Vaccine
Biotechnology company
Pharmaceutical codevelopment partner
Development stage attained
Development status
TA-NIC
Celtic (formerly Xenova)
−
Phase II
Halted
NicQβ
Cytos Biotechnology
Novartis
Phase IIb
Halted
NicVAX
Nabi Biopharmaceuticals
GlaxoSmithKline
Phase III
Halted
Niccine
Independent Pharmaceutical
−
Phase II
Halted
SEL–068
Selecta Biosciences
−
Phase I
Reformulation needed
NIC7
Pfizer
n/a
Phase I
Ongoing; results expected 1H 2016
2.3.1 More Recent Clinical Progress and a Major Late-Stage Failure
The original promising animal study results (Hartmann-Boyce et al. 2012) led to initially encouraging early-stage clinical studies where NicQβ and NicVAX both demonstrated a significant association between high levels of anti-nicotine antibodies (nic · IgG1) and long-term abstinence from smoking, when the vaccines were administered in smoking cessation phase II studies in the mid-2000’s. The increased long-term abstinence was observed when high-antibody (Ab) responders (approximately the top-third) were compared to the low-Ab responders (the lower two-thirds), and as well when compared to placebo.
In 2010 and 2011, NicVAX was tested as an aid to smoking cessation treatment in two nearly identical 1000-subject randomized, placebo-controlled, multi-center phase III clinical studies. The most promising vaccine at the time and the only nicotine vaccine to reach the Phase III clinical testing stage was shown to have similar efficacy to placebo (Fahim, et al. 2011).
As a consequence of these negative results many of the world’s experts and many of the investigators who are authors in this book took a step back and thoroughly assessed the field of therapeutic vaccines for treating nicotine addiction. Several comprehensive and insightful reviews (Moreno and Janda 2009; Hartmann-Boyce et al. 2012; Fahim et al. 2011, 2013; Raupach et al. 2012; Kosten and Domingo 2013; Pentel and LeSage 2014; Kinsey 2014) have generally concluded that the basic scientific principles underlying the PK mechanism are sound. Furthermore, the broad range of preclinical and clinical findings for vaccines and monoclonal antibodies (mAbs) targeting other drugs of abuse, support the continued research and development of therapeutic vaccines for treating addiction, in spite of the NicVAX phase III trial outcomes. Better technologies and platforms aimed at improving the immunogenicity of the vaccines are needed to increase the quantity and quality (high-affinity and high-avidity) nic · IgG elicited by the vast majority of vaccinated smokers.
2.3.2 Mechanism of Action
The molecular mechanism of action of nicotine-specific Abs is best understood as an alteration of the pharmacokinetics of nicotine leading to reduced brain nicotine levels and increased blood levels of nicotine (Gorelick 2012). In general, slower drug delivery to the brain is associated with less intense and less reinforcing effects and thus attenuation of nicotine’s addictive potential (Henningfield and Keenan 1993; deWit and Zacny 1995). This is particularly important with respect to nicotine as its greatest reinforcing and subjective effects occur within the first few minutes of smoking (Henningfield et al. 1985).
The nicotine in the blood is the target of the antibody, rather than the nicotinic acetylcholine receptors in the brain current pharmacotherapies target. Antibody binding of nicotine results in a substantial decrease in the rate of rise of nicotine levels in the brain and in lower doses of nicotine accessing neural reward targets, therefore reducing the addictive potential of the drug. Nicotine-specific IgGs have been shown to bind nicotine in the blood: slowing and reducing its distribution to the brain as the antibody-bound nicotine is too large to cross the blood-brain barrier (Hieda et al. 1999).
In summary, nicotine-specific Ab’s lead to a lowering of the peak nicotine brain levels (reduced C max) concurrently with a delay of reaching those peak levels (longer T max). This causes blunting of the initial spike of nicotine entering the brain and flattening of the concentration-time course, leading to an overall reduction of the amount of nicotine reaching the brain from a smoked cigarette or other modes of delivery (reduced AUC), thereby reducing the addictive potential of nicotine (the “rate hypothesis” of psychoactive drug addiction) (Porchet et al. 1987; Nelson et al. 2006).
