Chapter 9 The thyroid gland
Introduction
The thyroid gland secretes three hormones: thyroxine (T4) and triiodothyronine (T3), both of which are iodinated derivatives of tyrosine (Fig. 9.1), and calcitonin, a polypeptide hormone. T4 and T3 are produced by the follicular cells but calcitonin is secreted by the C cells, which are of separate embryological origin. Calcitonin is functionally unrelated to the other thyroid hormones. It has a minor role in calcium homoeostasis and disorders of its secretion are rare (see Chapter 12). Thyroid disorders in which there is either over- or under-secretion of T4 and T3 are, however, common.

Figure 9.1 Chemical structure of the thyroid hormones, T4 and T3, and the inactive metabolite of T4, rT3.
Thyroxine synthesis and release are stimulated by the pituitary trophic hormone, thyroid-stimulating hormone (TSH). The secretion of TSH is controlled by negative feedback by the thyroid hormones (see p. 119), which modulate the response of the pituitary to the hypothalamic hormone, thyrotrophin-releasing hormone (TRH; Fig. 9.2). This feedback is mediated primarily by T3 produced by the action of iodothyronine deiodinase on T4 in the thyrotroph cells of the anterior pituitary. Glucocorticoids, dopamine and somatostatin inhibit TSH secretion. The physiological significance of this is not known, but it may be relevant to the disturbances of thyroid hormones that can occur in non-thyroidal illness (see p. 160). The feedback mechanisms result in the maintenance of steady plasma concentrations of thyroid hormones.
The major product of the thyroid gland is T4. Ten times less T3 is produced (the proportion may be greater in thyroid disease), most (approximately 80%) T3 being derived from T4 by deiodination in peripheral tissues, particularly the liver, kidneys and muscle, catalysed by selenium-containing iodothyronine deiodinases. T3 is 3–4 times more potent than T4. In tissues, most of the effect of T4 results from this conversion to T3, so that T4 itself is essentially a prohormone. Deiodination can also produce reverse triiodothyronine (rT3; see Fig. 9.1), which is physiologically inactive. It is produced instead of T3 in starvation and many non-thyroidal illnesses, and the formation of either the active or inactive metabolite of T4 appears to play an important part in the control of energy metabolism.
Thyroid hormones
Synthesis
Thyroid hormone synthesis involves a number of specific enzyme-catalysed reactions, beginning with the uptake of iodide by the gland and culminating in the iodination of tyrosine residues in the protein thyroglobulin (Fig. 9.3); these reactions are all stimulated by TSH. Rare, congenital forms of hypothyroidism caused by inherited deficiencies of each of the various enzymes concerned have been described.
Thyroglobulin is stored within the thyroid gland in colloid follicles. These are accumulations of thyroglobulin-containing colloid surrounded by thyroid follicular cells. Release of thyroid hormones (stimulated by TSH) involves pinocytosis of colloid by follicular cells, fusion with lysosomes to form phagocytic vacuoles, and proteolysis (Fig. 9.4). Thyroid hormones are thence released into the bloodstream. Proteolysis also results in the liberation of mono- and diiodotyrosines (MIT and DIT); these are usually degraded within thyroid follicular cells and their iodine is retained and re-utilized. A small amount of thyroglobulin also reaches the bloodstream.
Thyroid hormones in blood
The normal plasma concentrations of T4 and T3 are 60–150 nmol/L and 1.0–2.9 nmol/L, respectively. Both hormones are extensively protein bound: some 99.98% of T4 and 99.66% of T3 are bound, principally to a specific thyroxine-binding globulin (TBG) and, to a lesser extent, to prealbumin and albumin. TBG is approximately one-third saturated at normal concentrations of thyroid hormones (Fig. 9.5). It is generally accepted that only the free, non-protein-bound, thyroid hormones are physiologically active. Although the total T4 concentration is normally 50 times that of T3, the different extents to which these hormones are bound to protein mean that the free T4 concentration is only 2–3 times that of free T3 (typical reference ranges are 9–26 pmol/L for free T4 and 3.0–9.0 pmol/L for free T3).
Total (free + bound) thyroid hormone concentrations in plasma are dependent not only on thyroid function but also on the concentrations of binding proteins. If these were to increase (Fig. 9.6), the temporary fall in free hormone concentration caused by increased protein binding would stimulate TSH release and this would restore the free hormone concentrations to normal: if binding protein concentrations were to fall, the reverse would occur. In either situation, there would be a change in the concentrations of total hormones, but the free hormone concentrations would remain normal.
This is a matter of considerable practical importance, as changes in the concentrations of the binding proteins occur in many circumstances (Fig. 9.7), causing changes in total hormone concentrations but not necessarily in those of the free (physiologically available) hormones. Furthermore, certain drugs, for example salicylates and phenytoin, displace thyroid hormones from their binding proteins, thus reducing total, but not free, hormone concentrations once a new steady state is attained. If an attempt is made to assess thyroid status in a patient who is not in a steady state, the results may be bizarre and misleading.
Tests of thyroid function
Thyroid-stimulating hormone
Typical results of thyroid function tests in various conditions are shown in Figure 9.8.
