INTRODUCTION
Microbiology is the study of microorganisms, a large and diverse group of microscopic organisms that exist as single cells or cell clusters; it also includes viruses, which are microscopic but not cellular. Microorganisms have a tremendous impact on all life and the physical and chemical makeup of our planet. They are responsible for cycling the chemical elements essential for life, including carbon, nitrogen, sulfur, hydrogen, and oxygen; more photosynthesis is carried out by microorganisms than by green plants. Furthermore, there are 100 million times as many bacteria in the oceans (13 × 1028) as there are stars in the known universe. The rate of viral infections in the oceans is about 1 × 1023 infections per second, and these infections remove 20–40% of all bacterial cells each day. It has been estimated that 5 × 1030 microbial cells exist on earth; excluding cellulose, these cells constitute about 90% of the biomass of the entire biosphere. Humans also have an intimate relationship with microorganisms; more than 90% of the cells in our bodies are microbes. The bacteria present in the average human gut weigh about 1 kg, and a human adult will excrete his or her own weight in fecal bacteria each year. The number of genes contained within this gut flora outnumber that contained within our genome 150-fold, and even in our own genome, 8% of the DNA is derived from remnants of viral genomes.
BIOLOGIC PRINCIPLES ILLUSTRATED BY MICROBIOLOGY
Nowhere is biologic diversity demonstrated more dramatically than by microorganisms, creatures that are not directly visible to the unaided eye. In form and function, be it biochemical property or genetic mechanism, analysis of microorganisms takes us to the limits of biologic understanding. Thus, the need for originality—one test of the merit of a scientific hypothesis—can be fully met in microbiology. A useful hypothesis should provide a basis for generalization, and microbial diversity provides an arena in which this challenge is ever present.
Prediction, the practical outgrowth of science, is a product created by a blend of technique and theory. Biochemistry, molecular biology, and genetics provide the tools required for analysis of microorganisms. Microbiology, in turn, extends the horizons of these scientific disciplines. A biologist might describe such an exchange as mutualism, that is, one that benefits all of the contributing parties. Lichens are an example of microbial mutualism. Lichens consist of a fungus and phototropic partner, either an alga (a eukaryote) or a cyanobacterium (a prokaryote) (Figure 1-1). The phototropic component is the primary producer, and the fungus provides the phototroph with an anchor and protection from the elements. In biology, mutualism is called symbiosis, a continuing association of different organisms. If the exchange operates primarily to the benefit of one party, the association is described as parasitism, a relationship in which a host provides the primary benefit to the parasite. Isolation and characterization of a parasite—such as a pathogenic bacterium or virus—often require effective mimicry in the laboratory of the growth environment provided by host cells. This demand sometimes represents a major challenge to investigators.
FIGURE 1-1
Diagram of a lichen, consisting of cells of a phototroph, either an alga or a cyanobacterium, entwined within the hyphae of the fungal partner. (Reproduced with permission from Nester EW, Anderson DG, Roberts CE, Nester MT (editors): Microbiology: A Human Perspective, 6th ed. McGraw-Hill, 2009, p. 293.)
The terms mutualism, symbiosis, and parasitism relate to the science of ecology, and the principles of environmental biology are implicit in microbiology. Microorganisms are the products of evolution, the biologic consequence of natural selection operating on a vast array of genetically diverse organisms. It is useful to keep the complexity of natural history in mind before generalizing about microorganisms, the most heterogeneous subset of all living creatures.
A major biologic division separates the eukaryotes, organisms containing a membrane-bound nucleus, from prokaryotes, organisms in which DNA is not physically separated from the cytoplasm. As described in this chapter and in Chapter 2, further major distinctions can be made between eukaryotes and prokaryotes. Eukaryotes, for example, are distinguished by their relatively large size and by the presence of specialized membrane-bound organelles such as mitochondria.
As described more fully later in this chapter, eukaryotic microorganisms—or, phylogenetically speaking, the Eukarya—are unified by their distinct cell structure and phylogenetic history. Among the groups of eukaryotic microorganisms are the algae, the protozoa, the fungi, and the slime molds.
VIRUSES
The unique properties of viruses set them apart from living creatures. Viruses lack many of the attributes of cells, including the ability to replicate. Only when it infects a cell does a virus acquire the key attribute of a living system—reproduction. Viruses are known to infect all cells, including microbial cells. Recently, viruses called virophages have been discovered that infect other viruses. Host–virus interactions tend to be highly specific, and the biologic range of viruses mirrors the diversity of potential host cells. Further diversity of viruses is exhibited by their broad array of strategies for replication and survival.
