2.12.17 The Role of Solvents and Additives in Reactions of Samarium(II) Iodide and Related Reductants
T. V. Chciuk and R. A. Flowers, II
General Introduction
Since Kagan introduced samarium(II) iodide (SmI2, samarium diiodide) to chemists in 1980,[1] it has attained an importance reserved for only select reagents. It is straightforward to prepare and has reasonable solubility in electron-donor solvents. The majority of samarium(II) iodide based reactions are carried out using samarium(II) iodide in tetrahydrofuran, since the reagent is stable in this medium and is soluble up to 0.1 M. The unique place held by samarium(II) iodide in the arsenal of synthetic chemists is a result of its versatility in mediating numerous fundamentally important reactions, including functional group reductions (alkyl halides, carbonyls, and related functional groups), the cross coupling of reducible functional groups (Grignard and Barbier reactions, carbonyl–and alkyl halide–alkene couplings, and related cross-coupling reactions), and cascade reactions that proceed through free-radical and anionic intermediates. A consequence of its versatility is that it can be used for the synthesis of a wide range of multifunctional targets. Given the broad utility of the reagent, there are several outstanding reviews on the applications of samarium(II) iodide in synthesis.[2–8]
One of the fascinating features of samarium(II) iodide is that additives can be employed to tune the rate, diastereoselectivity, and chemoselectivity of reactions. Additives that are commonly utilized in reactions of samarium(II) iodide and other samarium(II)-based reductants can be classified into three major groups: (1) Lewis bases (hexamethylphosphoric triamide and other electron-donor ligands and chelating ethers); (2) proton donors (water, alcohols, and glycols); and (3) inorganic additives (e.g., NiI2, FeCl3, LiCl). In addition, the solvent milieu can also play an important role in the reactivity of samarium(II) reductants, predominantly through changes in the coordination sphere of the metal. The main focus of this chapter will be on the use of additives and solvent milieu to provide selective and efficient reactions, and at least one example will be given for each subclass of samarium(II)-promoted reactions. Given the large number of reviews available on samarium(II)-based reagents, the majority of examples will be from the last 25 years, and only seminal reactions and preparations before 1990 will be presented.
2.12.17.1 Synthesis of Samarium(II) Reductants
One of the benefits of samarium(II) iodide is its ease of preparation and storage. It can be stored for long periods in tetrahydrofuran under an inert atmosphere. Because it is stable, it can be conveniently employed as an intermediate for the synthesis of a range of samarium(II)-based reductants, as shown in ▶ Scheme 1.
Several methods for the synthesis of samarium(II) iodide are outlined in ▶ Section 2.12.17.1.1, followed by methods for the preparation of other samarium(II)-based reductants employed in synthesis in ▶ Sections 2.12.17.1.2–2.12.17.1.5.
2.12.17.1.1 Samarium(II) Iodide
Samarium(II) iodide can be purchased from several commercial sources, but it is also convenient to prepare by reaction of samarium metal in slight excess with either iodine, 1,2-diiodoethane, or iodoform. These reactions are typically carried out in tetrahydrofuran, but other solvents can be used. In tetrahydrofuran, the maximum concentration of reagent is approximately 0.1 M. It should be noted that the color of the solution alone cannot be used to determine that complete reaction has occurred, because solutions containing 0.1 M samarium(II) iodide are indistinguishable from solutions containing significantly less samarium(II) iodide. As a consequence, it is important to titrate solutions with iodine before use to measure the solution concentration of the reductant. One of the main issues that researchers face is the inconsistent preparation of samarium(II) iodide. A recent paper by Procter addresses this issue and provides a number of protocols designed to provide consistent concentrations of samarium(II) iodide in tetrahydrofuran.[9] In addition, Flowers has described the preparation of samarium(II) iodide and the procedure is available as an open-access video.[10] The examples in the sections below describe the most common methods for the preparation of samarium(II) iodide in tetrahydrofuran. Preparation in other solvents is only described when the procedure varies significantly from preparation in tetrahydrofuran.
2.12.17.1.1.1 Method 1: Synthesis in Tetrahydrofuran from Samarium and 1,2-Diiodoethane
A seminal report by Kagan describes the synthesis of samarium(II) iodide (1) from excess samarium metal and 1,2-diiodoethane, as shown in ▶ Scheme 2.[1] Ethene leaves as a gas and the excess samarium metal helps to stabilize samarium(II) iodide. The solution is stable for extended periods of time under an inert atmosphere, but it is recommended that the reagent be used within a few days of preparation.
At times, difficulties can be encountered in the preparation of samarium(II) iodide that result from the impurity of the reagents. Procter has examined this issue thoroughly, and a procedure has been described for the purification of 1,2-diiodoethane and subsequent preparation of samarium(II) iodide using Kagan’s method.[9]
Samarium(II) Iodide (1) in Tetrahydrofuran; Synthesis from Samarium and 1,2-Diiodoethane:[1]
A flask containing Sm powder (3 g, 0.02 mol) and a magnetic stirrer was fitted with a dropping funnel containing 1,2-diiodoethane (2.82 g, 0.01 mol) dissolved in THF (250 mL). The THF soln was added dropwise with stirring at rt to produce a 0.04 M soln of SmI2.
Samarium(II) Iodide (1) in Tetrahydrofuran; Synthesis from Samarium and Purified 1,2-Diiodoethane:[9]
1,2-Diiodoethane (20 g) was dissolved in Et2O (400 mL) and the soln was washed with sat. aq Na2S2O3 (5 × 100 mL) and once with H2O (100 mL). The Et2O soln containing 1,2-diiodoethane was dried (Na2SO4) and concentrated to provide a white solid that was wrapped in aluminum foil and placed under vacuum for 30 min. Once this was complete, an oven-dried, 100-mL, round-bottomed flask containing a Teflon-coated stirrer bar and septum was placed under a positive pressure of argon (or N2). Next, Sm metal (1.65 g, 11.0 mmol) and the freshly prepared 1,2-diiodoethane (1.55 g) were weighed out and added to the round-bottomed flask, which was sealed with a septum. The flask was stirred at medium speed and THF (55 mL) was added using a syringe. The flask was removed from the inert-gas line, the septum was sealed with Parafilm, and the mixture was allowed to stir overnight before use.
2.12.17.1.1.2 Method 2: Synthesis in Tetrahydrofuran from Samarium and Iodine
Following the publication of the preparation of samarium(II) iodide by Kagan, Imamoto described an alternative, atom-efficient method that employs iodine and samarium metal in tetrahydrofuran (▶ Scheme 3).[11] It should be noted that this original report involved refluxing tetrahydrofuran, but subsequent studies have shown the reaction proceeds at room temperature.
One of the major issues with the preparation of samarium(II) iodide is the quality of the samarium metal. Procter has examined this issue carefully and has developed a “dry-stirring” method that is useful in reactivating “inactive” samarium metal.[9] If difficulties are encountered using the Imamoto method, it is recommended that this variation be used.
Often, chemists prefer a rapid approach for the synthesis of samarium(II) iodide and related reagents. Hilmersson has shown that microwave-assisted heating of samarium and iodine in tetrahydrofuran allows for the rapid synthesis of samarium(II) iodide.[12] The concentration can be determined using the reduction of heptan-2-one by the water/amine system developed by Hilmersson. Since there are no side reactions, two equivalents of samarium(II) iodide are consumed for every equivalent of ketone; a white precipitate of samarium(III) hydroxide is formed (▶ Scheme 4).
Flowers has also shown that ultrasound (20 kHz) can be used to accelerate the synthesis of samarium(II) iodide.[13] One advantage of the process is that supersaturated solutions of samarium(II) iodide can be easily prepared.
Samarium(II) Iodide (1) in Tetrahydrofuran; Synthesis from Samarium and Iodine:[11]
I2 (5.1 g, 20 mmol) was added to a stirring mixture of Sm powder (40 mesh; 3.3 g, 20 mmol) in dry THF (200 mL). The resulting suspension turned from yellow to green and was refluxed overnight to produce a 0.1 M soln of SmI2.