2.3.3 Early Clinical Success (Phase I and II)
The highest vaccine dose group in the NicVAX phase IIb study is the only reported nicotine vaccine treatment arm to demonstrate clinical efficacy prospectively, without stratification by nic · IgG level (NCT00318383). The intent-to-treat analysis indicated that the 5-injection 400 μg NicVAX dose group had significantly higher prolonged abstinence to 6 months as compared to placebo (17.6 % vs. 6.0 %; p = 0.015; OR of 4.14; 95 % CI, 1.32–13.02) (Hatsukami et al. 2011). The observation of an association between high levels of nic · IgG and long-term abstinence for two independently developed nicotine vaccines provided sufficiently compelling clinical “proof of mechanism” that three global pharmaceutical companies, Novartis, GlaxoSmithKline, and Pfizer, entered the field between 2007 and 2009 (Table 2.1). Novartis and GlaxoSmithKline entered codevelopment partnerships with Cytos and Nabi, respectively, while Pfizer chose to start a new program based upon the learnings from the first generation of vaccine candidates.
2.3.4 Late-Stage Clinical Setbacks
Failure to reproduce these results in large pivotal studies has plagued the field since 2010: clinical development of all first-generation nicotine conjugate vaccines has been halted due to the lack of clinical efficacy as compared to placebo, in the intent-to-treat population (TA-NIC, NicQβ, Niccine, and NicVAX). All four of the biotechnology companies developing these four nicotine vaccines no longer exist or have substantially shifted their therapeutic focus. Novartis and GlaxoSmithKline have exited from visible nicotine therapeutic development efforts.
Upon failure of a late-stage clinical study, financial considerations exert a strong influence to rapidly halt the failed effort, particularly in the microcap biotechnology environment where the cost of capital is very high. Unfortunately, this severely limits the availability of resources for exploratory analyses that otherwise could have uncovered new insights into the fundamental biochemical and clinical hurdles encountered. Oftentimes, these pressures prevent the primary study results and postmortem analyses that had been conducted to be published. These insights are what are needed to fuel the next iteration of innovative vaccine designs. In an exception to the rule, exploratory post hoc analyses of the unpublished NicVAX phase III clinic trials have recently entered the public domain via an abandoned patent application (Fahim and Kalnik 2012). These analyses will be described in the following section.
2.4 Correlation of Nicotine-Specific Abs with Abstinence Rates
In spite of these setbacks, insights regarding the putative threshold of high-affinity nicotine-specific Abs needed to support smoking cessation and long-term abstinence have been gained. Elegant preclinical experiments utilizing nicotine-specific mAbs to overcome the inherent variability of biological immune response have provided significant insight into the range of doses and preferred affinities of nic · IgG likely needed vaccination to be effective.
2.4.1 Dose-Response and Affinity-Response Relationships of Nicotine-Specific mAbs: In Vivo
Three independent laboratories have generated and characterized nicotine-specific monoclonal antibodies for the treatment of nicotine addiction (Scripps, NIC9D9 from the NIC-KLH vaccine; Pentel/Nabi, Nic311 from NicVAX; and Cytos, Nic12 from NicQβ). Nicotine mAbs (nic · mAbs) can be produced that have uniform affinities and dose amounts, whereas the amount and quality of nic · IgG arising from vaccination are subject to interindividual variability of vaccine response with ranges spanning atleast two orders of magnitude. Furthermore, nic · mAbs can be administered immediately when smokers who want to quit at that moment can begin their smoking cessation efforts when their motivation is highest. Vaccines require a priming series of injections lasting weeks to months before high-affinity nic · IgGs are elicited in high quantities, when the smoker’s motivation to quit may have subsided (Hughes and Callas 2011).
