Fig. 1.1
Schematic diagram of tissue architecture with regard to the spatial distribution of cellular and extracellular components
In other words, in order to expand in size beyond the diffusion limit of oxygen in tissue, a new vasculature must form in and around the tumor. The ingrowth of this vasculature is fueled by soluble and matrix-bound growth factors and enzymes produced and secreted by both the tumor cells and the stromal cells comprising the microenvironment (Fig. 1.2) [6]. Strong evidence exists indicating that the stromal cells play as central a role in matrix remodeling, invasion, and metastasis as the tumor cells themselves [7–9]. Critical to this process is the observation that carcinoma cells are manipulated and co-opt the surrounding stromal cells to enhance tumor growth [10]. Specifically, tumor-stromal paracrine signaling pathways have been demonstrated to play a major role in the tumorigenesis and subsequent outgrowth of tumors in multiple sites [11–13]. For example, stromal fibroblasts from prostate tumors are able to stimulate tumor formation of immortal but non-transformed prostate epithelial cells when the mixture is injected orthotopically into nude mice [10].


Fig. 1.2
Schematic diagram of the interaction between the tumor and microenvironment as mediated by tumor-secreted factors paracrine-acting factors that modulate angiogenesis
As stated above, tumor angiogenesis is intricately linked to signaling between the tumor and microenvironment. In normal tissue architecture, the epithelial compartment is not vascularized as it is generally only a few cell layers in thickness, thus allowing oxygen to diffuse across the basement membrane and nourish both layers. However, when squamous tumors grow in an uncontrolled manner or glandular tumors form and the lumen of epithelial-lined ducts fills with tumor cells, the cells in the center of the mass eventually become hypoxic due to the distance from existing blood vessels [14]. Thus, in order for carcinoma cells to gain access to blood vessels, the basement membrane must be degraded, allowing the blood vessels to grow into the epithelial compartment. In order for this to happen, tumor cells must secrete pro-angiogenic growth factors, turn off or reduce production of anti-angiogenic factors, and recapitulate this process in the microenvironment by secreting paracrine signals that act on the stromal cells to induce a pro-angiogenic environment. Though a myriad of pro- and anti-angiogenic factors have been discovered and studied, the initial effort in understanding their regulation was examined in a cell autonomous fashion, with most of the attention paid to vascular endothelial growth factor (VEGF) [15–19] and thrombospondin-1 (Tsp-1) [20, 21], two of the major positive and negative regulators of angiogenesis. However, as the importance of the tumor microenvironment became more apparent, the study of the regulation of angiogenic factors in stromal cells also increased.
Not only is the regulation of angiogenesis in the tumor microenvironment critical to primary tumor growth but also for metastatic dissemination and growth in distant organs. It is well established that tumors arising in different sites preferentially metastasize to specific organs [22]. For example, prostate cancer metastasizes preferentially to the bone and liver, while breast cancer metastasizes to the brain, bone, and lung [23]. The ability of a tumor cell to survive and proliferate in a metastatic environment ultimately relies on its ability to augment the angiogenic output of its microenvironment.
The tumor microenvironment can grossly be categorized into two types of cells: (1) resident tissue cells that are present prior to tumor development and (2) infiltrating cells that are recruited to the tumor from the circulation or bone marrow. The former is mainly comprised of fibroblasts and endothelial cells, while the latter is comprised of immune/inflammatory cells, which include B and T cells, neutrophils, mast cells, dendritic cells, and macrophages. In this chapter we will explore the roles of these different cell types, as well as the growth factors and extracellular matrix proteins that contribute to tumor progression.
Cell Signaling Mechanisms and Factors Influencing Stromal Angiogenesis
In order to understand how resident and infiltrating cells contribute to tumor angiogenesis , it is first necessary to delineate and describe the major angiogenic factors that stimulate and inhibit vessel ingrowth.
VEGF
Vascular endothelial growth factor (VEGF) stimulates endothelial cell migration and proliferation. It was first identified via its ability to increase vascular permeability and thus was initially called vascular permeability factor or VPF [24, 25]. The regulation of VEGF in tumor cells has been exhaustively studied. Signal transduction pathways leading from receptor tyrosine kinases or oncogenic Ras and PI3 kinase via the MAPK or Akt pathway lead to increased transcription of VEGF and its subsequent secretion into the extracellular matrix [17]. However, carcinoma cells also secrete proteins into the extracellular space, which do not act directly on endothelial cells but rather modulate VEGF production and secretion by stromal cells in the microenvironment, such as TGF-β, PDGF, and bFGF [26, 27].
