Therapeutic Regimens
Therapeutic Window
OBJECTIVES
The reader will be able to:
- Define the terms: dosage regimen, therapeutic effectiveness, therapeutic index, therapeutic utility, therapeutic utility curve, therapeutic window.
- List the factors that determine the dosage regimen of a drug.
- Explain the strategy behind the establishment of a therapeutic window.
- List the range of plasma concentrations associated with therapy within the target patient population for five of the drugs given in Table 9-1.
- Discuss briefly situations in which poor systemic exposure–response relationships may occur.
DOSAGE REGIMENS
The method of drug administration comprising dosage form, dosing rate, size of dose, interval between doses, route of administration, and duration of therapy is called a dosage regimen. All substances produce harmful effects if given in high enough amounts, drugs being no exception. Therapeutic dosage regimens are designed to optimize therapy by balancing the risk of ill effects against the benefits achievable with the drug.
In this section, Therapeutic Regimens, fundamental aspects of dosage regimens are covered primarily from the point of view of treating a typical patient within the target population with a given disease or condition. It is realized, of course, that individuals vary in their responses to drugs; subsequently, in Section IV, Individualization, focus is turned toward optimizing dosage regimens in individual patients.
A dosage regimen is basically derived from the kinds of information shown in Fig. 9-1. This scheme applies essentially to all drugs but particularly to those intended to produce therapeutic effects systemically; for locally acting drugs, local exposure is critical and systemic exposure is more of a safety concern. One consideration is how the body acts on the drug and its dosage form, the essence of pharmacokinetics. Another consideration includes those factors that relate systemic exposure to both efficacy and safety of the drug, pharmacodynamic factors. A third consideration is the clinical state of the patient, which can affect both the pharmacokinetics and the pharmacodynamics of a drug. This includes the disease or condition being treated, other diseases or conditions that the patient may have, as well as associated additional drug therapy—that is, his or her total therapeutic regimen. A fourth consideration includes all other factors such as genetics, age and weight, and the extent of adherence by the patient to the dosage regimen. Many of these determinants are interrelated and interdependent. For example, the requirement of frequent dosing can reduce adherence to a regimen and influence clinical outcome, whereas clinical outcome, particularly adverse effects without perceived benefit, can influence adherence even when maintenance of the regimen is critical to the ultimate therapeutic benefit. Also critical to our understanding and to the optimal design and application of dosage regimens are changes in dosage requirements with time. The status of a patient is never static and, in addition to changes that occur soon after drug administration, there are often progressive changes in the disease or condition over the time span of treatment, particularly when the treatment extends over many months, years, or the rest of one’s life. Such changes need to be taken into account when assessing the effectiveness and safety of the drug.
FIGURE 9-1. A dosage regimen of drug comprises dose, dosage form, dosing interval, route of administration, and duration of therapy. These factors are adjusted to achieve an exposure profile that maximizes the benefits compared with adverse effects of the drug. Clinical factors that determine the dosage regimen within a target population include the disease or condition being treated, the presence of other diseases and conditions, and other concomitantly administered drugs. These and other factors affect the regimen by influencing the drug’s pharmacokinetics, pharmacodynamics, or both.
Dosage regimens involve using various modalities of drug administration, extending from a single or occasional dose to continuous and constant input. An example of the former is the use of aspirin to treat an occasional headache; the continuous intravenous infusion of heparin to maintain a desired degree of anticoagulation in a patient with deep vein thrombosis is an example of the latter. More commonly, drugs are administered repeatedly in discrete doses. The frequency and duration vary with the condition being treated. For many drugs, maintenance of a relatively constant systemic exposure is needed to maintain a therapeutic effect, which may, for example, be achieved by giving the drug three times daily during the treatment. Other drugs do not require such a strict maintenance of systemic exposure and can be given relatively infrequently, which results in larger fluctuations in the systemic exposure–time profile. Reasons for this latter approach being therapeutically desirable include the development of tolerance to the desired effect of the drug if exposure is maintained; the need to produce high exposure for relatively short periods of time, as occurs in some antibiotic and anticancer chemotherapies; and persistence of drug effect even when the drug has been eliminated, as discussed in Chapter 8, Response Following a Single Dose. In all cases, the intent is to achieve effective therapy as safely as possible.
In this chapter, various elements of the dose–response and systemic exposure–response relationships in the context of dosage regimens are explored; these build on principles developed in Chapter 2, Fundamental Concepts and Terminology and Chapter 8, Response Following a Single Dose. Principles for achieving a desired exposure–time profile are discussed in the subsequent two chapters of this section, Constant-Rate Input (Chapter 10) and Multiple-Dose Regimens (Chapter 11).
THERAPEUTIC EXPOSURE
Historically, the range of doses of a drug initially tested in patients has been almost entirely empirical, observing desired and adverse effects and adjusting doses accordingly. Today, the choice of test doses is increasingly based on a combination of prior information on the pharmacokinetics of the drug and concentration–response relationships derived from in vitro and animal studies. However, much uncertainty still remains that can only be addressed through experimentation in the target patient population. During early clinical evaluation, usually involving a few hundred patients at most, detailed systemic exposure–response relationships are developed, which help to define a range of therapeutic useful dosage regimens of a drug. Often, however, much more limited systemic exposure data are gathered during the subsequent large-scale clinical trials, generally involving many thousands of patients. The therapeutic window between ineffective therapy and excessive adverse effects is then defined clinically in terms of the doses of drug administered rather than plasma concentration.