Samarium(II) Iodide (1) in Tetrahydrofuran; Synthesis from Samarium and Iodine Using the “Dry-Stirring” Method:[9]
A 110-mL Schlenk tube containing a Teflon-coated magnetic stirrer bar and fitted with a septum was flame-dried under vacuum. Once the tube had cooled to rt, it was evacuated and backfilled with argon (3 ×). Next, Sm metal (1.65 g, 11.0 mmol) was added to the tube, which was sealed with the septum and evacuated and backfilled with argon (3 ×). Once this process was complete, the tube was kept under a positive pressure of argon and the Sm metal was stirred at medium to high speed for 24 h. Once the Sm had been dry stirred, THF (45 mL) was added under argon and then I2 (1.40 g, 5.5 mmol) was added. The tube was then heated to 60 °C for 18 h. After the soln had cooled and settled for 2 h, it was titrated with I2 to determine the final concentration of SmI2.
Samarium(II) Iodide (1) in Tetrahydrofuran; Microwave-Assisted Synthesis from Samarium and Iodine, with Titration Using Heptan-2-one:[12]
Sm powder (113 mg, 0.75 mmol) and I2 (127 mg, 0.5 mmol) were weighed and diluted with dry THF (5 mL) under a N2 atmosphere. A magnetic stirrer bar was added to the vessel, which was then sealed. The vessel containing the mixture was inserted into a microwave oven and irradiated at 180 °C for 5 min to yield a soln of SmI2. To determine the concentration of SmI2, the reagent soln was centrifuged and a 5-mL portion of the soln was placed in a test tube containing a magnetic stirrer and fitted with a septum under an inert atmosphere. Et3N (approx. 4 equiv) and H2O (approx. 6 equiv) were added to the soln by syringe. Next, 0.07 M heptan-2-one in THF was added dropwise with vigorous stirring until the soln turned colorless and a white suspension appeared. The volume of heptan-2-one was noted and the concentration of SmI2 was determined using the balanced formula shown in ▶ Scheme 4.
Samarium(II) Iodide (1) in Tetrahydrofuran; Ultrasound-Assisted Synthesis from Samarium and Iodine:[13]
Sm powder (40 mesh; 0.62 g, 4 mmol) and I2 (0.64 g, 2.52 mmol) were added to THF (20 mL) at 25 °C in a flame-dried vial under an inert atmosphere. The mixture was sonicated for 7 min using a 750-W, 0.5-inch, full-wave probe working at a fixed frequency of 20 kHz and 40% of maximum amplitude. The concentration of the resulting sample was determined by iodometric titration.
2.12.17.1.1.3 Method 3: Synthesis in Tetrahydropyran
Kagan has developed a method for the synthesis of samarium(II) iodide from samarium metal and 1,2-diiodoethane in tetrahydropyran (▶ Scheme 5) that is simple and very similar to the method he developed using tetrahydrofuran as solvent (▶ Section 2.12.17.1.1.1).[14]
Samarium(II) Iodide (1) in Tetrahydropyran:[14]
To a mixture of Sm powder (601 mg, 4.00 mmol) and 1,2-diiodoethane (1.127 g, 4.00 mmol) under argon at rt was added THP (4.00 mL) with vigorous stirring. After a short induction period, a slightly exothermic reaction took place and the mixture turned dark blue. After 8 h at rt, a slurry of SmI2 was obtained.
2.12.17.1.1.4 Method 4: Synthesis in 1,2-Dimethoxyethane
Flowers has reported the use of 1,2-dimethoxyethane as an alternative solvent for samarium(II) iodide in a preparation from samarium metal and iodine (▶ Scheme 6). The solubility of samarium(II) iodide when first generated in 1,2-dimethoxyethane was measured to be 0.1 M, but after a few days dropped to 0.02 M.[15]
Samarium(II) Iodide (1) in 1,2-Dimethoxyethane:[15]
I2 (0.34 g, 1.3 mmol) was dissolved in dry DME (100 mL) and Sm metal (0.34 g, 2.3 mmol) was added to the soln. The mixture was allowed to stir overnight under a N2 atmosphere, during which time the characteristic blue color of SmI2 in DME appeared, indicating the presence of a divalent samarium species. The concentration of SmI2 was determined by iodometric titration to be approximately 0.02 M.
2.12.17.1.1.5 Method 5: Synthesis in Acetonitrile and Other Nitriles
Although Inanaga was the first to report the use of samarium(II) iodide in acetonitrile, a detailed procedure for the generation of the reactant in that solvent was not provided.[16] In 1992, Ishii and co-workers reported a method for generating samarium(II) iodide in acetonitrile using sodium iodide and chlorotrimethylsilane (▶ Scheme 7).[17]
Scheme 7 Synthesis of Samarium(II) Iodide in Acetonitrile from Samarium, Sodium Iodide, and Chlorotrimethylsilane[17]

Ruder has reported a simple method for the generation of samarium(II) iodide in acetonitrile using 1,2-diiodoethane (▶ Scheme 8), as in the synthesis in tetrahydrofuran.[18]
A simple alternative method for the generation of samarium(II) iodide in acetonitrile was reported by Flowers in 2004 (▶ Scheme 9).[15] By iodometric titration, the solubility of samarium(II) iodide in acetonitrile was found to be limited to 0.05 M.
Kagan has also reported the preparation of samarium(II) iodide in alternative nitriles (e.g., octanenitrile and pivalonitrile) (▶ Scheme 10). Work with pivalonitrile has shown that unique chemistry can be achieved using this solvent due to the absence of acidic hydrogens as well as the low reactivity of the hindered nitrile group. Another advantage gained by switching to this solvent is that it is easily removed in the workup. Furthermore, although the use of this solvent leads to slower reactions, it provides increased regioselectivity for Barbier reactions.[19]
Samarium(II) Iodide (1) in Acetonitrile; Synthesis from Samarium, Sodium Iodide, and Chlorotrimethylsilane:[17]
To a soln of NaI (0.9 g, 6 mmol) in MeCN (20 mL) under an N2 atmosphere at rt was added TMSCl (0.76 mL, 6 mmol). Sm metal (0.3 g, 2 mmol) was added to the resulting soln and the mixture was stirred at rt for 3 h to form a suspended dark green soln. THF could also be used as the solvent.
Samarium(II) Iodide (1) in Acetonitrile; Synthesis from Samarium and 1,2-Diiodoethane:[18]
Note: MeCN was distilled from CaH2, combined with 1,2-diiodoethane, and then deoxygenated using the freeze–thaw method before addition to Sm metal.
Sm metal (1.2 mmol) was placed in a flame-dried flask under N2 and 1,2-diiodoethane (0.6 mmol) in dry MeCN (7 mL) was added via cannula. After approximately 1 h, the soln was green-blue in color, indicating formation of SmI2.
Samarium(II) Iodide (1) in Acetonitrile; Synthesis from Samarium and Iodine:[15]
I2 (3.2 g, 13 mmol) was dissolved in dry MeCN (250 mL). Sm metal (5.0 g, 30 mmol) was added to the soln. The mixture was allowed to stir overnight under a N2 atmosphere, during which time the characteristic dark green color of SmI2 in MeCN appeared, indicating the presence of a divalent Sm species. The concentration of SmI2 was determined by iodometric titration to be approximately 0.05 M.
Samarium(II) Iodide (1) in Nitriles; Synthesis from Samarium and 1,2-Diiodoethane:[19]
To a mixture of Sm powder (601 mg, 4.00 mmol) and 1,2-diiodoethane (1.127 g, 4.00 mmol) under argon at ambient temperature was added the nitrile solvent (4.00 mL) with vigorous stirring. After a short induction period, a slightly exothermic reaction took place and the mixture turned dark green. After 8 h at rt, a slurry of SmI2 was obtained. For pivalonitrile and octanenitrile, heating at 40 °C was needed to consume all the Sm metal. Complexometric, argentometric, and iodine titrations were in agreement with quantitative formation of SmI2.
2.12.17.1.1.6 Method 6: Synthesis in Benzene/Hexamethylphosphoric Triamide
A great deal of work has been performed by Tani and co-workers in the area of samarium(II) iodide reactivity in benzene/hexamethylphosphoric triamide (▶ Scheme 11). A characteristic procedure for generation of the reductant under these conditions is provided below.[20]
Scheme 11 Synthesis of Samarium(II) Iodide in Benzene/Hexamethylphosphoric Triamide from Samarium and 1,2-Diiodoethane[20]

Samarium(II) Iodide (1) in Benzene/Hexamethylphosphoric Triamide:[20]
CAUTION:
Hexamethylphosphoric triamide is a possible human carcinogen and an eye and skin irritant.