Stromal VEGF expression was first demonstrated to be regulated by carcinoma cells in a transgenic mouse model in which GFP, driven by the VEGF promoter, was inserted into the mouse genome [28]. In this model, activation of the VEGF promoter results in the expression of GFP. Examination of tumor xenografts in these VEGF-GFP mice revealed, via fluorescence microscopy, that stromal fibroblasts had infiltrated the tumor fluoresced green, indicating that the VEGF promoter had been activated. Strikingly, in normal tissues there were no fluorescent cells, indicating that VEGF expression is not required for normal tissue homeostasis. These results indicated that tumors secrete factors that act on cells in the microenvironment to stimulate VEGF expression.
However, while it was clear from these experiments that VEGF expression was being stimulated, it was not evident whether this stimulation was required for tumor growth, supportive of tumor growth, or merely a physiological reaction to local tumor growth. The evidence that stromally produced VEGF was critical for tumor growth was obtained from studies designed to test the efficacy of a human specific anti-VEGF antibody, bevacizumab (Avastin). In these experiments, human tumor cells were injected into immunocompromised mice, which were subsequently treated with the human-specific VEGF antibody [29]. While the antibody was able to dramatically inhibit tumor growth, the tumors still grew. The authors of the study hypothesized that the continued growth of the treated tumors was due to a residual angiogenic stimulus driven by VEGF produced and secreted from the murine tumor microenvironment, which could not be inhibited by the human-specific antibody. To test this hypothesis, human tumor xenografts were treated with human-specific VEGF antibodies as well as a soluble version of the murine VEGFR1 (mFlt) fused to IgG, which acts as a decoy receptor for VEGF [30]. This combination treatment resulted in the complete blockage of tumor growth, demonstrating the importance of the contribution stromal-produced VEGF.
Basic Fibroblast Growth Factor (bFGF)
Basic fibroblast growth factor (bFGF, FGF2) is another potent pro-angiogenic growth factor [31–33]. One interesting oddity about bFGF is despite the presence of high-affinity cell surface receptors [34] and the myriad of observations that bFGF stimulates endothelial cell proliferation and angiogenesis in vivo and in vitro, the protein lacks a signal sequence to direct its secretion [35, 36]. The paracrine regulation of bFGF in stromal cells and subsequent effect on tumor angiogenesis has been confounded by its ability to potently stimulate tumor cell proliferation through FGFR signaling via both autocrine and paracrine signaling [37–39]. Nevertheless, bFGF expression in the stroma of lung adenocarcinoma patients inversely correlates with disease progression and overall survival [40]. Additionally, bFGF production is stimulated by stem cell factor (SCF) and TGF-β in inflammatory cells, including macrophages, mast cells, and neutrophils [41]. The role of these cells in tumor angiogenesis will be detailed later in this chapter.
TGF-β
One growth factor with perhaps the most paradoxical role in tumor growth and angiogenesis is TGF-β. While TGF-β has potent pro-angiogenic activity in vivo [42], its in vitro effects on endothelial cells are in diametric opposition as it is actually growth inhibitory in this context [43, 44]. These seemingly incongruous activities were resolved by the discovery that TGF-β stimulates the expression of VEGF in stromal fibroblasts, indicating that the pro-angiogenic effects of TGF-β were mediated by the induction of VEGF in the tumor microenvironment [26, 45]. Additionally, TFG-β also dramatically stimulates the expression of bFGF in fibroblasts [46]. These results suggest that low levels of tumor-secreted TGF-β stimulate tumor-associated fibroblasts to express VEGF and bFGF thereby stimulating angiogenesis. Conversely, higher levels of TGF-β may act directly on endothelial cells inhibiting their proliferation and thus having an anti-angiogenic effect.
Adding to the paradox of TGF-β’s role in tumor angiogenesis is its ability to stimulate the expression of the anti-angiogenic protein Tsp-1 (which will be discussed in detail later in the chapter) and which then activates TGF-β from its latent form [47–51]. TGF-β is activated by two discrete processes: via proteases that cleave the latency-associated peptide and via undergoing a conformational change that exposes the receptor-binding region. Tsp-1 activates TGF-β via the latter mechanism. Moreover, TGF-β expression in fibroblasts is induced by hypoxia, which is most often a result of a lack of tumor vascularization [52].
