, instrumentation of the pleural space (thoracentesis, chest tube placement, etc), and, rarely, hematogenous seeding from a distant site of infection.
Empyemas are divided into three phases based on their natural history: acute exudative, fibrinopurulent, and chronic organizing. The acute exudative phase is characterized by the outpouring of sterile pleural fluid (incited by pleural inflammation), with a low viscosity, white blood cell count, and LDH concentration as well as normal glucose level and normal pH. The pleura remains mobile during this phase. The fibrinopurulent phase develops at approximately 2-7 days, marked by an increase in the turbidity, white blood cell count, and LDH levels in the fluid. Glucose levels and pH of the fluid decrease and fibrin is deposited on pleural surfaces, limiting the empyema but also fixing (trapping) the lung. The chronic organizing phase begins 7-28 days after the onset of the disease and is characterized by a pleural fluid glucose level less than 40 mg/dL and a pH less than 7.0. The pleural exudate becomes thick, and the pleural fibrin deposits thicken and begin to organize, further immobilizing the lung. In patients with inadequately treated chronic empyema, erosion through the chest wall (empyema necessitatis), chondritis, osteomyelitis of the ribs or vertebral bodies, pericarditis, and mediastinal abscesses may occur.
The bacteriology of thoracic empyema has evolved over the years. Prior to the discovery of penicillin in the 1940s, most empyemas were caused by pneumococci and streptococci. With modern antibiotics and improved anaerobic culture techniques, however, the most common isolates from adult empyemas are now anaerobic bacteria, particularly bacteroides species as well as fusobacterium and Peptococcus species.
Staphylococcus is the most common organism causing empyema (92% in children under 2 years), and staphylococcal empyema is one of the most common complications of staphylococcal pneumonias in both adults and children (Table 18–2). Gram-negative bacteria also continue to be significant pathogens, particularly in parapneumonic empyemas. Escherichia coli and pseudomonas species account for 66% of aerobic gram-negative empyemas, and other organisms include Klebsiella pneumoniae, Proteus species, Enterobacter aerogenes, and Salmonella. Rarely, fungi (Aspergillus, Coccidioides immitis, Blastomyces, and Histoplasma capsulatum) and parasites such as Entamoeba histolytica can cause empyemas. In a review, empyemas were found to contain anaerobic bacteria in only 35% of cases, aerobic bacteria in only 24%, and a combination in 41%. In addition, the average number of bacterial species isolated was 3.2 per patient.
Aspiration of oropharyngeal flora may represent a source of polymicrobial infection. Although patients may rarely be completely asymptomatic, most patients with thoracic empyemas present with varying symptoms depending on the underlying disease process, the extent of the pleural involvement, and the immunologic state of the patient. Patients typically complain of fever, pleuritic chest pain or a sense of chest heaviness, dyspnea, hemoptysis, and a cough usually productive of purulent sputum. Signs of thoracic empyema include anemia, tachycardia, tachypnea, diminished breath sounds with dullness to percussion on the involved side, clubbing of fingertips, and occasionally pulmonary osteoarthropathy.
Although the medical history and physical examination often suggest the presence of thoracic empyema, the plain chest radiograph is the most important noninvasive diagnostic test. Empyemas may be associated with an underlying pneumonia, lung abscess, or pleural effusion and appear as posterolateral D-shaped densities on x-ray. In large empyemas, the mediastinum may be shifted away from the affected side. Bronchoscopy should be performed on all patients to exclude the presence of endobronchial obstruction. CT scanning provides critical anatomic detail regarding loculations and can assist in differentiation of empyema from lung abscess.
Thoracentesis is the procedure of choice for the diagnosis of thoracic empyema. Aspiration of pus establishes the diagnosis, permitting identification of the offending organisms. In early empyemas—particularly those partially treated with antibiotics—the pleural fluid may not be frankly purulent. In these cases, a pleural fluid pH less than 7.0, glucose less than 40 mg/dL, and an LDH level greater than 1000 units/L strongly suggest an evolving empyema even if Gram stain and cultures fail to identify organisms.