Nic · mAbs are precise probes for testing and validating the “rate hypothesis” and the antibody-mediated nicotine-PK mechanism for nicotine addiction as dose levels and affinities can be directly manipulated and controlled. Thus, nic · mAbs afford the opportunity to generate significant insight as to the nicotine affinities and nic · IgG levels required by vaccination for sufficient altering of the PK of the total body burden of nicotine and the commensurate reduction in brain nicotine levels.
The Pentel Lab has ascertained the nic · mAb dose- and affinity-response relationships needed in molecular mechanistic terms and in behavioral terms in vivo. Nic311 is a nic · mAb isolated from mice who were vaccinated with NicVAX using traditional hybridoma techniques (Keyler et al. 2005). In a separate effort (Beerli et al. 2008), B-cells isolated from a human volunteer vaccinated with NicQβ were screened and high-affinity fully human nic · mAbs were isolated and produced (Nic12). Dose-response and affinity-response relationships have been demonstrated with respect to reduction in brain nicotine levels in vivo for both Nic311 and Nic12 for attenuation of nicotine discrimination and self-administration behaviors.
Nic311 (K d = 60 nM2) was dosed from 10 to 160 mg/kg which led to an ~80 % maximal reduction in the amount of nicotine reaching the brain in rats within 3 min of nicotine IV dosing (Fig. 2.1). The dose of nicotine was calculated to be the analogous amount of nicotine absorbed by a smoker from 1 to 2 cigarettes (Benowitz 2008). Furthermore, brain nicotine levels were significantly dependent upon the affinity of the nic · mAb. Several nic · mAbs were isolated from mice vaccinated with NicVAX with nicotine Kd’s ranging from 60 to 250 nM. This series of nic · mAbs demonstrated a clear affinity-response relationship (Fig. 2.2).
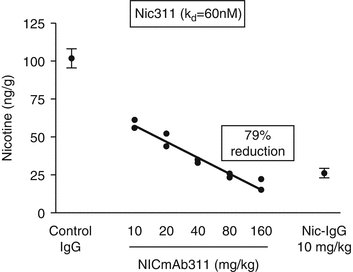
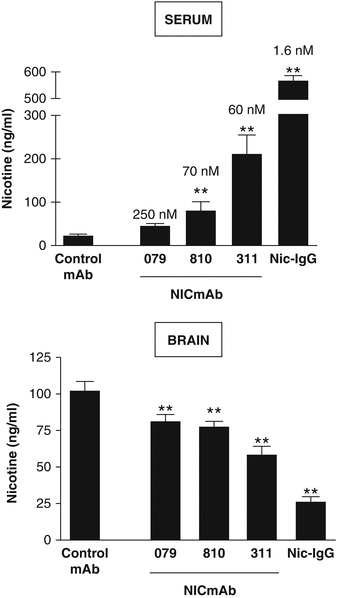
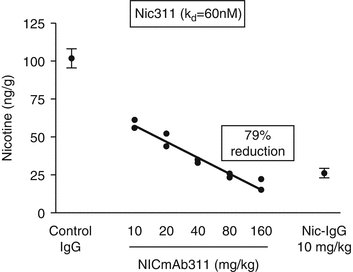
Fig. 2.1
Dose-response relationship for nicotine distribution to the brain after treatment with Nic311 compared with control-mAb in rats (N=2 per dose). Increasing Nic311 dose was associated with decreasing brain nicotine concentration (r = 0.99; p < 0.01) and increasing serum nicotine concentration (data not shown) measured 3 min after a nicotine dose (r = 0.99; p < 0.001) (adapted from Keyler et al. 2005)
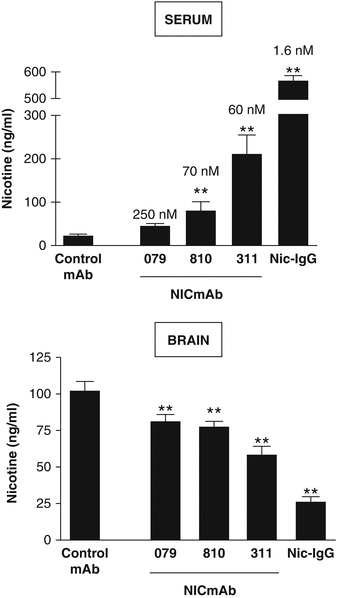
Fig. 2.2