PDGF
Another growth factor that possesses both pro- or anti-angiogenic characteristics is PDGF. In 1991, Goldsmith et al. demonstrated that PDGF was able to potently stimulate bFGF in lung fibroblasts [46]. Additionally, in response to the results achieved with the human-specific VEGF antibody described above, it was demonstrated that stromal VEGF expression was stimulated by tumor-derived PDGF [53]. In that context, inhibition of PDGF activity via a soluble version of PDGFR was able to block the stimulation of VEGF in the microenvironment and inhibit angiogenesis. Moreover, another member of the PDGF family, PDGF B, is also able to upregulate VEGF expression in vascular smooth muscle cells [26]. These data indicated that tumor-derived PDGF is a potent inducer of VEGF expression in the microenvironment.
The most logical conclusion to be drawn from the above study is that PDGF promotes angiogenesis via induction of stromal VEGF. However, somewhat analogous to TGF-β, the activities of PDGF are not as straightforward as these results would indicate. In addition to stimulating VEGF and bFGF, PDGF also stimulates Tsp-1 expression [54]. PDGF stimulation of Tsp-1 in fibroblasts is mediated by the Raf-MAPK pathway in a manner analogous to the stimulation of Tsp-1 by serum [55]. Intriguingly, the PDGF-mediated stimulation of VEGF is also mediated by the Raf-MAPK pathway [56]. Thus, whether PDGF acts as an anti-angiogenic factor or a pro-angiogenic factor is most likely dependent on orthogonal signals that act to inhibit or stimulate VEGF or Tsp-1.
Hormones and Nuclear Receptors
The studies described above indicate that two of the most potent inducers of stromal VEGF and, consequently, angiogenesis also possess the seemingly counterproductive ability to stimulate Tsp-1. These divergent events downstream from TGF-β and PDGF ligation to their receptors indicate that tumor-derived TGF-β and PDGF expression should have no net effect on angiogenesis. That being said, it has also been demonstrated that inhibition of PDGF activity inhibits tumor angiogenesis [53]. Also, as described above, despite its ability to stimulate Tsp-1, TGF-β is a potent stimulator of angiogenesis. One potential explanation for the observed pro-angiogenic activities of these two proteins is that the expression of Tsp-1 in the microenvironment is suppressed by an independent signaling mechanism. This suppression of Tsp-1 would result in the stimulation of only the pro-angiogenic factors VEGF and bFGF by these two growth factors and thus resolve the seemingly paradoxical observations.
Two candidates for such a Tsp-1-repressing factor are the hormones estrogen and androgen, which have both been demonstrated to repress Tsp-1 expression [57, 58]. While these hormones both repress Tsp-1 expression, the mechanisms utilized are different. Estrogen inhibition of Tsp-1 is mediated by activation of ERK1/2 and JNK [57]. Additionally, Tsp-1 repression by estrogen is mediated via inhibition of both transcription and protein secretion. Conversely, androgen-mediated repression of Tsp-1 is solely mediated by inhibition of transcription, through an androgen-responsive element in the Tsp-1 promoter [58].
While hormone-mediated effects on tumor growth have been largely studied through their actions on hormone-responsive tumor cells, it has also been demonstrated that estrogen can induce angiogenesis on a systemic level [59]. This study revealed that estrogen receptor (ER)-positive stromal cells stimulate angiogenesis and promote tumor growth in response to estrogen even when the tumor cells were ER negative.
It has also been demonstrated that the peroxisome proliferator-activated receptors (PPAR) , another nuclear receptor family, can regulate both VEGF and Tsp-1 expression. Specifically, it has been demonstrated that tumor cells injected into PPARα−/− mice remained dormant for a prolonged period of time [60]. Moreover, the dormancy of these tumors was due to increased Tsp-1 expression in the host stroma. Surprisingly, it was later determined that fenofibrate and WY14643, two agonists of PPARα, also stimulated the expression of Tsp-1 [61]. These seemingly discordant results suggest that in the absence of PPARα, another member or the PPAR family, perhaps, may compensate and stimulate the expression of Tsp-1. Of note, PPARγ also stimulates the expression of CD36 [62], a receptor for Tsp-1. In keeping with these observations, it was demonstrated that the PPARγ agonists rosiglitazone and pioglitazone inhibit bFGF and VEGF-mediated angiogenesis [63].