Goals for the treatment of thoracic empyemas include: (1) control of the infection; (2) removal of the purulent material with obliteration and sterilization of the pleural space and reexpansion of the lung; and (3) elimination of the underlying disease process.
Options for treatment include repeated thoracentesis, closed tube thoracostomy, rib resection and open drainage, decortication and empyemectomy, thoracoplasty, and muscle flap closure. Adjunctive maneuvers reported to aid in the disruption and drainage of loculated empyemas include instillation of fibrinolytic enzymes (such as tPA) and video-assisted thoracoscopic debridement. A rational approach to empyema management is outlined in Figure 18–8. Initially, an intercostal catheter of adequate size is carefully inserted into the most dependent portion of the empyema cavity. If after 24-72 hours sepsis persists—or if there is any question as to the adequacy of drainage—a CT scan should be obtained. If, on the other hand, complete drainage and reexpansion of the lung are achieved, no further drainage procedures are necessary.
Patients with residual spaces that are inadequately drained, patients with continued sepsis, and patients thought to require prolonged tube drainage are candidates for open drainage procedures. These can usually be safely performed 10-14 days after closed-tube drainage, since the pleurae fuse by that time and the risk of pneumothorax and lung collapse is eliminated. Options for an open drainage include simple rib resection and open flap drainage (Eloesser procedure). Simple rib resection involves the removal of short segments (3-6 cm) of one, two, or three ribs at the most dependent portion of the empyema cavity (at or anterior to the posterior axillary line). A tube can be placed through this opening and effective drainage established. A second approach involves the creation of a U-shaped flap of chest wall that is sewn to the parietal pleura after resection of short segments (3-6 cm) of one, two, or three ribs. This creates an epithelialized tract for long-term tubeless drainage of empyema cavities. This type of an open drainage allows the empyema cavity to drain reliably and to be easily debrided, irrigated, and cleaned. Ultimately, through lung reexpansion, wound contraction, and granulation, the cavity often completely disappears.
Another option is early decortication and empyemectomy. This is especially useful for good-risk patients with early loculated empyemas and inadequate tube drainage or lung expansion. Furthermore, if performed early in the course of the process, resection of both parietal and visceral pleural peels (decortication) can be performed as a thoracoscopic procedure. More advanced or chronic disease involves a thoracotomy with decortication with resection of the intact empyema itself (empyemectomy), if possible. The best results with this approach are obtained when the underlying lung is entirely normal and reexpands fully. Posttraumatic empyema, in particular, has been amenable to this treatment.
Empyemas that occur following pulmonary resection may be difficult to manage. If residual lung is present (resections less than pneumonectomy), the general principles outlined above still apply, although a complicating bronchopleural fistula is often present (Figure 18–9). Simple tube drainage is instituted initially followed by open drainage if necessary. Empyemas following pneumonectomy, however, pose a special problem because there is no longer any lung to obliterate the infected space. In addition, postpneumonectomy empyemas frequently are associated with bronchopleural fistulas. In these patients, specific surgical procedures designed to obliterate residual intrathoracic spaces and in many cases close remaining bronchopleural fistulas may be required (Figure 18–10). In the absence of a bronchopleural fistula, sterilization and closure of a postpneumonectomy space (without obliteration) may be attempted using an irrigation catheter inserted into the apex of the chest cavity. An antibiotic solution specific for the organisms present is then infused into the chest. The solution is allowed to drain through a dependent tube or opening created by simple rib resection. After 2-8 weeks, the catheters are removed and the cavity is closed. The success rate with this technique is quite variable and is reported to be 20%-88%. For patients who fail this approach and for those patients with bronchopleural fistulas, the main goal of therapy is to obliterate the residual space and close any bronchopleural fistulas. This is most readily accomplished by the transposition of muscle with or without omentum into the empyema cavity. Multiple muscles may be required, including pectoralis major, latissimus dorsi, serratus anterior, intercostal muscle, and rectus abdominis (Figure 18–11). Use of these muscles is highly successful in closing any remaining bronchopleural fistulas and in completely obliterating the remaining intrathoracic space. The success of muscle flap closure of empyema spaces has made thoracoplasty (once a common procedure for reducing empyema spaces) a rare operation.