Affinity-response of three (3) Nic · mAbs with affinities (Kd) for nicotine ranging from 60 nM to 260 nM. All three (3) of these Nic · mAbs were derived from hybridomas generated from mice vaccinated with NicVAX. Serum and brain nicotine concentrations were followed after a single nicotine dose in rats pretreated with 10 mg/kg control-mAb, monoclonal Nic · mAbs, or Nic · IgG. Nicotine (0.03 mg/kg) was administered 30 min after the pretreatment and serum and brain collected 3 min after the nicotine dose. Pretreatment with each of the three (3) Nic · mAbs or with Nic · IgG increased nicotine retention in serum and reduced nicotine distribution to brain. Decreasing Kd (values shown above columns in top panel) was associated with increasing nicotine concentration in serum (r = 0.67; p < 0.001) and decreasing nicotine concentration in brain (r = 0.69; p < 0.001). ** indicates p < 0.01 compared with control-mAb (adapted from Keyler et al. 2005)
Additionally, for the fully human nic · mAbs isolated from a single human donor (Beerli et al. 2008) in a completely separate, independent laboratory using a different nicotine vaccine (NicQβ), a dose- (Fig. 2.3) and affinity-response relationship was also observed for nic · mAbs with nicotine affinities ranging from 7 nM (Nic12) to over 744 nM. Even though the Nic12 experiments were conducted in mice as opposed to rats, albeit under similar relative nicotine doses and a similar time course to the Nic311 studies, the similarity of the dose-response is striking, with maximal reduction in brain nicotine levels of ~80 % by an ~150 mg/kg nic · mAb dose. Both studies clearly demonstrated statistically significant dose-response and affinity-response relationships in rodent models.
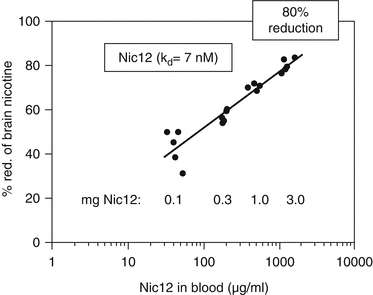
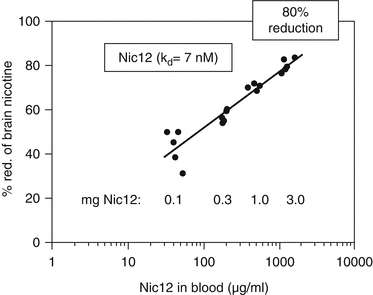
Fig. 2.3
Dose-response of inhibition of nicotine entry into brain by Nic12. Mice were injected i.p. with 0.1, 0.3, 1, or 3 mg of Nic12. One day later, mice were injected i.v. with 750 ng of tritium-labeled nicotine. Mice were sacrificed after 5 min and nicotine concentrations in brains were measured. Antibody concentrations in sera were determined and plotted against the percentage nicotine-reduction in the brain (adapted from Beerli et al. 2008). Copyright (2008) National Academy of Sciences, USA
Polyclonal nicotine-specific IgGs elicited by the Nic-Qβ vaccine (alum adjuvant) in humans have nicotine affinities in the 30–70 nM range (Maurer et al. 2005). Rabbits vaccinated with the 3’AmNic-rEPA vaccine in Freund’s adjuvant (Pentel et al. 2000) elicited nic · IgG with 1.6 nM affinities to nicotine. Interestingly, since larger vaccine doses and more potent adjuvants can be tested in the preclinical setting not suitable for clinical use, nic · IgG levels upto 500 μg/ml have been readily attained. These very high levels of nic · IgG significantly reduced nicotine levels entering the brain of rodents and attenuated associated nicotine behaviors (Maurer et al. 2005; Keyler et al. 2008).