Figure 9-2 displays data that typically might be found for a drug evaluated within the target patient population. Shown are the percent of the patients who did not respond, those who experienced a beneficial effect, those who experienced minor adverse effects, and those who experienced severe adverse responses following daily administration of either a placebo or a 10-, 20-, or 40-mg dose of a drug to different cohorts of patients. As is commonly the case, the drug was considered effective when the desired response reached a given endpoint, such as a lowering of systolic blood pressure by at least 10 mm Hg in hypertensive patients. Adverse effects were considered minor, such as an occasional transient headache, and severe, when the harmful effects necessitated discontinuation of the drug. In practice, there may be finer divisions of adverse effects, such as minor, moderate, serious, and severe.
FIGURE 9-2. A. Frequencies (percent of patients on each treatment) of ineffective therapy (white), effective therapy (colored), minor adverse effects (gray), and severe toxicity (black) observed in a target population in which patients were assigned randomly to receive: a placebo, 10 mg, 20 mg, or 40 mg of a drug once daily. B. The incidence of “therapeutic effectiveness” for the four groups of patients displayed in panel A. Therapeutic effectiveness is defined arbitrarily as the difference between the frequency of effective therapy and the frequency of all harmful effects; the therapeutic effectiveness of this drug reaches a maximum at 20 mg.
First, notice that, as is common in clinical practice, a graded response, which is lowering of blood pressure, has been transformed into a quantal response by setting a fixed endpoint as a measure of effectiveness. Along similar lines, an adverse effect, such as increase in heart rate, tachycardia, which is graded, is often divided into degrees of severity, and obviously, a person can only be in one category at a time. Accordingly, it is the sum of all such adverse events that has a maximum probability of 1. Second, it is not uncommon that even on placebo, some patients perceive benefit and some adverse effects even though they have not been exposed to drug. Third, some patients fail to gain clinical benefit even at the highest dose. Next, the incidences of beneficial and harmful responses are all significantly greater in those patients receiving the drug than those receiving the placebo, with the incidence of increasing responses as the daily dose increases. Such information provides an estimate of the likelihood of a patient to experience effective treatment without adverse effects, by subtracting, for example, the sum of the incidences of the adverse responses from that of the beneficial one, a measure of the therapeutic effectiveness of the drug (Fig. 9-2B). This difference measure is greater for all doses tested than for placebo, suggesting the drug has merit over the placebo. It also increases up to 20-mg dose and then declines at the 40-mg dose, because the increase in the incidence of harmful effects exceeds that of the beneficial one, suggesting that the optimal dose is 20 mg daily. However, in practice, due regard also needs to be taken of the relative weight to be assigned to measures of benefit and harm when estimating the dose or window of doses, for which therapeutic success is most probable. Obviously, severe adverse effects should be given more weight than minor or moderate ones. For this drug, the incidence may be considered to be so frequent at 40 mg that the upper dose should be limited to 20 mg.
Although helpful in exploring doses, this approach to understanding the therapeutic value of the drug leaves many questions unanswered. Why, for example, did some patients fail to respond beneficially even at the highest dose tested? And, why did others experience benefit with minimal adverse effects even at the highest dose, whereas others experienced severe adverse effects at the 20-mg dose?
These and other questions are often better addressed by exploring response as a function of systemic exposure to the drug, rather than dose administered. Certainly, systemic exposure is better than dose after a single dose of drug, when intensity of a response varies with time, especially for an acute response that correlates directly with drug concentration at all times, because with dose alone, time is not taken into account. It may also be true for long-term drug administration but for a different reason.
The objective of much of drug therapy is to maintain a stable therapeutic response for the duration of treatment, usually by maintaining the same daily dosage of drug. Recalling Fig. 1-7 (Chapter 1, Therapeutic Relevance, page 9), it shows the relationship between the plasma concentration and the daily dose/kg body weight of the antiepileptic drug, phenytoin, in a patient population receiving the drug chronically. Notice the large interindividual differences in the plasma concentration at any given daily dose; in the patient cohort studied, the plasma concentration ranged from nearly 0 to 50 mg/L when the daily dose was 6 mg/kg. Had no correction been made for body weight, the deviations at a given dose might have been even greater if body weight captured some of the variability in the pharmacokinetics of the drug. In contrast, plasma concentration correlates reasonably well with effect. Thus, seizures are usually effectively controlled without undue adverse events at plasma phenytoin concentrations between 10 and 20 mg/L. For this drug, plasma concentration is a better correlate than dose during chronic administration because pharmacokinetics is the major source of variability between dose and response. For other drugs, such as atenolol, used to lower blood pressure, pharmacokinetic variability within the patient population is relatively small, and variability in the concentration–response curve is large. In such cases, plasma concentration is no better a correlate with response than dose and indeed may be worse if, for example, metabolites contribute to activity and toxicity (see “Additional Considerations” later). Even then, understanding systemic exposure–response relationships is critically important in anticipating therapeutic outcome when the systemic exposure changes dramatically despite maintaining dose, for example, during some drug interactions and in the presence of diseases, such as hepatic and renal function impairment that may markedly diminish clearance. Moreover, without exploring the systemic exposure–response relationships, one can never know where the source of the major variability lies and how to develop methods for accommodating for it.