Matrix Metalloproteases
The ability of tumors to invade locally, across the basement membrane, is critical for tumor growth and ultimately metastasis. One critical step in tumor invasion and migration is the remodeling of the extracellular matrix by extracellular proteases. Some of the major players in this field are the matrix metalloproteases or MMPs. For example, an experiment in which MCF7 breast cancer cells and fibroblasts were co-injected into mice resulted in the significant acceleration of tumor growth [64]. Moreover, in a parallel experiment in which the fibroblasts ectopically expressed TIMP-2 (tissue inhibitor of metalloprotease 2), an inhibitor of MMP activity, the tumor-stimulating activity was abrogated [65]. Analogously, administration of a broad-spectrum MMP inhibitor, batimastat, also abrogated the ability of fibroblasts to stimulate tumor formation by MCF7 cells [65].
The matrix remodeling carried out by MMPs not only facilitates tumor cell migration into the surrounding microenvironment but also stimulates the migration of endothelial cells into the tumor by facilitating the formation of the leading edge of new blood vessels. MMPs also liberate growth factors, such as VEGF and bFGF that are otherwise sequestered in the ECM. The ability of MMPs to stimulate angiogenesis was established in an elegant genetic experiment in which tumor-prone RIP-TAG2 mice were crossed with various matrix protease knockout mice [66]. By crossing the RIP-TAG mice with MMP2 knockout mice, the authors demonstrated that tumor growth was impaired but not due to any defect in angiogenesis [66]. Conversely, MMP9−/− RIP-TAG mice displayed inhibited tumor growth and defective angiogenesis [66]. In addition to cleaving matrix proteins, MMP9 also cleaves the latency-associated peptide from TGF-β, converting it to the active form and thereby stimulating tumor growth in a mammary tumor model [67].
Thrombospondin-1
While much of the attention in the field of angiogenesis has been paid to the identification and characterization of pro-angiogenic factors, the studies detailing the role of one of the most potent anti-angiogenic proteins, Tsp-1, should not be overlooked. Thrombospondin-1 (Tsp-1) is an endogenous anti-angiogenic protein that functions via a multimodal approach: it binds to cell surface receptors CD36 and CD47 on the endothelial cell surface and renders the cell insensitive to both VEGF and bFGF, as well as inducing caspase-dependent apoptosis mediated by downstream signaling from CD36 [68–71]. Tsp-1 also binds to MMP9 and functionally inactivates it [66, 72]. In tumor cells, Tsp-1 expression is repressed via a signal transduction cascade emanating from PI3 kinase via Rho GTPase to ROCK to Myc, which represses Tsp-1 in a phosphorylation-dependent manner [73]. This pathway has been shown to be active in several human breast cancer cell lines in which Tsp-1 expression was virtually silenced [73]. Furthermore, in a majority of the surveyed breast cancer cell lines, the pathway previously described [74] was demonstrated to be responsible for the silencing. Thus, this pathway represents the first biochemical elucidation of a cell-autonomous “angiogenic switch .”
While the expression of VEGF in the tumor-associated stroma is widely accepted to have a positive correlation with tumor progression [28, 75, 76], the role of thrombospondin-1 (Tsp-1) expression in the tumor-associated stroma is unclear. Tsp-1 expression by epithelial tumor cells is observed infrequently, and ectopic expression of Tsp-1 is inhibitory to tumor growth [20, 73, 77]. Stromal Tsp-1, meanwhile, has been correlated with a desmoplastic response and increased invasiveness in a subset of breast cancers [75, 78, 79], while it has been demonstrated to be inhibitory to early-stage breast cancers [80]. Expression of Tsp-1 by stromal fibroblasts has been shown to inhibit tumor formation and growth [81]. Intriguingly, the same report demonstrated that tumors that arose in an environment high in Tsp-1 eventually overcame the inhibitory effects of this protein by increasing their production of VEGF. Thus, the complex interrelationship between these two proteins and their relative expression levels in the tumor-associated stroma can play a key role in the induction and maintenance of the angiogenic phenotype in human tumors.
The work described above demonstrated that VEGF expression in the stroma is a critical component in tumor-mediated angiogenesis. Conversely, Tsp-1 expression in the tumor-associated stroma can be a potent inhibitor of tumor angiogenesis and growth. The question that arises then is how do tumors stimulate the expression of VEGF in the stroma while concomitantly repressing the expression of Tsp-1?