The 1.6 nM, 10 mg/kg dose of nicotine-specific rabbit IgG lowered brain nicotine levels by an additional 50 % of those attained by the 60 nM Nic311 nic · mAb (10 mg/kg, Fig. 2.1). In theory, a nicotine vaccine which can elicit antibodies with nicotine affinities in the single digit nanomolar range may reduce brain nicotine levels to >90 % compared to controls. Next-generation vaccines will likely benefit from technological improvements (e.g., adjuvants, carriers, haptens/linkers, increased hapten densities, nanoparticles, delivery, etc.) aimed at improving nicotine affinity – possibly even to the sub-nanomolar level, leading to a significant further reduction in brain nicotine levels.
In preclinical studies of the combination of vaccination augmented with nic-mAbs, a functional dose-response relationship was extended to nicotine-specific antibody levels that were higher than either approach was able to attain alone. Figure 2.4 depicts this additive effect where (i) vaccination of rats yielded 166 μg/ml concentration of nic · IgG, (ii) 80 mg/kg dosing of Nic311 yielded a 172 μg/ml levels of nic · mAb, and (iii) combined active and passive immunization yielded nicotine-specific antibody levels of 420 μg/ml nic · IgG and Nic · mAb. The group receiving both nicotine vaccine and nic · mAb demonstrated brain nicotine levels >95 % less than controls. (Roiko et al. 2008).
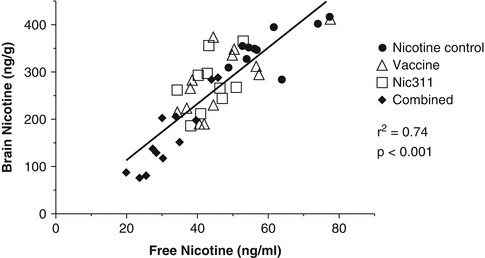
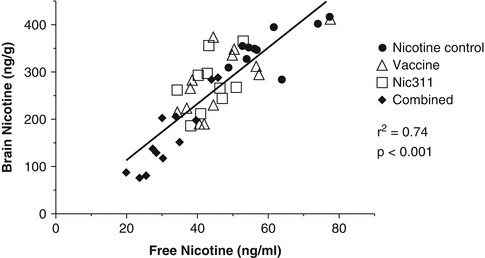
Fig. 2.4
Relationship between free nicotine concentration in serum and the brain nicotine concentrations for rats receiving 0.03 mg/kg doses of nicotine following a four injection regimen of NicVAX and 80 mg/kg nic311 mAb doses according to the locomotor sensitization protocol as described in Roiko et al. 2008. Animals were sacrificed 50 minutes after the final nicotine dose was administered
2.5 Dose-Response Relationships Observed in NicVAX Phase III Studies
In 2012, a patent application from Nabi Biopharmaceuticals was published (WO 2012/07477, PCT/US2011/061229) (Fahim and Kalnik 2012) that contains post hoc exploratory analysis for hypothesis generation of a putative threshold of nic · IgG needed to support long-term abstinence from smoking in an effort to guide future development of nicotine vaccines. Table 2.2 describes the association of nic · IgG in the top 1/3 (33 %) of vaccine responders measured by area under the curve (AUC) with abstinence. Even though non-stratified ITT results were negative in the NicVAX phase III studies (Abstinence rates to one-year: NicVAX = 11 % and placebo = 11 %), a secondary analysis using pre-specified nic · IgG levels defined in the statistical analysis plan to stratify the phase III intent-to-treat (ITT) population and a statistically significant correlation with long-term abstinence was observed (Fahim et al. 2013). Thus, as these stratification analyses were pre-specified prior to unblinding of the phase III results, the observation that high nic · IgG levels support long-term abstinence in the NicVAX phase IIb study (NCT00318383) prospectively confirms the correlation of high nic · IgG with long-term abstinence to 1 year seen in the phase II study.