Antibiotics are an important adjunct in the treatment of empyemas, but it must be emphasized that drainage is the primary treatment modality. Although antibiotic therapy is always instituted early in the course of therapy when signs of systemic infection generally are present, they need not be continued once effective drainage is established. In fact, overuse of antibiotics may lead to the generation of resistant bacteria and therefore compromise the success of any subsequent procedures designed to obliterate residual intrathoracic space.
Blood in the pleural space usually occurs secondary to trauma, surgery, diagnostic or therapeutic procedures, neoplasms, pulmonary infarction, and infections (tuberculosis). Most hemothoraces can be treated effectively with large-bore (32-36F) closed chest tube drainage, particularly since small amounts of blood (occupying less than one-third of the hemithorax) are readily reabsorbed by the body. However, if significant blood clot has formed (occupying more than one-third of the hemithorax) or if secondary infection occurs, further measures must be taken to avoid the development of an empyema or fibrothorax with pulmonary compromise. Many hemothoraces requiring more than simple tube drainage can be managed with VATS procedures. Rarely, open thoracotomy may be required for complete decortication and evacuation.
Accumulation of chyle within the pleural space is most often due to surgical procedures, particularly cardiothoracic and esophageal operations. Trauma, malignancy, central venous catheterization, congenital lymphatic malformations, thoracic aortic aneurysms, filariasis, and cirrhosis may also rarely cause chylothorax. Penetrating thoracic trauma can lacerate the thoracic duct at any level, but blunt thoracic trauma usually causes a shearing of the duct at the right crus of the diaphragm. This may also occur with violent coughing or hyperextension of the spine. The initial treatment of chylothorax is similar to that of a malignant pleural effusion. Closed chest tube drainage is instituted; the lung is fully reexpanded; and a low-fat diet is started. In some cases, intravenous hyperalimentation (either peripheral or central) may greatly improve the patient’s condition. Some evidence supports the use of somatostatin to decrease the output from chylous effusions. The irritating nature of chyle promotes pleurodesis, and in half of patients the leak will stop spontaneously. The instillation of sclerosing agents (see section on pleural effusion, above) has also been advocated to increase the chances of success. If chyle continues to drain for more than 7 days or if significant drainage continues for even a shorter period of time, serious consideration should be given to operation since patients quickly become malnourished from the large associated protein losses. Video-assisted thoracoscopic techniques are usually ideal, making open thoracotomy rarely necessary. The standard approach is via the right chest, where the thoracic duct may be identified as it emerges from beneath the diaphragm between the aorta and the azygos vein. Ligation of the tissues in this area is usually all that is needed.
Air in the pleural space (pneumothorax) can occur as a result of a breach in either the parietal (trauma, esophageal perforation, surgery, etc) or visceral pleura (bulla, fine-needle aspirations, etc). Rarely, infections of the pleural space with gas-forming organisms may produce a pneumothorax. Since a chest radiograph is only a two-dimensional representation of a three-dimensional space, a relatively small separation of the pleural surfaces (eg, 1 cm) on a chest x-ray can translate into a relatively large pneumothorax. A large amount of intrapleural air that causes a shift of the mediastinum toward the contralateral lung is referred to as a tension pneumothorax. A pneumothorax associated with an open chest wound may be termed an open pneumothorax or sometimes a “sucking chest wound.” Tension and open pneumothoraces are surgical emergencies because both ventilation and venous return of blood to the heart are compromised. Intrapleural air may mix with blood, as frequently occurs after trauma (hemopneumothorax) or esophageal perforation (pyopneumothorax).