Nonprotein Mediators of Angiogenesis
The vast majority of studies of angiogenesis focus on the roles of cytokines and growth factors. However, one largely understudied signaling mechanism is lipid and phospholipid signaling. Significantly, two landmark studies demonstrated that these molecules could regulate the expression of Tsp-1. Two independent studies showed that generation of phospholipids and the resultant signaling pathways potently repress Tsp-1 expression in stromal fibroblasts. The first demonstrated that platelet-mediated generation of phospholipids, specifically sphingosine-1-phosphate (S1P), downregulated Tsp-1 expression in dermal fibroblasts by activating the Gi-protein-coupled S1P receptors [82].
The second study also implicated S1P as a repressor of Tsp-1 by demonstrating that secretion of a low molecular weight molecule (<3kD) was upregulated in Ras-transformed cells and that it repressed Tsp-1in dermal fibroblasts in an S1P-dependent manner [83]. These two reports indicate that tumor cells augment the angiogenic output of their microenvironment by secreting factors that repress Tsp-1 in the surrounding stromal cells.
Carcinoma-Associated Fibroblasts
Tumor progression is intricately regulated by the interactions with fibroblasts present in the tumor microenvironment [84]. Fibroblasts present in the tumor microenvironment are referred to as carcinoma-associated fibroblasts (CAFs) [10]. Based on genetic analysis, CAFs are very similar, virtually identical, to activated fibroblasts found in the stroma of damaged or wounded tissue [85–88]. Specifically, both express smooth muscle actin, EGF [89, 90], HGF [91–95], IGF-I, and IGF-2 [96–98] as well as matrix metalloproteases (MMPs) [65, 99–108].
Strikingly, underscoring the importance of their contribution to tumor progression, some carcinomas are comprised of up to 90% fibroblasts [109]. While the paracrine signaling mechanisms that convert normal fibroblasts to CAFs have not been completely delineated, in vitro studies have demonstrated that TGF-β can induce CAF-like properties in normal fibroblasts [110]. Moreover, human carcinoma cells have been observed to convert normal fibroblasts into CAFs in a mouse xenograft model [111]. The CAF phenotype is also very stable as they can be cultured in the absence of carcinoma cells in culture until they undergo senescence [112].
When carcinomas progress to the invasive state, the basement membrane is degraded, and stromal cells, including CAFs, inflammatory response cells, and newly formed capillaries, come into contact with the tumor cells [113]. CAFs in the stroma of invasive carcinoma continue depositing large amounts of ECM, including tenascin C in some cases [114, 115]. It has been shown that in breast and bladder carcinomas, expression of tenascin C correlates with increased tumor invasiveness [116, 117]. The accumulation of ECM in tumors contributes to increased interstitial fluid pressure that hinders oxygen and nutrient diffusion [118, 119]. Thus, CAF-mediated hypoxia could lead to the expression of HIF-1α and the induction of VEGF, thus providing a mechanism by which CAFs can promote angiogenesis in tumors.
An elegant study utilizing both in vivo and in vitro models demonstrated that injecting human breast cancer cells mixed with CAFs into the mouse mammary gland resulted in tumors that grew faster and were more angiogenic than when tumor cells were mixed with normal fibroblasts [112]. The increase in tumor growth was mediated by the production and secretion of stromal cell-derived factor 1 (SDF1) by CAFs which, in turn, bound to and activated its cognate receptor, CXCR4, on the surface of tumor cells. Moreover, the CAF-secreted SDF1 also stimulated angiogenesis by recruiting endothelial progenitor cells (EPCs) to the tumor.
Another study demonstrating the tumor-promoting activity of CAFs utilized a genetically engineered mouse model in which the Mts1 gene, which stimulates tumor metastasis, was knocked out. When otherwise metastatic breast cancer cells were injected into these mice, they were unable to form metastases [120]. However, when tumor cells were mixed with Mts1-expressing fibroblasts and injected into Mts1 knockout mice, the metastatic potential of these tumors was partially restored.
Bone Marrow-Derived Cells
In addition to fibroblasts, the tumor microenvironment is made up of several other nonresidents that were not present prior to tumorigenesis but migrated to the tumor. The most prominent among these cells are bone marrow-derived cells: mesenchymal stem cells, macrophages, neutrophils, mast cells, and T cells. These cells migrate in response to the growing tumor mass and by the secretion of discrete growth factors and chemokines produced by the tumor cells, which create a wound-like environment.
Macrophages
By far, the most prevalent nonresident cells present in tumors are tumor-associated macrophages (TAMs) [121]. Activated macrophages, those recruited to sites of inflammation, are generally categorized into two types: M1 and M2 [122–124]. M1 macrophages are effector cells that are able to potently kill microorganisms as well as tumor cells [122]. They also secrete high levels of proinflammatory cytokines [122]. M2 macrophages scavenge debris and stimulate angiogenesis as well as tissue remodeling and repair [122, 125–128]. TAMs are most similar to M2 macrophages.