Viral particles are generally small (eg, adenovirus is 90 nm) and consist of a nucleic acid molecule, either DNA or RNA, enclosed in a protein coat, or capsid (sometimes itself enclosed by an envelope of lipids, proteins, and carbohydrates). Proteins—frequently glycoproteins—in the capsid determine the specificity of interaction of a virus with its host cell. The capsid protects the nucleic acid and facilitates attachment and penetration of the host cell by the virus. Inside the cell, viral nucleic acid redirects the host’s enzymatic machinery to functions associated with replication of the virus. In some cases, genetic information from the virus can be incorporated as DNA into a host chromosome. In other instances, the viral genetic information can serve as a basis for cellular manufacture and release of copies of the virus. This process calls for replication of the viral nucleic acid and production of specific viral proteins. Maturation consists of assembling newly synthesized nucleic acid and protein subunits into mature viral particles, which are then liberated into the extracellular environment. Some very small viruses require the assistance of another virus in the host cell for their duplication. The delta agent, also known as hepatitis D virus, is too small to code for even a single capsid protein and needs help from hepatitis B virus for transmission. Viruses are known to infect a wide variety of plant and animal hosts as well as protists, fungi, and bacteria. However, most viruses are able to infect specific types of cells of only one host species.
Some viruses are large and complex. For example, Mimivirus, a DNA virus infecting Acanthamoeba, a free-living soil ameba, has a diameter of 400–500 nm and a genome that encodes 979 proteins, including the first four aminoacyl tRNA synthetases ever found outside of cellular organisms and enzymes for polysaccharide biosynthesis. An even larger marine virus has recently been discovered (Megavirus); its genome (1,259,197-bp) encodes 1120 putative proteins and is larger than that of some bacteria (see Table 7-1). Because of their large size, these viruses resemble bacteria when observed in stained preparations by light microscopy; however, they do not undergo cell division or contain ribosomes.
A number of transmissible plant diseases are caused by viroids—small, single-stranded, covalently closed circular RNA molecules existing as highly base-paired rodlike structures. They range in size from 246 to 375 nucleotides in length. The extracellular form of the viroid is naked RNA—there is no capsid of any kind. The RNA molecule contains no protein-encoding genes, and the viroid is therefore totally dependent on host functions for its replication. Viroid RNA is replicated by the DNA-dependent RNA polymerase of the plant host; preemption of this enzyme may contribute to viroid pathogenicity.
The RNAs of viroids have been shown to contain inverted repeated base sequences at their 3′ and 5′ ends, a characteristic of transposable elements (see Chapter 7) and retroviruses. Thus, it is likely that they have evolved from transposable elements or retroviruses by the deletion of internal sequences.
The general properties of animal viruses pathogenic for humans are described in Chapter 29. Bacterial viruses are described in Chapter 7.
PRIONS
A number of remarkable discoveries in the past three decades have led to the molecular and genetic characterization of the transmissible agent causing scrapie, a degenerative central nervous system disease of sheep. Studies have identified a scrapie-specific protein in preparations from scrapie-infected brains of sheep that is capable of reproducing the symptoms of scrapie in previously uninfected sheep (Figure 1-2). Attempts to identify additional components, such as nucleic acid, have been unsuccessful. To distinguish this agent from viruses and viroids, the term prion was introduced to emphasize its proteinaceous and infectious nature. The cellular form of the prion protein (PrPc) is encoded by the host’s chromosomal DNA. PrPc is a sialoglycoprotein with a molecular mass of 33,000–35,000 Da and a high content of α-helical secondary structure that is sensitive to proteases and soluble in detergent. PrPc is expressed on the surface of neurons via a glycosylphosphatidyl inositol anchor in both infected and uninfected brains. A conformational change occurs in the prion protein, changing it from its normal or cellular form PrPc to the disease-causing conformation, PrPSc (Figure 1-3). When PrPSc is present in an individual (owing to spontaneous conformational conversion or to infection), it is capable of recruiting PrPc and converting it to the disease form. Thus, prions replicate using the PrPc substrate that is present in the host.
There are additional prion diseases of importance (Table 1-1 and see Chapter 42). Kuru, Creutzfeldt-Jakob disease (CJD), Gerstmann-Sträussler-Scheinker disease, and fatal familial insomnia affect humans. Bovine spongiform encephalopathy, which is thought to result from the ingestion of feeds and bone meal prepared from rendered sheep offal, has been responsible for the deaths of more than 184,000 cattle in Great Britain since its discovery in 1985. A new variant of CJD (vCJD) has been associated with human ingestion of prion-infected beef in the United Kingdom and France. A common feature of all of these diseases is the conversion of a host-encoded sialoglycoprotein to a protease-resistant form as a consequence of infection.