Pneumothoraces usually are classified as either spontaneous or acquired (those caused by a specific event such as trauma, invasive procedures, etc). Spontaneous pneumothoraces sometimes are divided into “primary” and “secondary” categories; however, all spontaneous pneumothoraces are secondary to some underlying pathologic process, and such a division is therefore strictly artificial. Most commonly, spontaneous pneumothoraces are caused by rupture of small subpleural blebs due to increased transpulmonary pressure most pronounced at the apex of the lung (apex of the upper lobe and superior segment of the lower lobe). Coughing, rapid falls in atmospheric pressure (> 10 millibars/24 h), rapid decompression (scuba divers), and high altitudes (jet pilots) all are associated with increased transpulmonary pressures and spontaneous pneumothorax. In addition, normal transpulmonary pressures can cause rupture of blebs in patients with connective tissue disorders such as Marfan syndrome. Other causes of spontaneous pneumothorax include apical bullae (patients with chronic obstructive pulmonary disease [COPD]), Pneumocystis pneumonia (patients with AIDS), metastatic cancer (particularly sarcomas), lymphangioleiomyomatosis, eosinophilic granuloma, rupture of the esophagus or of a lung abscess, cystic fibrosis, and menstruation (catamenial pneumothorax). Classically, however, spontaneous pneumothoraces occur in asthenic males (male-to-female ratio 6:1) between the ages of 16 and 24, often with a history of smoking. The true incidence is unknown, since up to 20% of patients remain asymptomatic and do not seek medical attention.
Patients with pneumothoraces complain of pleuritic chest pain and dyspnea. If severe underlying cardiopulmonary disease exists or if a tension pneumothorax develops, symptoms become much more dramatic and include diaphoresis, cyanosis, weakness, and symptoms of hypotension and cardiovascular collapse. Physical examination reveals tachypnea, tachycardia, deviation of the trachea away from the involved side (tension pneumothorax), decreased breath sounds, hyperresonance, and diminished vocal fremitus on the involved side. Arterial blood gases may demonstrate hypoxia and occasionally hypocapnia from hyperventilation, and the EKG may show axis deviations, nonspecific ST segment changes, and T wave inversion. The standard test for the diagnosis of pneumothoraces is the posteroanterior (PA) and lateral chest radiograph. Exhalation accentuates the contrast between the collapsed lung and the intrapleural air as well as the magnitude of the collapse. Rarely, a CT scan may be necessary to differentiate a pneumothorax from a large bulla in patients with severe emphysema. In 5%-10% of patients, a small pleural effusion may be present and can be hemorrhagic.
The treatment of spontaneous pneumothoraces varies depending on the patient’s symptoms and condition, the degree of collapse, the cause, and the estimate of the chance of recurrence. Small (< 20%-25%), stable, asymptomatic pneumothoraces in otherwise healthy patients can be followed (often on an outpatient basis) with the expectation of complete resolution within several weeks, since air is normally reabsorbed at a rate of 1%-1.25% per day. Larger asymptomatic pneumothoraces taking longer than 2-3 weeks to resolve place the patient at risk for developing trapped lung as a result of deposition of fibrin on the visceral pleura. These patients—as well as patients with symptoms, increasing pneumothoraces, or pneumothoraces associated with pleural effusions—should have them evacuated. In highly selected patients, this can be accomplished with simple aspiration as long as the immediate and 2-hour delayed chest radiographs document reexpansion. It should be emphasized, however, that some small breaks in the visceral pleural seal once the lung collapses and can reopen with reexpansion. The chance of recurrence is 20%-50% with this method, and follow-up x-ray is therefore mandatory after 24 hours.