TAMs have been shown to stimulate the growth and progression of both human and experimental tumor models [129]. TAMs are also preferentially recruited to sites of hypoxia, which in non-tumorigenic contexts is symptomatic of damaged or inflamed tissue [129]. Hypoxia stimulates the activity of the transcription factor HIF-1, which activates the expression of the pro-angiogenic growth factors VEGF, bFGF, TNFα, and CXCL8 [129].
While the TAMs normally stimulate tumor, they can also inhibit tumor growth. For example, CSF has been shown to stimulate production and secretion of metalloelastase by macrophages [130, 131]. Metalloelastase is an extracellular protease that cleaves plasminogen into multiple fragments, one of which is the anti-angiogenic protein angiostatin [132]. Thus, the effects of macrophage recruitment on tumor growth are highly context dependent.
Mast Cells
Mast cells are multifunctional secretory cells, characterized by numerous large electron-dense granules comprised of proteoglycans, predominantly heparin [133]. Mast cells are the progeny of pluripotent bone marrow progenitor cells, which are characterized as positive for CD34, c-kit, and CD13 [134]. In the circulation, mast cells are progenitor-like cells that differentiate/mature after being recruited to a given tissue. Mast cells express and secrete a myriad of proteases, most notably chymases, tryptases, and matrix metalloproteases, which are stored in secretory granules [133]. These proteases, specifically MMP2 and MMP9, are crucial for the ability of mast cells to stimulate tissue repair and remodeling [135]. Additionally, mast cell secretory granules are depots for cytokines and growth factors, including VEGF, bFGF, TNF-α, GM-CSF, SCF, EGF, PDGF, IFN-gamma, multiple interleukins, and chemokines, such as MIP-1 α and MCP-1 [133]. The release of proteases, cytokines, and growth factors stored in the secretory granules of macrophages can be triggered by multiple cytokines, including IL-1, IL-3, GM-CSF, platelet factor 4, IL-8, SCF, (MCP)-1, and MIP-1 alpha [136]. Moreover, mast cells also produce and secrete matrix metalloproteases (MMPs) MMP2 and MMP9, which have been shown to promote angiogenesis by liberating VEGF and bFGF from the extracellular matrix [137, 138]. Interestingly, mast cells have been shown to be recruited to tumors by the pro-angiogenic proteins VEGF, bFGF, and TGF-β [139, 140]. Thus, conditions within a tumor that necessitate the growth of new blood vessels recruit mast cells, which in turn further stimulate angiogenesis.
Experimental evidence for the functional role of mast cells in angiogenesis and tumor growth was provided by an elegant murine genetic model in which Myc expression in β cells was driven via fusion to a mutant form of the estrogen receptor [141]. In this model it was demonstrated that Myc activation by systemic administration of 4-hydroxy tamoxifen induced β-cell tumors that were characterized by blood vessel infiltration accompanied by mast cell recruitment. These findings indicated that mast cells are required for angiogenesis at the onset of tumorigenesis and for maintenance of angiogenesis during tumor growth and progression.
Mesenchymal Stem Cells
Mesenchymal stem cells (MSCs) are bone marrow-derived cells that have the ability to differentiate into a myriad of cells of mesenchymal lineage including fibroblasts, osteoblasts, chondrocytes, adipocytes, pericytes, and muscle cells. MSCs are an extremely rare cell type within the bone marrow, comprising between 0.01% and 0.001% of the mononuclear cells [142, 143]. Human MSCs are defined by the expression CD44 adhesion molecule (HCAM), CD73, CD90, CD105 (endoglin), CD106 (VCAM-1), and STRO-1 [144].
MSCs have been shown to be recruited to sites of wounding or inflammation, as well as to tumors [145]. MSCs are recruited to tumors by multiple different growth factors and cytokines, including VEGF, bFGF, IL-8, EGF, HGF, and PDGF as well as CCL2, CCL7, and CXCL12 (SDF-1) [146–150]. Following recruitment to the tumor, MSCs have been shown to secrete VEGF to stimulate angiogenesis [151]. Moreover, in melanoma, a correlation has been demonstrated between MSCs and angiogenesis [152].
