In this section, we outline the potential, methods, and probable limitations of gene transfer for the treatment of human genetic disease. The minimal requirements that must be met before the use of gene transfer can be considered for the treatment of a genetic disorder are presented in the Box.
Essential Requirements of Gene Therapy for an Inherited Disorder
The identity of the affected gene must be known.
A complementary DNA (cDNA) clone of the gene or the gene itself must be available. If the gene or cDNA is too large for the current generation of vectors, a functional version of the gene from which nonessential components have been removed to reduce its size may suffice.
The most commonly used vectors at present are derived from the adeno-associated viruses (AAVs) or retroviruses, including lentivirus.
Knowledge of the pathophysiological mechanism of the disease must be sufficient to suggest that the gene transfer will ameliorate or correct the pathological process and prevent, slow, or reverse critical phenotypic abnormalities. Loss-of-function mutations require replacement with a functional gene; for diseases due to dominant negative alleles, inactivation of the mutant gene or its products will be necessary.
A substantial disease burden and a favorable risk-to-benefit ratio, in comparison with alternative therapies, must be present.
Tight regulation of the level of gene expression is relatively unimportant in some diseases and critical in others. In thalassemia, for example, overexpression of the transferred gene would cause a new imbalance of globin chains in red blood cells, whereas low levels of expression would be ineffective. In some enzymopathies, a few percent of normal expression may be therapeutic, and abnormally high levels of expression may have no adverse effect.
Ideally, the target cell must have a long half-life or good replicative potential in vivo. It must also be accessible for direct introduction of the gene or, alternatively, it must be possible to deliver sufficient copies of the gene to it (e.g., through the bloodstream) to attain a therapeutic benefit. The feasibility of gene therapy is often enhanced if the target cell can be cultured in vitro to facilitate gene transfer into it; in this case, it must be possible to introduce a sufficient number of the recipient cells into the patient and have them functionally integrate into the relevant organ.
Cultured cell and animal studies must indicate that the vector and gene construct are both effective and safe. The ideal precedent is to show that the gene therapy is effective, benign, and enduring in a large animal genetic model of the disease in question. At present, however, large animal models exist for only a few monogenic diseases. Genetically engineered or spontaneous mutant mouse models are much more widely available.
Protocol review and approval by an institutional review board are essential. In most countries, human gene therapy trials are also subject to oversight by a governmental agency.
General Considerations for Gene Therapy
In the treatment of inherited disease, the most common use of gene therapy will be the introduction of functional copies of the relevant gene into the appropriate target cells of a patient with a loss-of-function mutation (because most genetic diseases result from such mutations).
In these instances, precisely where the transferred gene inserts into the genome of a cell would, in principle, generally not be important (see later discussion). If gene editing (see earlier discussion and Table 13-4) to treat inherited disease becomes possible, then correction of the defect in the mutant gene in its normal genomic context would be ideal and would alleviate concerns such as the activation of a nearby oncogene by the regulatory activity of a viral vector, or the inactivation of a tumor suppressor due to insertional mutagenesis by the vector. In some long-lived types of cells, stable, long-term expression may not require integration of the introduced gene into the host genome. For example, if the transferred gene is stabilized in the form of an episome (a stable nuclear but nonchromosomal DNA molecule, such as that formed by an adeno-associated viral vector, discussed later), and if the target cell is long-lived (e.g., T cells, neurons, myocytes, hepatocytes), then long-term expression can occur without integration.
Gene therapy may also be undertaken to inactivate the product of a dominant mutant allele whose abnormal product causes the disease. For example, vectors carrying siRNAs (see earlier section) could, in principle, be used to mediate the selective degradation of a mutant mRNA encoding a dominant negative proα1(I) collagen that causes osteogenesis imperfecta (see Chapter 12).
Gene Transfer Strategies
An appropriately engineered gene may be transferred into target cells by one of two general strategies (Fig. 13-16). The first involves introduction of the gene into cells that have been cultured from the patient ex vivo (that is, outside the body) and then reintroduction of the cells to the patient after the gene transfer. In the second approach, the gene is injected directly in vivo into the tissue or extracellular fluid of interest (from which it is taken up by the target cells). In some cases, it may be desirable to target the vector to a specific cell type; this is usually achieved by modifying the coat of a viral vector so that only the designated cells bind the viral particles.
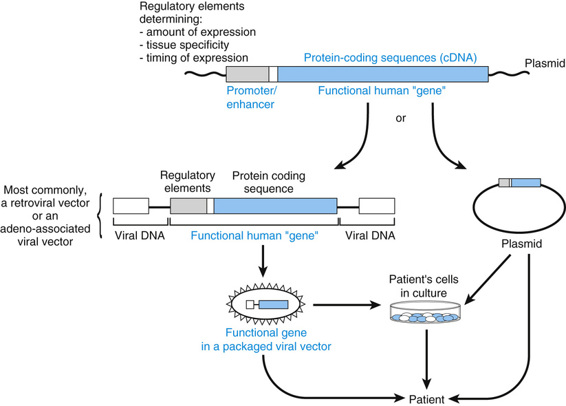
The Target Cell
The ideal target cells are stem cells (which are self-replicating) or progenitor cells taken from the patient (thereby eliminating the risk for graft-versus-host disease); both cell types have substantial replication potential. Introduction of the gene into stem cells can result in the expression of the transferred gene in a large population of daughter cells. At present, bone marrow is the only tissue whose stem cells have been successfully targeted as recipients of transferred genes. Genetically modified bone marrow stem cells have been used to cure two forms of SCID, as discussed later. Gene transfer therapy into blood stem cells is also likely to be effective for the treatment of hemoglobinopathies and storage diseases for which bone marrow transplantation has been effective, as discussed earlier.