Most patients with significant pneumothoraces (> 30%) require placement of a closed-chest catheter (8-20F) for acceptable reexpansion. This catheter then can be placed either to underwater suction drainage or to a Heimlich (one-way) valve. If a Heimlich valve maintains full expansion, the patient may be treated as an outpatient; however, if a Heimlich valve fails to reexpand the lung fully or if the patient’s condition is not optimal, admission to hospital and underwater chest tube suction drainage is required. Unless some contraindication exists, chest tubes should be placed in the midaxillary line at the level of the fifth intercostal space (nipple line). In women, the breast tissue should be retracted medially and avoided in the dissection to the chest wall. Placement with the use of blunt clamp dissection avoids the dangers of trocar insertion and should almost always be used. Following resolution of any air leakage, the tube may be taken off suction (water seal) and removed if the lung remains fully inflated. In patients with classic spontaneous pneumothoraces, the chance of recurrence increases with each episode. Following a single episode, the risk of a recurrent pneumothorax is 40%-50%. After two episodes, the risk increases to 50%-75%, and with three previous episodes, the risk is in excess of 80%. Currently, most first-time patients are treated initially with simple chest tube drainage; however, with subsequent recurrences, additional therapy generally is indicated. Furthermore, with the development of VATS, some feel that a more aggressive approach should be taken even for first-time pneumothoraces.
Patients with air leaks lasting longer than 7 days, patients who do not fully reexpand their lungs, patients in high-risk occupations (scuba divers, airline pilots, etc), patients with large bullae or poor pulmonary function, and patients with bilateral or recurrent pneumothoraces are candidates for additional medical (pleurodesis) or surgical intervention. Furthermore, patients who frequently travel to places distant from medical care are offered early surgical intervention. Previously, tetracycline pleurodesis was used to decrease the incidence of recurrent pneumothoraces. The use of this substance, however, was associated with significant pain and controversy. It is currently no longer available. The use of talc slurry or powder in this setting is also controversial due to the potential for long-term fibrothorax and restrictive lung disease but has been shown to reduce the recurrence rate to as low as 2%. Many other chemical agents have been used in the past, including mechlorethamine, doxycycline, iodoform, guaiacol, urea, and hypertonic glucose, with varying success rates. Since pleurodesis can make subsequent surgical procedures more difficult, the use of this treatment option continues to generate controversy.
Medically fit patients who are candidates for pleurodesis are also candidates for operation. The procedures used to prevent recurrent pneumothoraces include: (1) axillary thoracotomy with apical bullectomy, mechanical pleurodesis, and partial pleurectomy; and (2) complete parietal pleurectomy. Both procedures can be performed with either open or VATS techniques. Complete parietal pleurectomy generally is avoided, since some patients may require future thoracic surgical procedures that are extremely difficult in the face of total parietal pleurectomy. Apical bullectomy, mechanical pleurodesis, and partial apical pleurectomy have been shown to reduce the recurrence rate to near zero. In addition, this procedure is easily accomplished either with VATS techniques or through a small transaxillary thoracotomy, both of which are well tolerated.
Several special situations exist that require particular expertise in treatment decisions. Patients with cystic fibrosis and severe COPD may be candidates for lung transplantation, and both pleurodesis and operation may make subsequent transplantation more dangerous. Therefore, consultation with a transplant surgeon is advisable prior to considering these therapies. AIDS patients with Pneumocystis pneumonia and pneumothorax are extremely difficult to manage, having a high rate of persistent bronchopleural fistula, treatment failure, and death. For optimal management, the pulmonary surgeon should have extensive experience in the management of chest catheters.
Primary pleural tumors are uncommon neoplasms of two main types: diffuse malignant pleural mesotheliomas and localized fibrous tumors of the pleura (previously referred to as localized mesotheliomas). Although diffuse malignant pleural mesothelioma is the most common primary pleural tumor, involvement of the pleura with metastatic disease is more frequent and represents the most likely cause of any newly diagnosed pleural malignancy.