A soln of 1,2-diiodoethane (3.0 g, 10.6 mmol) in benzene (96 mL) (CAUTION: carcinogen) containing HMPA (10.7 mL, 57.8 mmol) was added slowly to Sm powder (2.4 g, 16.0 mmol) at 100 °C under N2. The mixture was refluxed with stirring overnight to give a purple soln. The concentration of the soln was determined by back titration (treatment with an excess of a soln of I2 in dry toluene, then the remaining I2 was titrated with Na2S2O3).
2.12.17.1.2 Samarium(II) Bromide and Samarium(II) Chloride
Although samarium(II) iodide is the most utilized samarium(II) halide in organic synthesis, samarium(II) bromide and samarium(II) chloride have also found application. The primary shortcoming of these reagents is their limited solubility in organic solvents. Nevertheless, there are several instances where there are advantages to using these reagents over samarium(II) iodide. Several syntheses of these reagents were reported before 1980, but they required conditions that were not amenable to easy preparation. The syntheses of samarium(II) bromide and samarium(II) chloride reductants described in the sections below are more recent methods that can be readily performed in synthetic organic laboratories.
2.12.17.1.2.1 Method 1: Synthesis of Samarium(II) Bromide from Samarium(III) Oxide and Hydrobromic Acid
Kagan has developed asynthesis of samarium(II) bromide that proceedsthrough the conversion of samarium(III) oxide (Sm2O3) into a samarium(III) bromide hydrate, which is dried and subsequently reduced with lithium metal in tetrahydrofuran to produce a suspension of samarium(II) bromide (▶ Scheme 12).[21]
Samarium(II) Bromide (2) in Tetrahydrofuran:[21]
An 8.65 M aq soln of HBr (100 mL) was added to a dispersion of Sm2O3 (34.8 g, 0.1 mol) in H2O (100 mL) over 2 min, maintaining a temperature below 45 °C. The yellow soln was stirred for 2 h and then concentrated under reduced pressure. Further H2O (100 mL) was added and the soln was concentrated again. Next, EtOH (200 mL) was added and the soln was concentrated again. The solid was washed with hexane (200 mL) and dried, providing SmBr3·6H2O; yield: 99.5%.
SmBr3·6H2O (30 g, 60 mmol) in a Schlenk tube was diluted with trimethyl orthoformate (49.4 mL, 0.45 mol) and the mixture was stirred for 1 h. The soln was concentrated by distillation, and then dry THF (150 mL) was added and then distilled off. This process was repeated twice. After cooling, THF (300 mL) was added, followed by Li (0.42 g, 60 mmol). The mixture was stirred for 20 h, providing a quantitative yield of SmBr2 suspended in THF.
2.12.17.1.2.2 Method 2: Synthesis of Samarium(II) Bromide from Samarium and 1,1,2,2-Tetrabromoethane
A more recent method for the synthesis of samarium(II) bromide by reduction of 1,1,2,2-tetrabromoethane has been developed by Namy (▶ Scheme 13).[22] The preparation provides good yields of samarium(II) bromide suspended in tetrahydrofuran.
Hilmersson has developed a similar approach for the synthesis of samarium(II) bromide using excess samarium metal and 1,1,2,2-tetrabromoethane followed by microwave irradiation. The main advantage of this approach is that the time required for synthesis of the reductant is reduced from several hours to five minutes.[12]
Samarium(II) Bromide (2) in Tetrahydrofuran; Synthesis from Samarium and 1,1,2,2-Tetrabromoethane:[22,23]
1,1,2,2-Tetrabromoethane (415 mg, 1.2 mmol) was dissolved in THF (24 mL). The soln was degassed at −78 °C. Once degassed, the soln was added by syringe to Sm powder (40 mesh; 360 mg, 2.40 mmol) and stirred at rt for 16 h. The reaction produced a black suspension of SmBr2. Because the reductant is relatively unstable, it was prepared immediately before use.
Samarium(II) Bromide (2) in Tetrahydrofuran; Microwave-Assisted Synthesis from Samarium and 1,1,2,2-Tetrabromoethane:[12]
Sm powder (340 mg, 2.28 mmol) was placed in a microwave vessel. Next, THF (3.5 mL) was added by syringe, followed by 1,1,2,2-tetrabromoethane (89 μL, 0.75 mmol). A magnetic stirrer bar was added and the vessel was sealed and irradiated in a microwave oven for 5 min at 180 °C, leading to a black suspension of SmBr2. The concentration was determined as described in ▶ Section 2.12.17.1.1.2.
2.12.17.1.2.3 Method 3: Synthesis of Samarium(II) Bromide from Samarium(II) Iodide and Lithium Bromide
Flowers has developed an alternative synthesis of samarium(II) bromide that employs samarium(II) iodide and lithium bromide, producing the reductant in situ (▶ Scheme 14).[24] The main advantage of this procedure is that the samarium(II) bromide remains soluble for several hours before precipitating out of solution. It has been proposed that because the starting samarium(II) iodide is soluble in tetrahydrofuran, displacement of the iodide by the bromide counterion leads to a reductant that is initially soluble, but aggregates over time.
Samarium(II) Bromide (2) in Tetrahydrofuran:[24]
LiBr (0.695 g, 8 mmol) was placed in a flame-dried, 100-mL, round-bottomed flask with a stirrer bar, and THF (5 mL) was added to the flask by syringe to dissolve the salt. Next, a 0.1 M soln of SmI2 in THF (20 mL, 2 mmol) was added to the flask and the mixture was allowed to stir for several minutes until a purple color appeared. The SmBr2 reductant precipitated slowly over several hours, so it was recommended that the reagent be used soon after preparation.
2.12.17.1.2.4 Method 4: Synthesis of Samarium(II) Chloride from Samarium(III) Chloride
The first synthesis of samarium(II) chloride by reduction of samarium(III) chloride using lithium naphthalenide in tetrahydrofuran was reported by Rossmanith in 1979 (▶ Scheme 15).[25] The procedure provides a high yield of insoluble samarium(II) chloride.
Samarium(II) Chloride (3):[25]
SmCl3 (3 g, 11.7 mmol) was combined in a flask with naphthalene (1.5 g, 11.7 mmol) and Li (80 mg, 11.5 mmol) in THF (60 mL). The mixture was heated at reflux with stirring for 6 h and cooled overnight, yielding a precipitate. The precipitate was removed from the soln by filtration and washed with THF (2 × 5 mL). The product was dried under vacuum; yield: 2.4 g (93%).
2.12.17.1.2.5 Method 5: Synthesis of Samarium(II) Chloride from Samarium(II) Iodide and Lithium Chloride
Flowers has developed an alternative synthesis of samarium(II) chloride that employs samarium(II) iodide and lithium chloride, producing the reductant in situ (▶ Scheme 16).[24] The preparation is modified slightly from the preparation of samarium(II) bromide described in ▶ Section 2.12.17.1.2.3.
Samarium(II) Chloride (3):[24]
LiCl (0.339 g, 8 mmol) was placed in a flame-dried, 100-mL, round-bottomed flask with a stirrer bar, and THF (5 mL) was added by syringe to dissolve the salt. Next, a 0.1 M soln of SmI2 in THF (20 mL, 2 mmol) was added and the mixture was allowed to stir for several minutes until a purple color appeared. The SmCl2 reductant precipitated quickly to produce a dispersion in THF.
2.12.17.1.2.6 Method 6: Synthesis of Samarium(II) Chloride in Water from Samarium(III) Chloride and Samarium
Matsukawa has developed an interesting synthesis of samarium(II) chloride in water using samarium(III) chloride and samarium. The reagent produced by this method is capable of carrying out pinacol couplings and Barbier reactions in water, but otherwise has had limited use in synthesis. The reagent is generated in situ with substrate present.[26] An example of the quantities and conditions used for the application of samarium(II) chloride in a pinacol coupling is given in ▶ Scheme 17 and the accompanying experimental procedure.