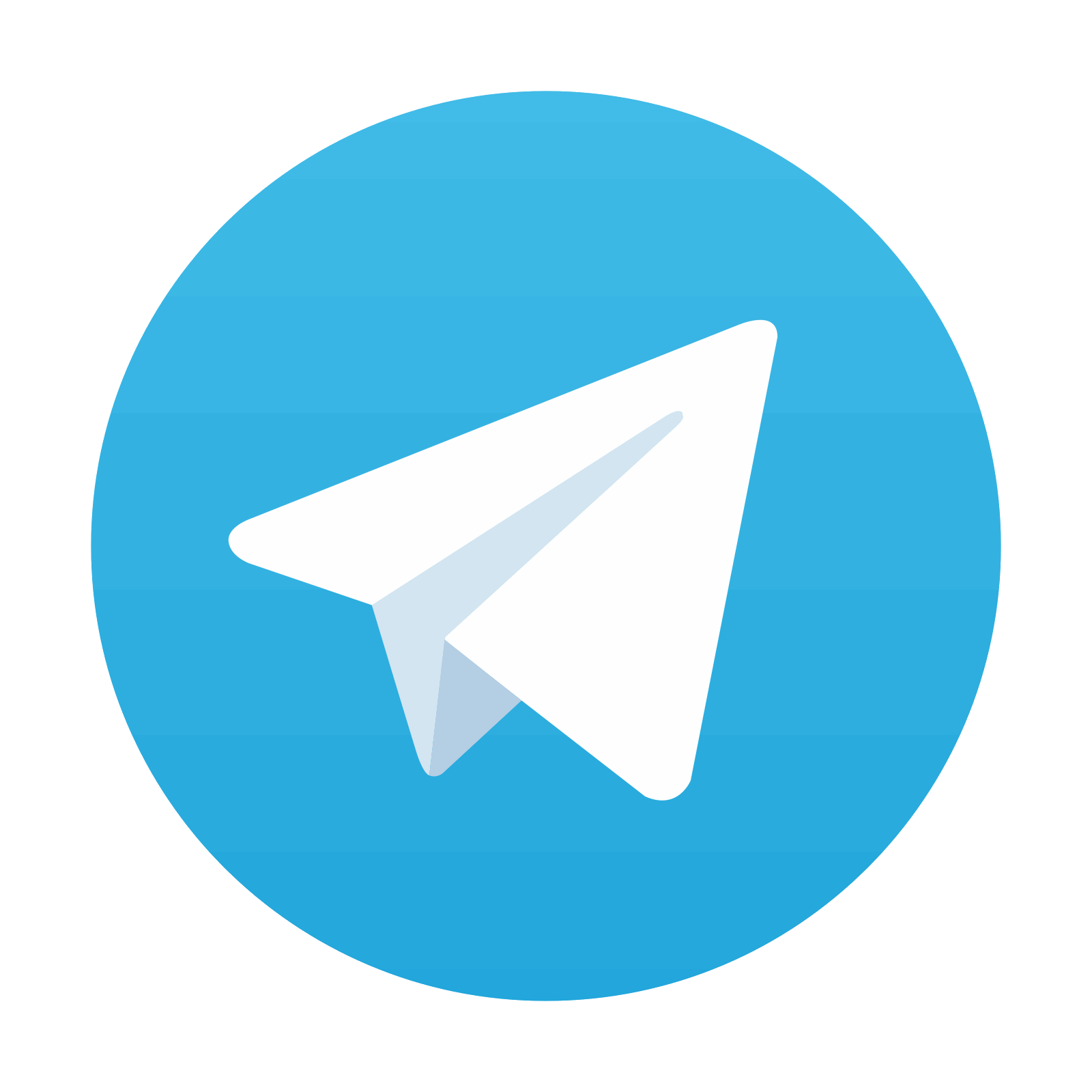
Stay updated, free articles. Join our Telegram channel

Full access? Get Clinical Tree
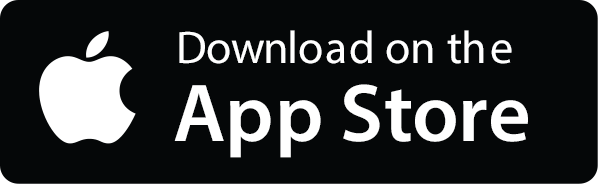
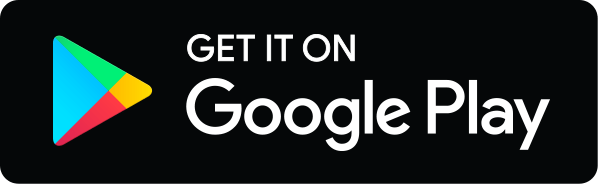