An important logistical consideration is the number of cells into which the gene must be introduced in order to have a significant therapeutic effect. To treat PKU, for example, the approximate number of liver cells into which the phenylalanine hydroxylase gene would have to be transferred is approximately 5% of the hepatocyte mass, or approximately 1010 cells, although this number could be much less if the level of expression of the transferred gene is higher than wild type. A much greater challenge is gene therapy for muscular dystrophies, for which the gene must be inserted into a significant fraction of the huge number of myocytes in the body in order to have therapeutic efficacy.
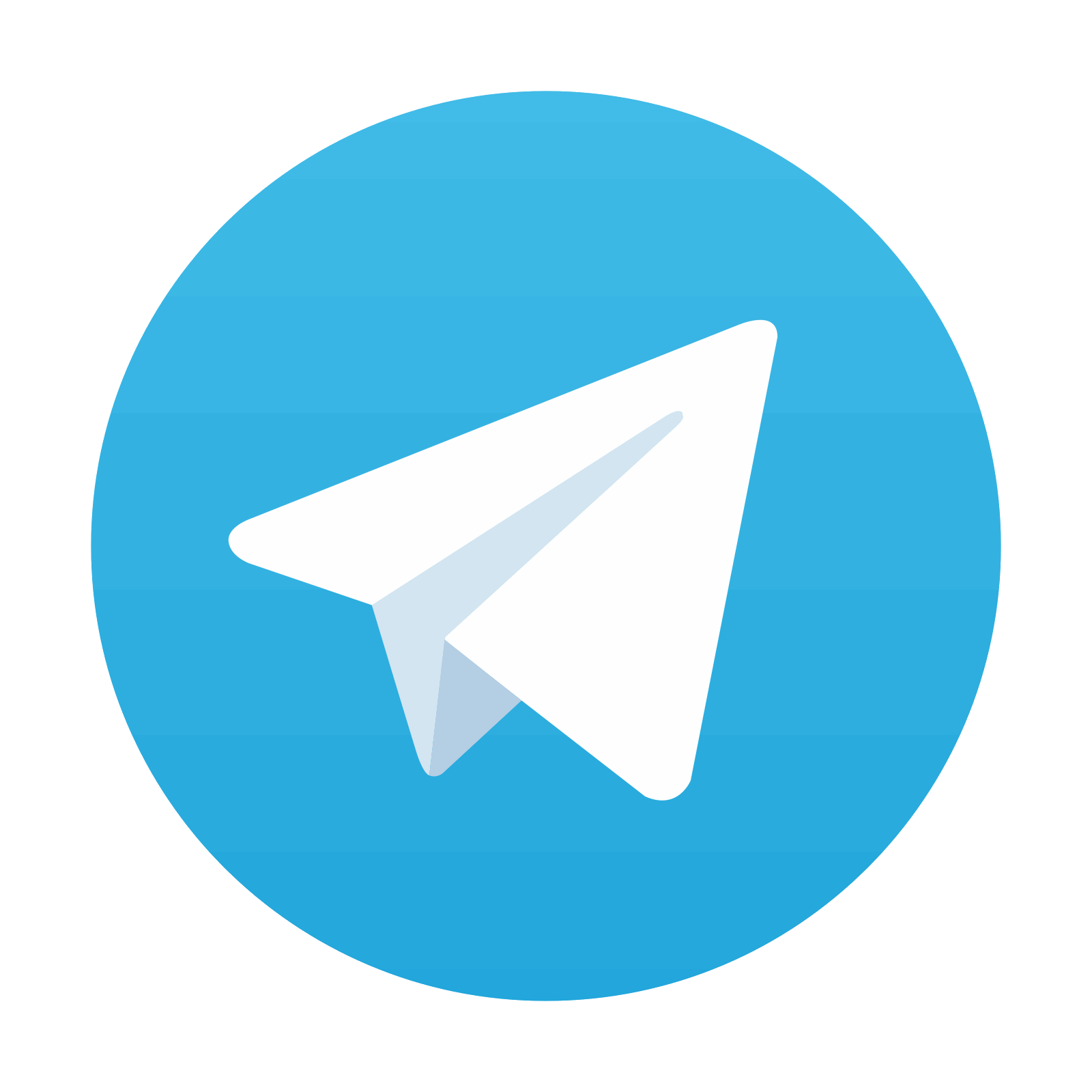
Stay updated, free articles. Join our Telegram channel

Full access? Get Clinical Tree
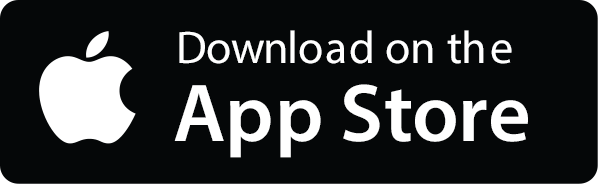
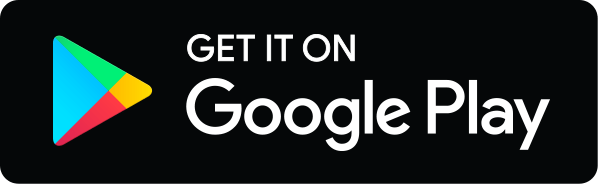