Scheme 17 Synthesis of Samarium(II) Chloride in Water by Disproportionation of Samarium(III) Chloride and Samarium, and In Situ Application in a Pinacol Coupling[26]

Samarium(II) Chloride (3); Typical Procedure for In Situ Generation and Use in Pincol Coupling of Benzaldehyde:[26]
To a mixture of Sm powder (450 mg, 3 mmol) and SmCl3 (364 mg, 1 mmol) in H2O was added PhCHO (106 mg, 1 mmol). After 36 h, the resultant yellow-green suspension was treated with 2 M HCl (10 mL) and extracted with Et2O. The organic layer was washed with NaHCO3 (2 ×) followed by brine. It was then dried and concentrated under reduced pressure. The crude product was subjected to flash column chromatography, and the coupled 1,2-diphenylethane-1,2-diol product was obtained; yield: 86mg (81%); ratio (dl/meso) 58:42.
2.12.17.1.3 Samarium(II) Trifluoromethanesulfonate
Samarium(II) trifluoromethanesulfonate [Sm(OTf)2, samarium(II) triflate] has been shown to have a number of applications in organic synthesis and can be used in place of samarium(II) iodide. There have been limited studies that compare the two reagents, but there are several cases where samarium(II) trifluoromethanesulfonate is more effective in providing higher diastereoselectivity in pinacol coupling reactions[27] and the addition of alkyl iodides to cyclic ketones.[28] Although tetrahydrofuran is the most common solvent employed, the reagent can also be readily synthesized in acetonitrile[29] and 1,2-dimethoxyethane.[30] The reductant is typically prepared by the reaction of samarium(III) trifluoromethanesulfonate with an equivalent of a Grignard or organolithium reagent; typically, a secondary organolithium provides better yields than a secondary Grignard. A number of other preparations have been developed. Several important preparations are described in the following sections.
2.12.17.1.3.1 Method 1: Synthesis from Samarium(III) Trifluoromethanesulfonate, Samarium Metal, and Ethylmagnesium Bromide
Inanaga and co-workers have found that the addition of ethylmagnesium bromide to samarium(III) trifluoromethanesulfonate in tetrahydrofuran at room temperature produces samarium(II) trifluoromethanesulfonate (▶ Scheme 18). The presence of samarium metal is found to be important in stabilizing the reductant.[31]
Scheme 18 Synthesis from Samarium(III) Trifluoromethanesulfonate, Samarium, and Ethylmagnesiuum Bromide[31]

Samarium(II) Trifluoromethanesulfonate (4) in Tetrahydrofuran:[31]
A mixture of Sm(OTf)3 (120 mg, 0.20 mmol) and Sm metal (2.0 mg, 0.02 mmol) was placed in a flask under argon. Next, THF (2 mL) was added, followed by the addition of 0.90 M EtMgBr in THF (0.22 mmol). The soln was stirred for 1 h, producing a purple color indicative of the Sm(II) reductant.
2.12.17.1.3.2 Method 2: Synthesis from Samarium(III) Trifluoromethanesulfonate and sec- Butyllithium
Several years after the reported synthesis of samarium(II) trifluoromethanesulfonate by Inanaga, Fukuzawa and co-workers presented a thorough study on the synthesis of the reagent using both Grignard and organolithium reagents to reduce samarium(III) trifluoromethanesulfonate. They found that sec-butyllithium provides the best results, and have suggested that the presence of lithium trifluoromethanesulfonate may play a role in subsequent reactions mediated by the reagent (▶ Scheme 19).[32]
Samarium(II) Trifluoromethanesulfonate (4) in Tetrahydrofuran:[32]
Sm(OTf)3 (1.2 g, 2.0 mmol) was placed in a 50-mL Schlenk tube under N2. The tube was sealed with a septum and heated to 200 °C under reduced pressure for 2 h. After cooling to rt, a magnetic stirrer bar was placed in the tube, which was then flushed with N2 and THF (5 mL) was added through the septum with stirring. After stirring for 1 h, 1.0 M s-BuLi in cyclohexane (2 mL, 2.0 mmol) was added to the mixture at −20 °C. The soln was warmed to 0 °C and then rt over the course of 1 h, producing a purple soln of Sm(OTf)2. The quantity of the reductant was determined to be 1.8 mmol by iodometric titration.
2.12.17.1.3.3 Method 3: Synthesis from Samarium Metal and 1,5-Dithioniabicyclo[3.3.0]octane Bis(trifluoromethanesulfonate)
Tani and Mashima have developed the first salt-free synthesis of samarium(II) trifluoromethanesulfonate using excess samarium metal and 1,5-dithioniabicyclo[3.3.0]octane bis(trifluoromethanesulfonate) in acetonitrile (▶ Scheme 20). The solid samarium(II) trifluoromethanesulfonate is stable under an inert atmosphere, and the bound acetonitrile ligands are labile. When the solid is placed in tetrahydrofuran, the ligated acetonitrile is displaced, providing the tetrahydrofuran solvate.[33] Although this procedure has not been used often in producing samarium(II) trifluoromethanesulfonate for follow-up reactions in organic synthesis, it remains the most direct approach to producing a salt-free reductant in acetonitrile.
Samarium(II) Trifluoromethanesulfonate–Acetonitrile Adduct 5:[33]
Finely divided Sm metal (938 mg, 6.24 mmol), 1,5-dithioniabicyclo[3.3.0]octane bis(trifluoromethanesulfonate) (1.392 g, 3.12 mmol), and I2 (39.5 mg, 0.156 mmol) were placed in a flask containing MeCN (40 mL) and the mixture was stirred at 50 °C for 24 h. Excess Sm was removed by centrifugation and all volatiles were removed under reduced pressure. The crude product was dissolved in MeCN (5 mL) and precipitated by adding toluene (25 mL). The supernatant was removed using a syringe and the precipitated solid was dried under reduced pressure to afford the MeCN solvate of Sm(OTf)2 as a purple powder; yield: 66%.
2.12.17.1.3.4 Method 4: Mercury-Catalyzed Reduction of Samarium(III) Trifluoromethanesulfonate
A straightforward synthesis of samarium(II) trifluoromethanesulfonate in 1,2-dimethoxyethane has been developed by Collin and co-workers.[30] The synthesis requires acatalytic amount of mercury to provide efficient reduction of samarium(III) trifluoromethanesulfonate by samarium metal (▶ Scheme 21). The reagent is useful in a wide range of cross-coupling reactions including Grignard, ketone–alkene, pinacol, and related coupling reactions in tetrahydrofuran.
Scheme 21 Reduction of Samarium(III) Trifluoromethanesulfonate by Samarium Metal in the Presence of Mercury[30]

Bis(1,2-dimethoxyethane)samarium(II) Bis(trifluoromethanesulfonate) (6):[30]
Sm(OTf)3 (11.95 g, 20 mmol), Sm metal (1.82 g, 12.1 mmol), and Hg (230 mg) were placed in a 500-mL Schlenk flask attached to a vacuum line and cooled to −78 °C in a dry ice–acetone slurry. Dry DME (120 mL) was condensed by vacuum distillation into the mixture, which was stirred under static vacuum at rt for 48 h. The contents of the flask were concentrated to dryness and extracted into THF (250 mL) in an argon-filled dry box. The Sm residue was filtered off and the filtrate was concentrated to dryness. After drying under reduced pressure for 3 h, the residue was mixed with DME (100 mL) and stirred at 40 °C for 1 h. This was followed by cooling to 0 °C and filtration, and the collected precipitate was dried under reduced pressure; yield: 17.2 g (91%).
2.12.17.1.3.5 Method 5: Synthesis from Samarium(III) Trifluoromethanesulfonate and Samarium Metal
Flowers has shown that high-intensity ultrasound (20 kHz) can be used to generate samarium(II) trifluoromethanesulfonate from samarium metal, samarium(III) trifluoromethanesulfonate, and catalytic iodine in a wide range of solvents including tetrahydrofuran, acetonitrile, 1,2-dimethoxyethane, and even alcohols (▶ Scheme 22).[13] During these studies, it was found that while samarium(II) trifluoromethanesulfonate can be prepared in several solvents, it is exceptionally stable in acetonitrile as long as it is stored under an inert atmosphere of argon or nitrogen. The reagent is stable in this solvent for well over 6 months, and shows no decrease in reactivity.