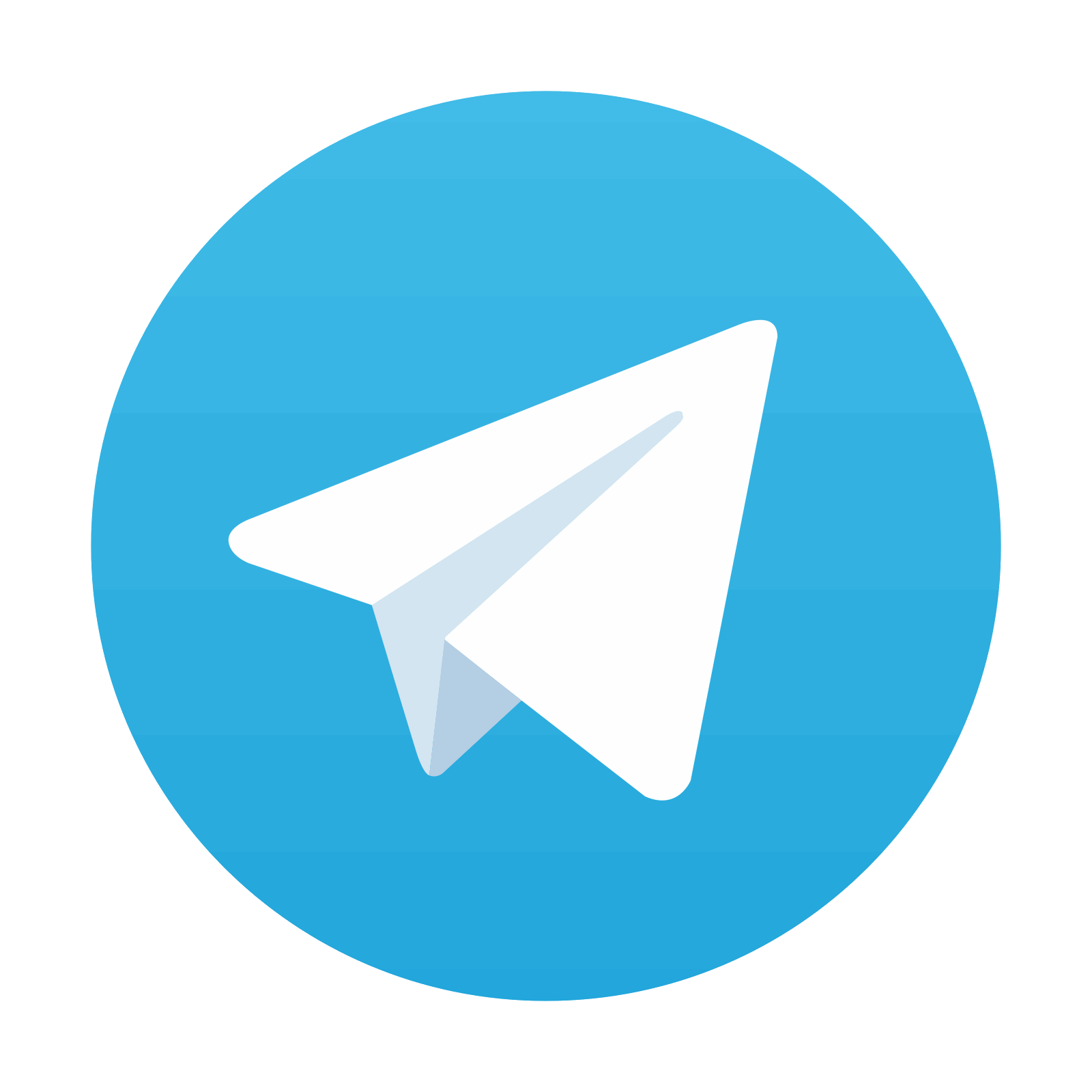
Stay updated, free articles. Join our Telegram channel

Full access? Get Clinical Tree
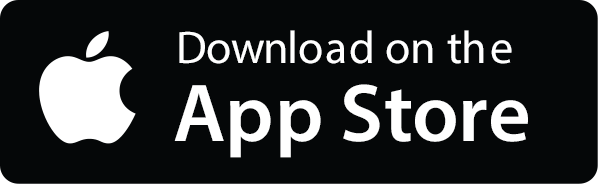
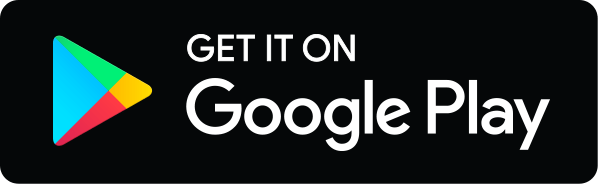