In addition to correlation and expression studies, MSCs have been demonstrated to stimulate angiogenesis in in vitro models as well as in murine pancreatic xenografts [153]. In that study, tumors formed after injecting wild-type MSCs had twice as many blood vessels as control tumors. Conversely, tumors in mice injected with MSCs, in which VEGF had been silenced by lentiviral shRNA, had comparable numbers of blood vessels to control tumors [153]. Thus, the ability of MSCs to home to tumors and secrete VEGF stimulates tumor growth via enhanced angiogenesis.
Neutrophils
While TAMs are the most prevalent and common leukocyte present in the tumor microenvironment, neutrophils are the most abundant leukocyte in the circulation in cancer patients [136]. Neutrophil recruitment from the bone marrow is mediated, in part, by the chemokine CXCL12 (SDF-1) as its cognate receptor, CXCR4, is expressed at high levels on the cell surface of neutrophils [154]. There are two types of neutrophils present in the circulation: circulating neutrophils, which, as their name suggests, are freely circulating and are recruited to tumors [155, 156], and marginated neutrophils, which are bound to the endothelium of capillaries [136]. The marginated pool can be mobilized into the circulating pool by cytokines such as IL-6 [157, 158].
Elevated levels of neutrophils are associated with multiple human tumors, including colon, lung, melanoma, myxoid fibrosarcoma, and gastric carcinoma [159–162]. In addition to CXCL12, one of the most potent chemoattractants of neutrophils is CXCL8, which is expressed by both tumor and stromal cells in many types of tumors [159, 163]. Once recruited to tumors, neutrophils stimulate angiogenesis by secreting VEGF and matrix metalloproteases, which release angiogenic growth factors from sequestration in the extracellular matrix [164, 165].
In a genetic murine model of squamous cell skin carcinoma, it was observed that the source of MMP9 in the skin tumors was not from the tumors themselves but from neutrophils. Specifically, it was found that MMP9 produced and secreted by neutrophils was required for the angiogenic switch [138]. These results have since been recapitulated using anti-GR1 antibody-mediated neutrophil ablation in the RIP-TAG2 islet cell tumor model as well as a human ovarian cancer xenograft model in MMP9 deficient mice [166, 167].
Similar to what has been observed with macrophages and mast cells, neutrophils also possess antitumor activity. For example, in 1975 it was observed that neutrophils could kill tumor cells [168]. The original conclusion was that the killing was mediated exclusively by myeloperoxidase. However, it was later shown that neutrophils kill tumor cells via multiple mechanisms including secreting proteases, membrane-perforating agents, reactive oxygen species, and cytokines such as TNFα and IL-1β [169]. Additionally, neutrophils can actually inhibit angiogenesis via two distinct mechanisms, mediated by the same protease—neutrophil elastase. The first mechanism is characterized by neutrophil elastase degradation of VEGF and bFGF [170]. Secondly, neutrophil elastase also cleaves plasminogen into angiostatin, which inhibits VEGF- and bFGF-mediated angiogenesis [171]. These findings underscore the complexity of the role of the tumor microenvironment in tumor angiogenesis and progression and serve as an example that analysis of any cell type of biomarker requires a more complete understanding of the contextual signals within the tumor microenvironment.
Conclusion
Angiogenesis is a complex process that is stimulated by a myriad of growth factors and cytokines and inhibited by an equally diverse cohort of proteins. Accordingly, the regulation of angiogenesis by the tumor microenvironment is an extremely complex phenomenon. The signaling molecules secreted by tumors that act on stromal cells can often have different, and even opposite, activities with respect to the production of pro- and anti-angiogenic factors. Therefore, the composition of the tumor microenvironment as well as the stage of the tumor has profound effects on determining whether the tumor microenvironment is pro-angiogenic or anti-angiogenic. The complex signaling mechanisms described in this chapter provide a myriad of potential and, as yet largely untapped, targets for therapeutic intervention to inhibit tumor growth in patients. Ultimately, the strategy of targeting molecules that mediate processes, such as angiogenesis, via tumor-stromal interactions may prove to be hugely successful as the accounts of genomic instability and mutation in the cells comprising the microenvironment are exceedingly rare. The hope then is that anti-angiogenic therapy targeting the tumor microenvironment will result in lower rates and incidences of acquired resistance than traditional therapeutic strategies.