Scheme 22 Synthesis from Samarium Metal and Samarium(III) Trifluoromethanesulfonate Using Ultrasound[13]

One of the shortcomings of the synthesis of samarium(II) trifluoromethanesulfonate described above is that it requires the use of high-intensity ultrasound. After examining numerous approaches, it has been found that stirring samarium(III) trifluoromethanesulfonate with samarium metal, and catalytic iodine in acetonitrile over a 3 hour period also produces samarium(II) trifluoromethanesulfonate in high yield.[29]
Samarium(II) Trifluoromethanesulfonate (4) in Acetonitrile; Procedure Using Ultrasound:[13]
To Sm powder (40 mesh; 0.15 g, 1 mmol), Sm(OTf)3 (0.6 g, 1 mmol), and I2 (0.03 g, 10 mol%) in a flame-dried vial was added MeCN (15 mL). The soln was sonicated in pulsing mode for 6–7 min. The concentration was determined by iodometric titration. Typical yields were 85–90%, with a concentration of 0.07 M in MeCN.
Samarium(II) Trifluoromethanesulfonate (4) in Acetonitrile:[29]
Sm powder (40 mesh; 0.265 g, 1.76 mmol), Sm(OTf)3 (1.03 g, 1.72 mmol), and I2 (0.102 g, 0.4 mmol) were added to dry MeCN (20 mL) in a flame-dried, round-bottomed flask. The soln was stirred under an argon atmosphere for 3 h until a dark green soln was obtained. The concentration of the soln was determined by iodometric titration and found to be in the range of 0.09 to 0.1 M.
2.12.17.1.4 Samarium(II) Amides
Sterically bulky anionic ligands have played an important role in the development of samarium(II)-based chemistry. The most important nitrogen-based ligand is bis(trimethylsilyl)amide (hexamethyldisilazanide), which is used to produce samarium(II) bis(trimethylsilyl)amide [Sm{N(TMS)2}2]. The reagent, first introduced by Evans,[34] is well-characterized and has been used in a number of important synthetic transformations.[35–37] The most significant advantage of this reagent is its solubility in a variety of solvents such as tetrahydrofuran, toluene, and hexane. Recent work by Hilmersson has shown that choice of solvent is important in the reductive cleavage of alkyl fluorides by the tetrahydrofuran solvate of samarium(II) bis(trimethylsilyl)amide [Sm{N(TMS)2}2(THF)2].[38] Flowers has shown that reductions in hexanes are three orders of magnitude faster than in tetrahydrofuran, and mechanistic studies show an inhibitory role for tetrahydrofuran.[39] The most straightforward synthesis of the reagent is shown in ▶ Scheme 23.
Samarium(II) Bis(trimethylsilyl)amide Bis(tetrahydrofuran) Adduct (7):[34]
A 1 M soln of NaHMDS (9.5 mL, 9.5 mmol) in THF was added to a stirred soln of SmI2(THF)2(2.60 g, 4.74 mmol) in THF (10 mL) in an inert-atmosphere drybox. The soln was stirred for 4 h and the solvent was removed by rotary evaporation, producing a purple solid. The solid was dissolved in hexane and precipitated NaI was removed by filtration. The procedure was repeated until no more precipitate was evident; yield: 2.68 g (92%).
2.12.17.1.5 (η5-Cyclopentadienyl)samarium(II) Complexes
Ligands based on the cyclopentadienyl unit have played a seminal role in the development and mechanistic understanding of samarium(II)-based reductions. While there are a number of ligands based on the cyclopentadienyl unit, the most prevalent in reactions are η5-cyclopentadienyl (Cp) and η5-pentamethylcyclopentadienyl (Cp*). The insolubility of bis(η5-cyclopentadienyl)samarium(II) has limited its use in organic synthesis. Nevertheless, there are a few instances where the use of bis(η5-cyclopentadienyl)samarium(II) has benefits over samarium(II) iodide. Bis(η5-pentamethylcyclopentadienyl)samarium(II) is used mostly in inorganic and polymer synthesis.
2.12.17.1.5.1 Method 1: Synthesis of Bis(η5-cyclopentadienyl)samarium(II)
The most straightforward synthesis of bis(η5-cyclopentadienyl)samarium(II) was developed by Kagan and co-workers (▶ Scheme 24).[40] Despite the insolubility of bis(η5-cyclopentadienyl)samarium(II), reactions with benzylic halides are efficient in producing organosamarium intermediates that react with several electrophiles including activated ketones and acid chlorides.
Scheme 24 Preparation of Bis(η5-cyclopentadienyl)samarium(II) from Samarium(II) Iodide and Sodium Cyclopentadienide[40]

Bis(η5-cyclopentadienyl)samarium(II) (8):[40]
A 0.1 M soln of SmI2 in THF (60 mL, 6 mmol) was slowly added to a stirring 0.4 M soln of sodium cyclopentadienide in THF (30 mL, 12 mmol) under an inert atmosphere. After stirring for 1 h, the precipitate was decanted and washed twice with THF to remove NaI. The Sm(Cp)2 is stable and can be stored for several days in a Schlenk tube.
2.12.17.1.5.2 Method 2: Synthesis of Bis(η5-pentamethylcyclopentadienyl)samarium(II)
In 1981, Evans and co-workers reported the synthesis of bis(η5-pentamethylcyclopentadienyl)samarium(II) in tetrahydrofuran.[41] This seminal report has had a profound impact on the use of samarium(II) reductants in a wide range of areas, including inorganic and organic synthesis, polymerization, and catalysis. The most straightforward synthesis of the reagent from samarium(II) iodide is illustrated in ▶ Scheme 25.[42]
Scheme 25 Preparation of Bis(η5-pentamethylcyclopentadienyl)samarium(II) from Samarium(II) Iodide and Potassium Pentamethylcyclopentadienide[42]

Bis(η5-pentamethylcyclopentadienyl)samarium(II) Bis(tetrahydrofuran) Adduct 9:[42]
K(Cp*) (5.43 g, 31.2 mmol) was added to a stirring soln of SmI2(THF)2 (7.78 g, 14.2 mmol) in THF (75 mL) in a 125-mL Erlenmeyer flask contained in an inert-atmosphere drybox. The soln rapidly turned purple and a white powder (KI) was formed. After stirring for 4 h, the THF was removed by rotary evaporation and toluene (100 mL) was added to the flask. The resulting soln containing potassium salts was stirred vigorously for 10 h, and then filtered. The solvent was removed by rotary evaporation to provide Sm(Cp*)2(THF)2; yield: 5.95 g (74%).
2.12.17.2 Use of Lewis Bases in Samarium(II)-Based Reactions
Lewis bases containing basic nitrogen and oxygen are often employed to accelerate reactions of samarium(II) iodide and other samarium(II)-based reductants. Typically, Lewis base additives act as ligands for samarium(II), accelerating the electron-transfer process by making the reagent a stronger reductant or by stabilizing the samarium(III) oxidation state.[43–45] More recently, it has been shown that these additives can also have an impact in the post-electron-transfer steps of reduction or in activating carbon—halide bonds. This portion of the review will focus on the most commonly utilized Lewis bases, and examples are presented where their presence is critical in important reductions and bond-forming reactions.
2.12.17.2.1 Hexamethylphosphoric Triamide
The most commonly utilized Lewis base in reactions of samarium(II) reductants is hexamethylphosphoric triamide. Despite its toxicity and suspected carcinogenicity, hexamethylphosphoric triamide remains the additive of choice in many samarium(II) iodide promoted reductions and bond-forming reactions. It not only exhibits unique behavior as a ligand for samarium(II), but it has synthetic advantages as well in that it significantly enhances the rate and stereochemical outcome of samarium(II)-mediated bond-forming reactions.[46,47] The examples in the following sections show the key classes of reductions and C—C bond-forming reactions initiated by samarium(II)/hexamethylphosphoric triamide. In some instances, proton-donor sources can be employed in place of hexamethylphosphoric triamide to carry out samarium(II) iodide mediated reductions. As a consequence, only substrate classes that require hexamethylphosphoric triamide are described below.
2.12.17.2.1.1 Method 1: Reduction of Alkyl and Aryl Halides
The potential of the combination of a samarium(II) reductant and hexamethylphosphoric triamide was first realized when Inanaga showed that a range of alkyl and aryl halides can be readily reduced by samarium(II) iodide when hexamethylphosphoric triamide is employed as a cosolvent.[16] It is important to note that this seminal report alerted the synthetic community to the importance of hexamethylphosphoric triamide and its use has led to the development of a large number of reactions. An important example shown in ▶ Scheme 26 is the reduction of an aryl halide in the presence of an ester.