Thyrotrophin-releasing hormone test
In this test, plasma TSH is measured immediately before, and 20 and 60 min after, giving the patient 200 µg of TRH i.v. (Fig. 9.9). The normal response is an increase in TSH concentration of 2–20 mU/L in 20 min, with reversion towards the basal value at 60 min.
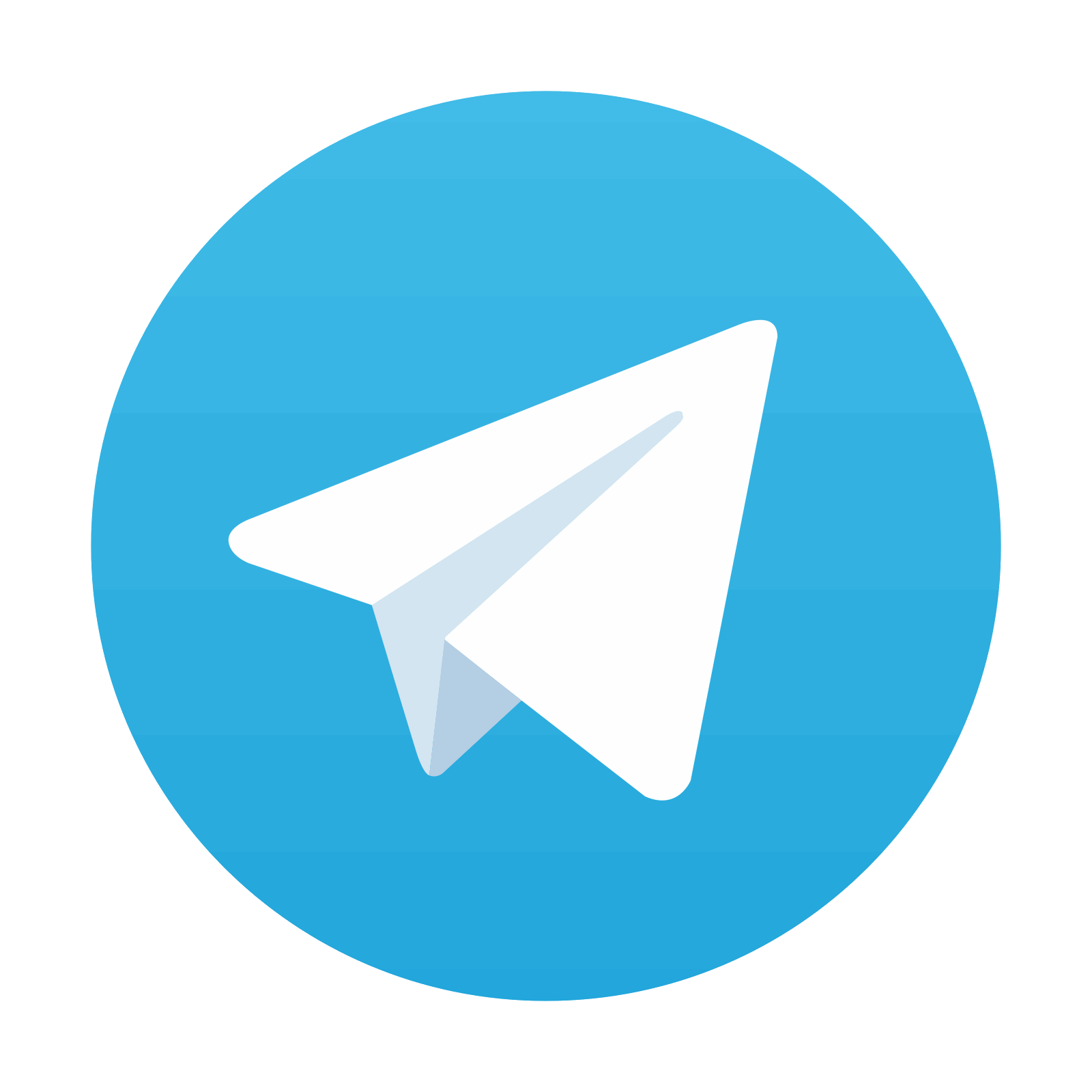
Stay updated, free articles. Join our Telegram channel

Full access? Get Clinical Tree
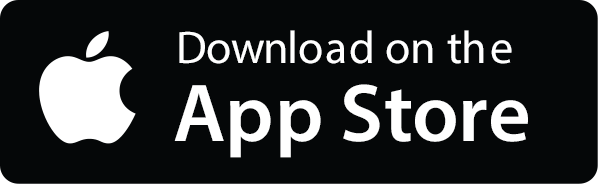
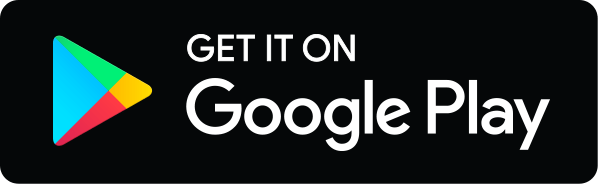