Localized fibrous tumors of the pleura arise from subpleural fibroblasts than produce an array of lesions varying from peripheral pulmonary nodules to sessile subpleural masses to the more typical large pedunculated neoplasms. The visceral pleura is involved more often than the parietal pleural, and both benign (70%) and malignant (30%) variations exist. Histologically, benign tumors can exhibit three patterns—fibrous, cellular, and mixed—while malignant ones also have three distinct appearances: tubulopapillary, fibrous, and dimorphic. These tumors behave more like sarcomas of the pleura than diffuse malignant mesotheliomas. Most localized fibrous tumors of the pleura are asymptomatic, discovered only incidentally on chest radiography. Extremely large tumors, however, may produce symptoms of bronchial compression with dyspnea, cough, and chest heaviness—and, rarely, symptoms of hypoglycemia from the production of an insulin-like peptide (4% of patients). On physical examination, signs of clubbing and hypertrophic pulmonary osteoarthropathy (20%-35%) may be present. Chest radiography most often demonstrates a well-circumscribed mass that may move with changes in position if the tumor is pedunculated. A pleural effusion is present in 15% of cases and can be bloody, though this does not indicate unresectability. Fine-needle aspiration cytology may be suggestive; however, the diagnosis generally can be established with certainty only at surgery.
The treatment of these lesions is a complete resection. Although lobectomy is usually not required for lesions involving the visceral pleura, wedge resection of the pulmonary parenchyma in the area of the tumor is recommended. For neoplasms arising from the parietal pleura, chest wall resection is prudent. Following complete surgical excision, no further therapy is indicated and the prognosis is good, with some patients surviving for over 10 years without recurrence; however, if the resection is incomplete, radiation therapy should be contemplated because the prognosis is poor, with a median survival of only 7 months.
Diffuse malignant pleural mesothelioma is the most common primary tumor of the pleura. Since 1960, the disorder has been strongly linked to the use of asbestos. Thick serpentine asbestos fibers (chrysotile) generally are deposited in the proximal airways and are easily cleared with less risk of the development of tumors; however, thin needle-like amphibole fibers (crocidolite, amosite, actinolite, anthophyllite, and tremolite) and the soil silicate zeolite, found in the Anatolia region of Turkey, usually lodge in the terminal airways and migrate to the pleura, thereby increasing the risk of diffuse malignant pleural mesothelioma to more than 300 times that of the general population. The increased incidence of mesothelioma in shipbuilders exposed to asbestos-laden insulation from World War II-era ships further implicates asbestosis in the pathophysiology of the disease. The latency period after exposure ranges from 15 to 50 years. Recent research suggests that the generation of free radicals (including nitric oxide), depression of the immune system (both cellular and humoral), induction of cytokines (tumor necrosis factor [TNF]-α, interleukin [IL]-1α, IL-1β, and IL-6), and the production of genetic defects, such as abnormalities of chromosomes 1, 3, 4, 6, 7, 9, 11, 17 (p53), and 22 (involves c-sis, which encodes for one chain of platelet-derived growth factor) all may play a role in the mechanism of asbestos-related disease.