Arenes, e.g. 10; General Procedure:[16]
CAUTION:
Hexamethylphosphoric triamide is a possible human carcinogen and an eye and skin irritant.
A 0.1 M soln of SmI2 in THF was prepared (2.5 equiv) containing HMPA (5% by volume). Next, an aryl bromide (1 equiv) was added to the soln under N2. The soln was stirred for 2 h at rt and conversion into the product was determined by GLC.
2.12.17.2.1.2 Method 2: Reduction of α-Oxygenated Carbonyl Compounds
Typically, α-oxygenated esters are difficult to reduce. Inanaga has shown that the combination of samarium(II) iodide and hexamethylphosphoric triamide efficiently reduces α-methoxy and α-acetoxy esters.[48] In these cases, the addition of a proton source (either methanol or ethanol) is critical for complete reduction. The reaction time for these systems varies from several minutes to several hours, with some representative examples shown in ▶ Scheme 27.
R1 | R2 | R3 | R4 | Proton Source | Time | Yield (%) | Ref |
(CH2)4Me | H | Me | Ac | MeOH | <5 min | >95 | [48] |
Ph | H | Me | Ac | MeOH | 1 min | 96 | [48] |
Me | Me | Et | Ac | EtOH | <5 min | >95 | [48] |
Me | Ph | Me | Me | MeOH | 12 h | 95 | [48] |
While the reduction of α-methoxy and α-acetoxy esters can be performed readily using samarium(II) iodide/hexamethylphosphoric triamide containing methanol, this procedure is not effective for the reduction of α-hydroxy esters. After screening various reagents, Inanaga has found that pivalic acid must be used in place of an alcohol to carry out such α-deoxygenations effectively.[48] An example is shown in ▶ Scheme 28.
Esters bearing a β-hydroxy group constitute important intermediates in organic synthesis. Inanaga and co-workers have found that α,β-epoxy esters can be selectively reduced with samarium(II) iodide/hexamethylphosphoric triamide to produce β-hydroxy esters, e.g. 13, in good yields (▶ Scheme 29).[49] A key feature of the method is the presence of 2-(dimethylamino)ethan-1-ol, which is essential to the success of the reaction. The reaction likely proceeds through electron transfer and cleavage of the α-carbon—oxygen bond. Related reduction of γ,δ-epoxy-α,β-unsaturated esters to produce δ-hydroxy-α,β-unsaturated esters can be carried out by a similar protocol.
Esters 11; General Procedure:[48]
CAUTION:
Hexamethylphosphoric triamide is a possible human carcinogen and an eye and skin irritant.
A soln of the ester (0.2 mmol), MeOH (0.24 mmol), and HMPA (0.3 mL) were combined in a flask and stirred under N2 at rt. To this was added a 0.1 M soln of SmI2 in THF (5–6 mL). The crude product was purified by column chromatography (silica gel, hexane/EtOAc 3:1).
Esters, e.g. 12; General Procedure:[48]
CAUTION:
Hexamethylphosphoric triamide is a possible human carcinogen and an eye and skin irritant.
A soln of pivalic acid (0.5 mmol) in THF (3 mL) was added dropwise to a mixture of ester (0.2 mmol), HMPA (0.3 mL), and 0.1 M SmI2 in THF (6 mL), and the mixture was then stirred under an inert atmosphere of N2 at rt. After stirring for 2 h, the reaction was quenched and the crude product was purified by column chromatography (silica gel, hexane/EtOAc 3:1).
β-Hydroxy Esters, e.g. 13; General Procedure:[49]
CAUTION:
Hexamethylphosphoric triamide is a possible human carcinogen and an eye and skin irritant.
The epoxy ester (0.2 mmol) was placed in a flask with HMPA (1 mmol), 2-(dimethylamino)ethanol (0.4 mmol), and a 0.1 M soln of SmI2 in THF (4.5 mL) and stirred under N2 at rt.
2.12.17.2.1.3 Method 3: Reduction of 4-Methylbenzoates
Markó and Lam have recently found that samarium(II) iodide/hexamethylphosphoric triamide can be employed to reduce tertiary, secondary, and primary 4-methylbenzoates under refluxing conditions, as shown in ▶ Scheme 30.[50] Primary 4-methylbenzoates provide lower yields than secondary or tertiary substituted esters. The reaction is remarkably selective, and can be carried out in the presence of a range of functionalities including acetates, amides, and unprotected alcohols. In addition, higher yields are obtained in tetrahydropyran in place of tetrahydrofuran.
Scheme 30 Reduction of Representative 4-Methylbenzoates by Samarium(II) Iodide/Hexamethylphosphoric Triamide[50]

R1 | Solvent | Yield (%) | Ref |
1-adamantyl | THF | 73 | [50] |
1-adamantyl | THP | 75 | [50] |
![]() | THF THP | 30 60 | [50] [50] |
![]() | THF THP | 62 71 | [50] [50] |
![]() | THF THP | 59 67 | [50] [50] |
(CH2)21Me | THP | 63 | [50] |
Alkanes 14; General Procedure:[50]
CAUTION:
Hexamethylphosphoric triamide is a possible human carcinogen and an eye and skin irritant.
HMPA (1.24 mL, 7.1 mmol, 12 equiv) was added to a 100-mL, flame-dried, three-necked flask equipped with a condenser and a magnetic stirrer, and containing a 0.1 M soln of SmI2 in THF or THP (17.8 mL, 1.8 mmol, 3 equiv), under an inert atmosphere of argon. The soln was heated to reflux, and then the 4-methylbenzoate (160 mg, 0.6 mmol) was added dissolved in a minimum of THF or THP. The reaction was monitored by TLC until completion. Then, the mixture was cooled and the reaction was quenched with sat. aq NH4Cl (10 mL). The aqueous layer was extracted with CH2Cl2 (3 × 10 mL) and the organic phases were combined, washed with sat. aq Na2CO3 (2 ×), and dried (Na2SO4). The solvent was removed under reduced pressure and the crude product was purified by column chromatography (silica gel, pentane).
2.12.17.2.1.4 Method 4: Grignard and Barbier Reactions
The Grignard reaction is an important classic reaction that is employed to couple an alkyl halide to a carbonyl. From a procedural standpoint, the reaction is a two-step process. First, a stable Grignard reagent (R1MgX) is formed through the reaction of magnesium metal with an alkyl halide in an ethereal solvent. Second, a carbonyl-containing substrate is added to the solution to produce a carbinol after workup. The Barbier reaction is similar, except that it is a one-step process where the magnesium, alkyl halide, and carbonyl are added simultaneously. Yields are sometimes affected by the change in mode of reagent addition.
When samarium(II) iodide/hexamethylphosphoric triamide is employed as the reductant, most reactions are carried out under Barbier conditions, where all components of the reaction are added together. A great deal of the early mechanistic work on samarium(II) iodide focused on this reaction. Under Barbier conditions, samarium(II) iodide can potentially reduce either the alkyl halide or carbonyl substrate.[51] The early mechanistic work of Curran[52,53] and later rate studies by Flowers[46,54,55] have shown that samarium(II) iodide/hexamethylphosphoric triamide preferentially reduces alkyl halides to organosamarium intermediates, which add to the carbonyl producing the final alcohol product after workup. In most cases, reactions are run under Barbier conditions since this approach succeeds in many cases where discrete organometallic additions are unsuccessful, especially in intramolecular reactions. If a Grignard approach is necessary, it is advised that other organometallics (organolithium or organomagnesium reagents) are attempted first. Nonetheless, Grignard conditions may be advantageous when an activated carbonyl is employed or when alkyl bromides or chlorides are used. When an activated carbonyl is employed, it can be reduced faster than the alkyl halide leading to pinacol side products, while alkyl bromides or chlorides are difficult to reduce through electron transfer from samarium(II) iodide, making carbonyl reduction a competitive process. Several representative examples of inter- and intramolecular coupling of alkyl halides with carbonyls by samarium(II) iodide/hexamethylphosphoric triamide are shown in the sections below.