Table 2.2
Long-term abstinence stratified by post-vaccine Ab level: NicVAX PhIII
Subject group | 12-month, 36-week CARa (weeks 17–52) |
---|---|
High Ab (top 33 % by AUC) | 13.0 % (n = 22/169) |
Low Ab (lower 67 %) | 5.6 % (n = 19/337) |
Placebo | 6.3 % (n = 32/510) |
Difference (high Ab vs. low Ab) | 7.4 % (p = 0.005) |
Difference (high Ab vs. placebo) | 6.7 % (p = 0.006) |
Difference (low Ab vs. placebo) | −0.7 % (not significant) |
In the post hoc analyses presented in Table 2.2, the treatment effect of the top 33 % nic · IgG responders by AUC compared to placebo was 6.7 % for 36 weeks of continuous abstinence (CAR weeks 17–52, secondary endpoint). This modest rate of efficacy in the high nic · IgG responder group suggests that other factors beyond inadequate nic · IgG levels may have contributed to the failure of the NicVAX phase III studies (e.g., highly variable nicotine affinities). Unfortunately, one of the main attributes not characterized were the nicotine affinities of nic · IgG due to the rapid wind-down of Nabi Biopharmaceuticals as a result of the phase III failures. Eliciting high-affinity nic · IgG is currently viewed as one of the most important aspects driving functional efficacy and is being actively targeted in the development of second generation of nicotine conjugate vaccines (McCluskie et al. 2013a, b).
Table 2.3 describes a post hoc, pooled, completer analysis of both NicVAX phase III studies (NCT00836199 and NCT01102114) hypothesis generation of the putative threshold level of nic · IgG needed to support long-term abstinence. In a similar post hoc analysis of the NicVAX phase IIb data (unpublished), it was theorized that the nic · IgG level present at the target quit date (TQD) and maintained for the ensuing 4 months would provide insight into the minimum prolonged nic · IgG levels needed to support long-term abstinence. Thus, the minimum threshold level of nic · IgG over weeks 14–30 of the study (TQD through one month following the final injection at 6 months; Cmin14–30 See Figure 2.5) was used to stratify the ITT population in increments of 25 μg/ml. Notably, this minimum threshold analysis revealed a statistically significant dose-response relationship of nic · IgG levels with long-term abstinence. The statistical analyses were an uncorrected Fisher’s exact test for multiple comparisons as this particular stratification analysis had uncovered an association in the phase IIb study. The highest Cmin14–30 stratification group with a meaningful group size (N ≥ 30) had a threshold of ≥125 μg/ml nic · IgG, yielding a long-term abstinence rate of 23.3 % for the completer population in this meta-analysis of both studies. Assuming a 25 % dropout rate, a 17.5 % abstinence rate was achieved for this top ~5 % of IgG responders.