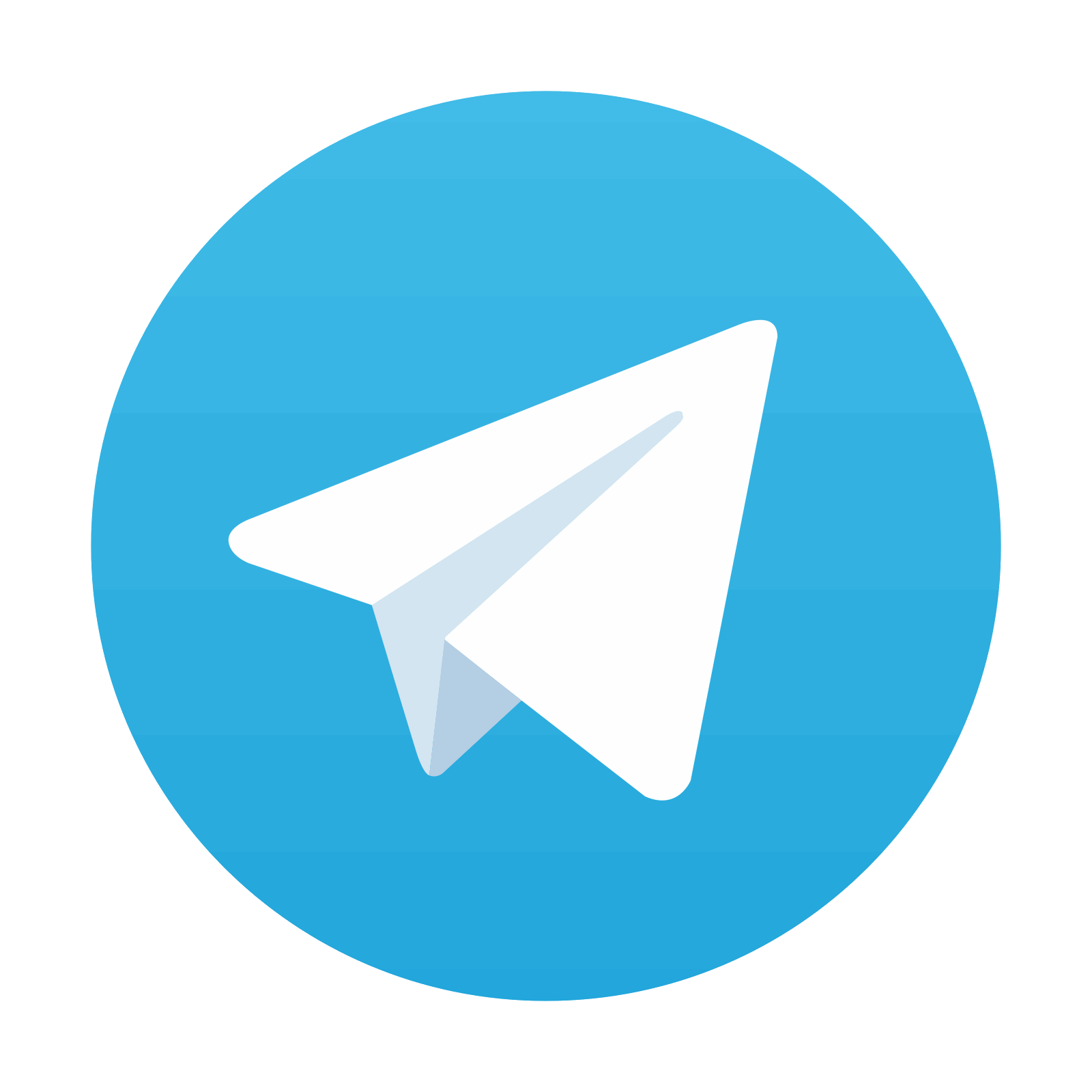
Stay updated, free articles. Join our Telegram channel

Full access? Get Clinical Tree
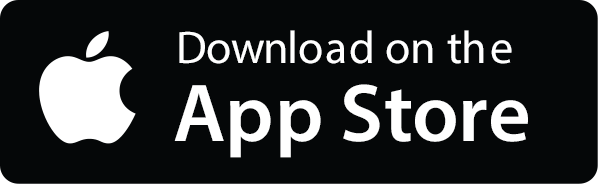
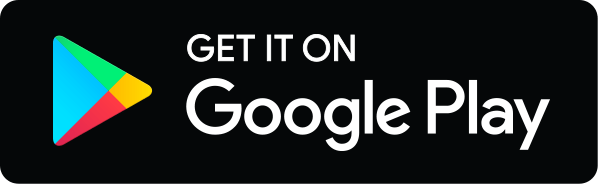