2.12.17.2.1.4.1 Variation 1: Intermolecular Samarium Grignard Reactions
Curran and co-workers carried out a great deal of the early mechanistic studies designed to elucidate the mechanism of the samarium Barbier reaction. A series of reactions run under both Barbier and Grignard conditions were examined, and two examples of the latter approach are shown below in ▶ Scheme 31.[52] In all cases it is found that the product yields and stereoselectivity do not depend on the mode of reagent and substrate addition, which is consistent with the formation of an organosamarium intermediate under Barbier conditions.
R1 | R2 | dr | Yield (%) | Ref |
t-Bu | H | 62:38 | 81 | [52] |
H | Me | 91:9 | 88 | [52] |
Alkanols, e.g. 15; General Procedure:[52]
CAUTION:
Hexamethylphosphoric triamide is a possible human carcinogen and an eye and skin irritant.
The alkyl halide (0.5 mmol) in dry THF (1.5 mL) was added over 1–2 min to a 0.1 M soln of SmI2 in THF (11 mL) and HMPA (0.62 mL) under an inert atmosphere. After stirring for 5 min, the ketone was added and the reaction was stirred at rt for 30–40 min. Once complete, the reaction was quenched with sat. aq NH4Cl and extracted with pentane/Et2O (1:1). The organic extract was washed twice with H2O, followed by a wash with 3% aq Na2S2O3 and brine. The organic layer was dried (MgSO4), filtered, and concentrated. The crude product was purified by flash chromatography (hexanes/EtOAc 9:1).
2.12.17.2.1.4.2 Variation 2: Intermolecular Samarium Barbier Reactions
The classic work of Inanaga has shown that the addition of hexamethylphosphoric triamide in reactions of samarium(II) iodide significantly accelerates conversion of reactants into product. In follow-up studies, it is found that the use of samarium(II) iodide/hexamethylphosphoric triamide in the addition of β-, γ-, or δ-bromo esters to ketones and aldehydes under Barbier conditions provides good yields of lactones, as shown below in ▶ Scheme 32. Most reactions are complete within minutes in the presence of hexamethylphosphoric triamide. The major by-product of the reaction is the corresponding pinacol that forms through competitive carbonyl reduction by samarium(II) iodide/hexamethylphosphoric triamide.[16]
Scheme 32 Samarium(II) Iodide/Hexamethylphosphoric Triamide Barbier Reaction of Bromo Esters and Carbonyls[16]

R1 | R2 | R3 | n | Isolated Yield (%) | Ref |
CHMeBu | H | Me | 1 | 88 | [16] |
CHMeBu | H | Et | 2 | 54 | [16] |
CHMeBu | H | Et | 3 | 53 | [16] |
(CH2)11 | Me | 1 | 75 | [16] | |
(CH2)2Ph | Me | Et | 2 | 55 | [16] |
One of the advantages of using samarium(II) iodide/hexamethylphosphoric triamide is that the reagent combination can reduce substrates that are typically recalcitrant to reduction by samarium(II) iodide alone. Clayden and Julia have found that the combination of samarium(II) iodide and hexamethylphosphoric triamide successfully couples allylic sulfones to ketones and aldehydes under Barbier conditions to produce allylic alcohols 17 (▶ Table 1).[56] The reaction works well with aliphatic ketones and aldehydes, but aromatic aldehydes and ketones yield pinacol coupling derivatives as the major products. In addition, unsymmetrical sulfones give a mixture of homoallylic alcohol products resulting from inversion of the allylic system. One of the interesting features of this procedure is the inversion of the electrophilic character of the sulfone, enabling its use as a nucleophilic allylating agent.
Substrates | Product(s) | Yield (%) | Ref | |
Sulfone | Carbonyl | |||
![]() | ![]() | ![]() | 77 | [56] |
![]() | ![]() | ![]() | 87 | [56] |
![]() | ![]() | ![]() | 84 | [56] |
![]() | ![]() | ![]() | 62 | [56] |
![]() | ![]() | ![]() | 79 | [56] |
More recently, Takahashi and co-workers have reported a creative Barbier-type coupling that proceeds through the coupling of an alkyl bromide, an isocyanide, and an isocyanate.[57] The reaction is proposed to proceed via initial reduction of the alkyl bromide to an organosamarium(III) species, that first adds to an isocyanide followed by addition to an isocyanate to produce the final α-iminocarboxamide in good to excellent yield. The reaction works well with a range of alkyl bromides, aryl isocyanides, and aryl isocyanates. A few representative examples are shown in ▶ Scheme 33.
R1 | R2 | R3 | Yield (%) | Ref |
(CH2)2Ph | 2,6-Me2C6H3 | Ph | quant | [57] |
Et | 2,6-Me2C6H3 | Ph | 80 | [57] |
(CH2)3OTHP | 2,6-Me2C6H3 | Ph | 82 | [57] |
Lactones 16; General Procedure:[16]
CAUTION:
Hexamethylphosphoric triamide is a possible human carcinogen and an eye and skin irritant.
Reactions were carried out by stirring the carbonyl compound (0.1–1 mmol), bromo ester (0.2–2 mmol), and a 0.1 M soln of SmI2 in THF (2–20 mL), followed by addition of HMPA (0.1–1 mL) dissolved in THF (1–5 mL). The reaction was stirred at rt under N2. After the reaction was complete, it was quenched by the addition of 1 M aq HCl (0.1–1 mL) and silica gel (1–10 g) and stirred for 10 min before extraction and isolation of product.
Alcohols 17; General Procedure:[56]
CAUTION:
Hexamethylphosphoric triamide is a possible human carcinogen and an eye and skin irritant.
A soln containing the allylic sulfone (0.5 mmol), carbonyl substrate (1.0 mmol), and HMPA (1 mL) was degassed by repeated freeze–thaw cycles under reduced pressure. A 0.1 M soln of SmI2 in THF (20 mL) was added and the mixture was stirred under N2 until the purple color disappeared and a white precipitate formed. Once the reaction was complete, Et2O and 1% aq HCl were added to the flask. The layers were separated and the aqueous layer was extracted with Et2O (2 ×). The organic layers were combined and washed with sat. aq NaHCO3, dried (Na2SO4), and concentrated, and the residue was purified over silica gel.
α-Iminocarboxamides 18; General Procedure:[57]
CAUTION:
Hexamethylphosphoric triamide is a possible human carcinogen and an eye and skin irritant.
The alkyl bromide (3.0 equiv) and isocyanide (2.0 equiv) were added to a soln of SmI2(6.0 equiv) and HMPA (16.0 equiv) in THF at −15 °C. After stirring for 4 h at −15 °C, the isocyanate (1.0 equiv) was added to the mixture at −78 °C. After stirring for 5 min-12 h, the reaction was quenched with H2O and diluted with hexane, filtered with Florisil, and concentrated under reduced pressure. The residue was purified by TLC (Et2O/hexane 1:9).
2.12.17.2.1.4.3 Variation 3: Intramolecular Samarium Barbier Reactions
Matsuda and co-workers have recently employed an intramolecular samarium Barbier reaction in the synthesis of 10-isocyano-4-cadinene.[58] The Barbier reaction is used to form the trans-decalin framework, closing the ring in high yield, as shown in ▶ Scheme 34. This is the first example of the synthesis of 10-isocyano-4-cadinene with the correct natural absolute configuration.
(4R,4aS,8aS)-4-Isopropyl-4a,6,8a-trimethyl-1,2,3,4,4a,7,8,8a-octahydronaphthalen-1-ol (19):[58]
CAUTION:
Hexamethylphosphoric triamide is a possible human carcinogen and an eye and skin irritant.
A 0.1 M soln of SmI2 in THF/HMPA (55.5 mL, 5.55 mmol) was added to a soln of the alkyl iodide [crude product resulting from oxidation of the corresponding alcohol (1.85 mmol) to the aldehyde using Dess–Martin periodinane] in THF (19 mL) at rt under argon. The mixture was stirred for 30 min and then the reaction was quenched with sat. aq NaHCO3. The layers were separated and the aqueous layer was extracted with EtOAc. The organic layers were combined, washed with brine, and dried (Na2SO4). After filtering, the soln was concentrated under reduced pressure. The resulting residue was purified by column chromatography (silica gel, EtOAc/hexane 3:97); yield: 94%; dr 66:28.