Table 2.3
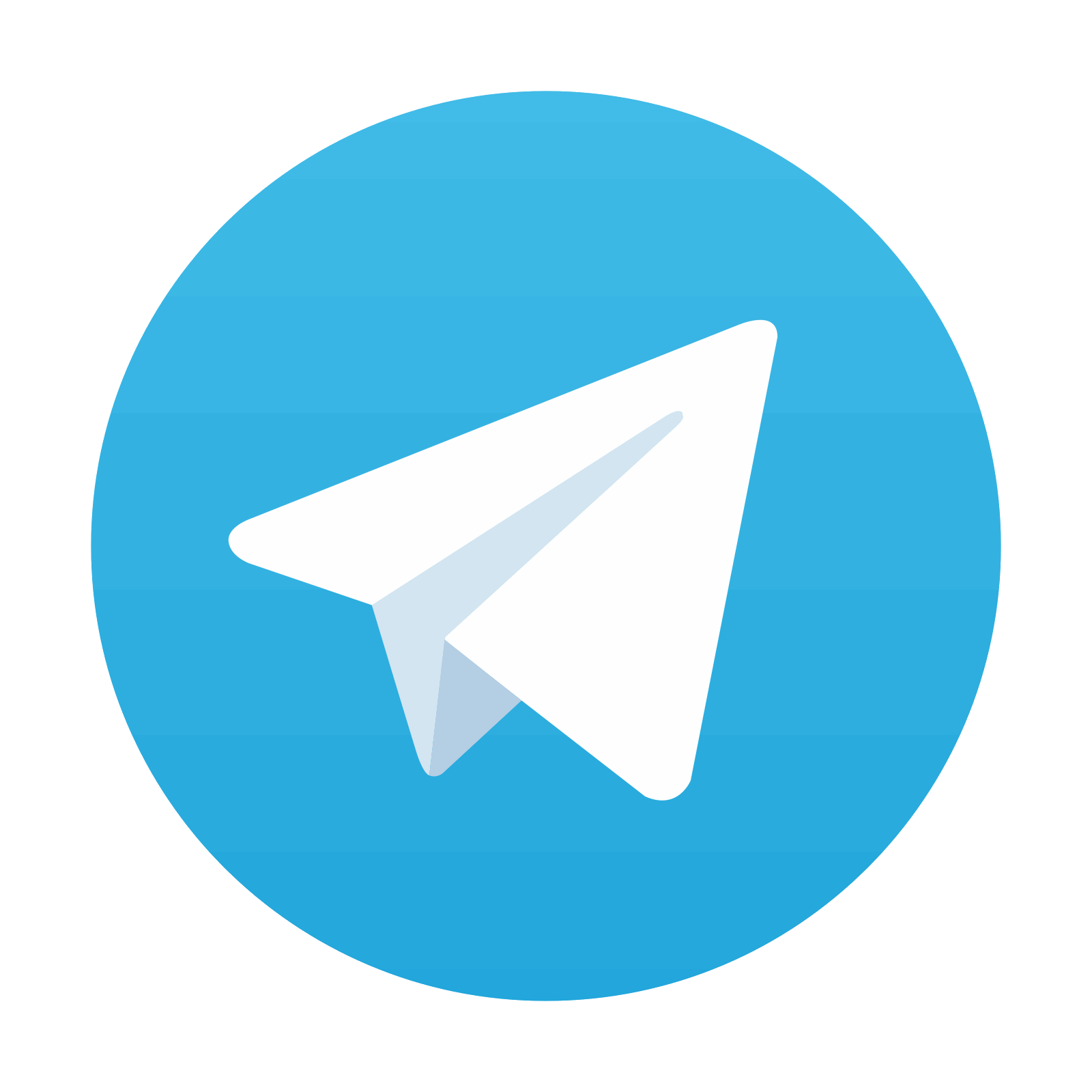
Antibody dose-response correlation analysis: hypothetical threshold levels
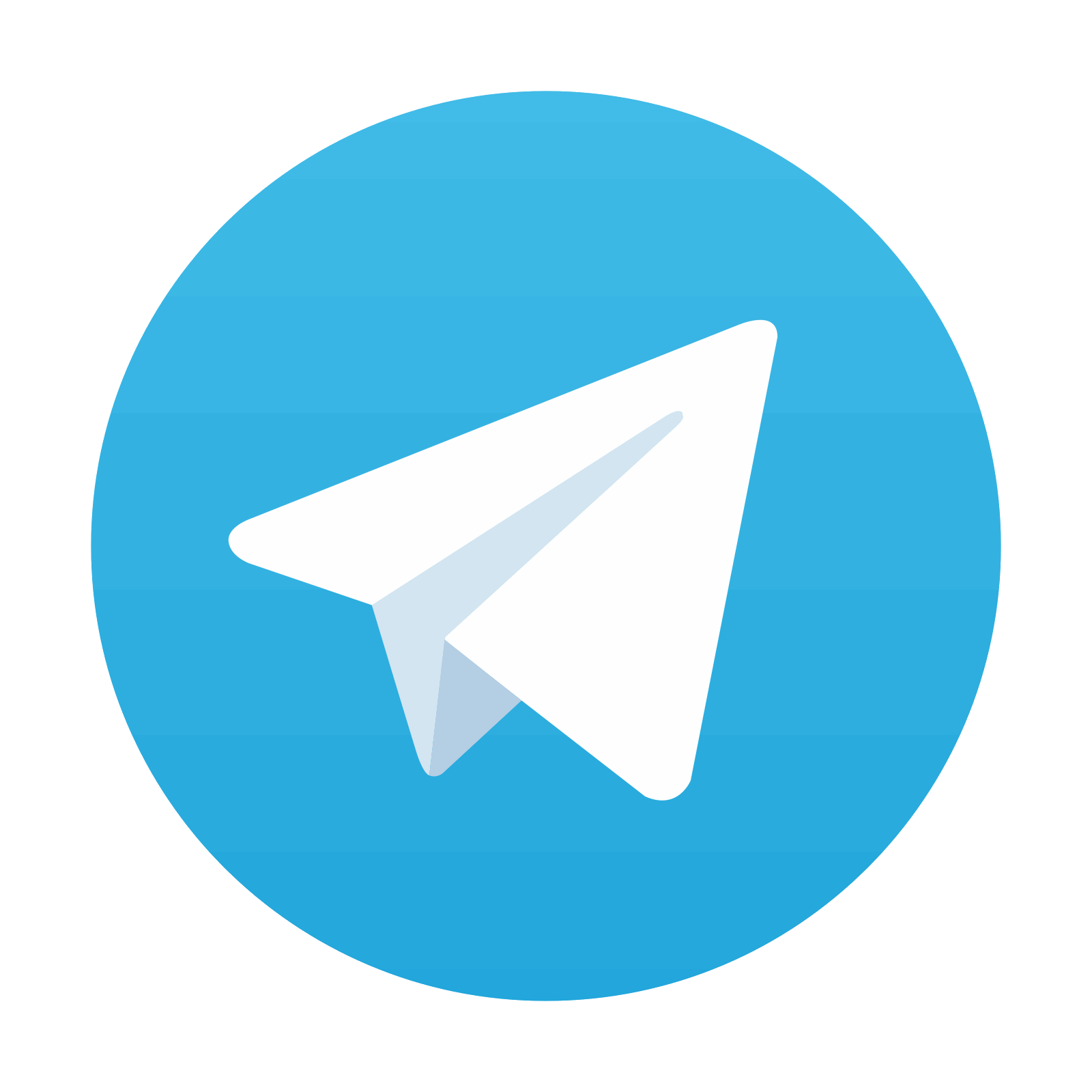
Stay updated, free articles. Join our Telegram channel

Full access? Get Clinical Tree
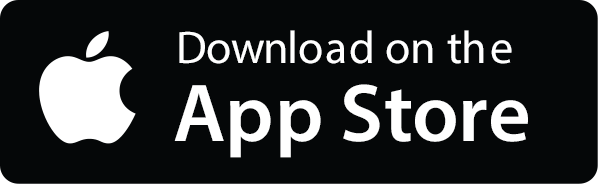
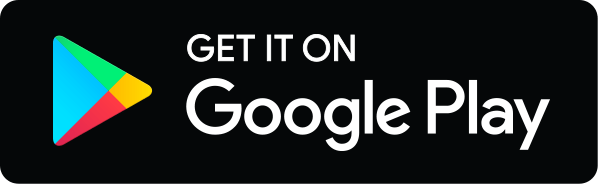