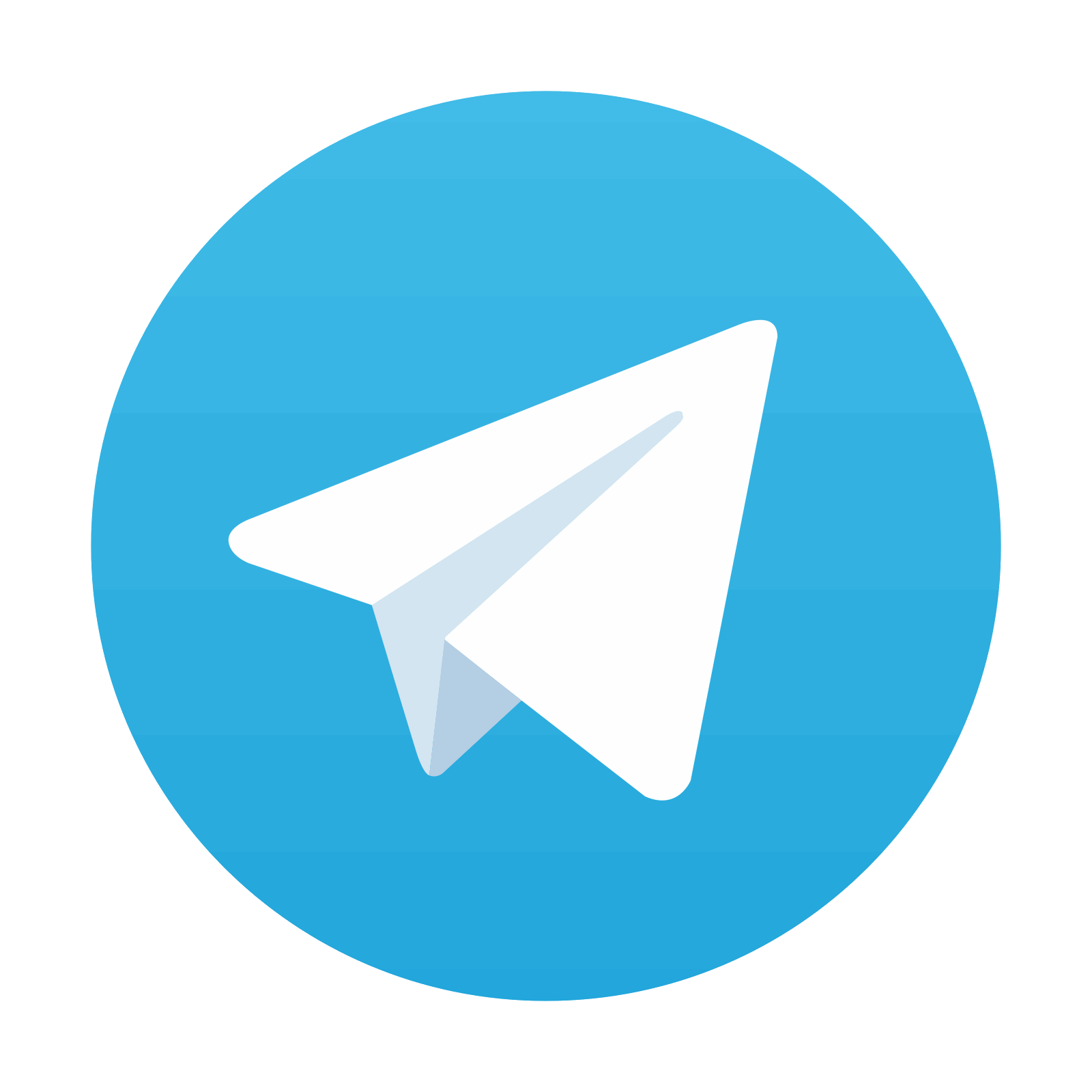
Stay updated, free articles. Join our Telegram channel

Full access? Get Clinical Tree
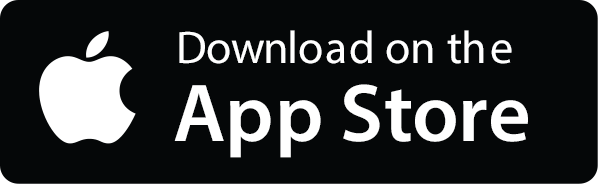
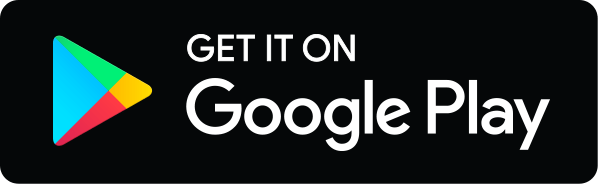