2.12.17.2.1.5 Method 5: Reformatsky- and Aldol-Type Reactions
The Reformatsky reaction is traditionally carried out by coupling an α-halo carbonyl compound to a ketone or aldehyde employing zinc as a reductant. Samarium(II) iodide mediated versions have a number of advantages in intramolecular cyclizations. Usually, the reaction does not require hexamethylphosphoric triamide because most α-substituted esters are reduced readily to their corresponding enolates by samarium(II) iodide alone. After initial conversion into a samarium enolate, addition to an aldehyde or ketone provides a β-hydroxy ester. However, if α-chloro esters or related substrates are employed, hexamethylphosphoric triamide is necessary for efficient conversion into the product. During the past several years, the most important use of this reaction employing hexamethylphosphoric triamide has been the functionalization of α-chlorocyclopropane carboxylates.
Nishii and co-workers have developed an efficient samarium(II) iodide Reformatsky approach for the reductive coupling of α-chlorocyclopropane esters with aldehydes and ketones. The reaction proceeds to provide predominantly trans adducts in good to excellent yields.[59] The reaction works well for a range of substitution patterns. When aldehydes are employed, the reaction is run at −78 °C. A number of sample reactions employing symmetrical ketones are shown in ▶ Table 2. When unsymmetrical ketones are used, the major product is S configured.
Table 2 Samarium(II) Iodide Promoted Reformatsky-Type Reaction of 1-Chlorocyclopropane-1-carboxylates with Ketones[59]

Substrates | Product | Yield (%) | Ref | |
Cyclopropane | Ketone | |||
![]() | ![]() | ![]() | 77 | [59] |
![]() | ![]() | ![]() | 95 | [59] |
![]() | ![]() | ![]() | 90 | [59] |
![]() | ![]() | ![]() | 79 | [59] |
Alkyl 1-[Dialkyl(hydroxy)methyl]cyclopropane-1-carboxylates 20; General Procedure:[59]
CAUTION:
Hexamethylphosphoric triamide is a possible human carcinogen and an eye and skin irritant.
A soln of CH2I2 (115 μL, 1.42 mmol) in THF (14 mL) was added to Sm (286 mg, 1.90 mmol) at 0 °C under argon, followed by stirring at rt for 2 h. Next, HMPA (996 μL, 5.70 mmol) was added, and the mixture was stirred for 15 min. A soln of α-chlorocyclopropane ester (0.48 mmol) and ketone (0.57 mmol) in THF (1 mL) was added to the mixture, and it was stirred for a further 2 h. The reaction was quenched with sat. aq NH4Cl (10 mL). H2O was added and the soln was extracted with Et2O (5 × 20 mL). The organic phase was washed with H2O and brine, dried (Na2SO4), and concentrated. The crude product was purified by column chromatography (silica gel, hexane/EtOAc 7:1).
2.12.17.2.1.6 Method 6: Halide–Alkene Coupling Reactions
Although this type of coupling reaction can be achieved using hexamethylphosphoric triamide, work by Hilmersson has shown that a samarium(II) iodide/water/amine additive system successfully mediates these reactions as well, without potentially harmful additives.[60] Therefore, use of hexamethylphosphoric triamide for this type of reaction has received little attention in recent years and the reader may wish to refer to ▶ Section 2.12.17.3.2 for further details.
Curran has utilized hexamethylphosphoric triamide to cyclize an aryl radical onto a cyclobutene in the total synthesis of penitrem D (▶ Scheme 35).[61] A range of intramolecular radical cyclizations have been carried out in this fashion, producing yields ranging from 49 to 90%. It has also been found that the addition of acetone leads to coupling onto the cyclobutane, resulting in a tertiary alcohol side chain in the products.
(2aS*,8bR*)-2,2a,4,8b-Tetrahydro-1H-spiro[cyclobuta[a]naphthalene-3,2′-[1,3]dioxane] (21); Typical Procedure:[61]
CAUTION:
Hexamethylphosphoric triamide is a possible human carcinogen and an eye and skin irritant.
A soln of t-BuOH (31 mg, 0.42 mmol) and the cyclobutene (50 mg, 0.14 mmol) in THF (0.2 mL) was added to a soln of HMPA (0.35 g, 2.0 mmol) and 0.1 M SmI2 in THF (4.2 mL, 0.42 mmol) at 23 °C. After 50 min, air was bubbled through the mixture, and 0.1 M HCl (10 mL) was added. After extraction with Et2O (3 × 10 mL), the combined ethereal layers were dried (Na2SO4) and concentrated under reduced pressure. Purification by chromatography (silica gel, hexanes/EtOAc 10:1) provided (in order of elution) product 21 as a clear oil; yield: 23.6 mg (73%); and 2-benzyl-2-(cyclobut-2-enyl)-1,3-dioxane as a clear oil; yield: 5 mg (16%).
2.12.17.2.1.7 Method 7: Spirocyclization via Intramolecular Aryl Iodide Radical Addition
In a number of publications by Tanaka it has been shown that samarium(II) iodide, in combination with hexamethylphosphoric triamide and a proton donor, can be utilized to generate spirocycles. It has been found that the spirocyclization of N-(iodophenyl)-benzamides with electron-donating o-methyl or o-methoxy substituents provides high yields of product (▶ Scheme 36).[62]
Scheme 36 Spirocyclization of an N-(Iodophenyl)benzamide Using Samarium(II) Iodide/Hexamethylphosphoric Triamide[62]

1′-Methylspiro[cyclohexane-1,3′-indoline]-2,5-dien-2′-ones, e.g. 22; General Procedure:[62]
CAUTION:
Hexamethylphosphoric triamide is a possible human carcinogen and an eye and skin irritant.
A mixture of Sm (232 mg, 1.54 mmol) and 1,2-diiodoethane (335 mg, 1.19 mmol) in THF (12 mL) was stirred for 1.5 h. After cooling to 0 °C, HMPA (0.742 mL, 4.27 mmol) was added to the mixture, and stirring was continued for 20 min at this temperature. After cooling to −35 °C, a soln of the amide (83 mg, 0.237 mmol) and iPrOH (0.036 mL, 0.474 mmol) in THF (2 mL) was added to the mixture, and it was stirred for 15 min. After the mixture was exposed to air, sat. NaHCO3 was added, and the mixture was extracted with Et2O. The extract was washed with sat. NaHCO3 and brine and dried (MgSO4). The filtrate was concentrated under reduced pressure to leave a residue, which was purified by column chromatography (silica gel, hexane/EtOAc 6:1).
2.12.17.2.1.8 Method 8: Carbonyl–Alkene Coupling
2.12.17.2.1.8.1 Variation 1: Intramolecular Cyclization of Unactivated Alkenyl Ketones
The use of hexamethylphosphoric triamide in the intramolecular coupling of alkenes and carbonyls has led to an efficient cyclization pathway to synthesize both carbocycles and heterocycles with high diastereoselectivity. This is an especially common synthetic method for preparing cyclopentanoids with varying functionalities. Molander has demonstrated the importance of hexamethylphosphoric triamide in the ketone–alkene cyclization of unactivated alkenic ketones to produce five- and six-membered carbocycles.[63] ▶ Table 3 shows a few examples of cyclizations that can be achieved under these conditions.
Table 3 Examples of Intramolecular Ketone–Alkene Cyclization with Samarium(II) Iodide/Hexamethylphosphoric Triamide[63]

Substrate | Product | Isolated Yield (%) | dr | Ref |
![]() | ![]() | 91 | 36:1 | [63] |
![]() | ![]() | 90 | >150:1 | [63] |
![]() | ![]() | 89 | 6:1 | [63] |
Cycloalkanols 23; General Procedure:[63]
CAUTION:
Hexamethylphosphoric triamide is a possible human carcinogen and an eye and skin irritant.
Sm metal (0.30 g, 2.0 mmol) was added to an oven-dried, round-bottomed flask equipped with a magnetic stirrer bar and a septum under a flow of argon. The flask was gently flame-dried and then cooled under argon. To the Sm was added THF (13 mL) followed by CH2I2 (0.482 g, 1.8 mmol), and the mixture was allowed to stir at rt for 1.5 h. HMPA (2.51 mL, 14.5 mmol) was added and the soln was allowed to stir for 10 min.
A soln of the alkenyl ketone mixed with t-BuOH (3 equiv) in THF (0.05 M in substrate) was prepared and slowly added over 15 min at rt to the 0.15 M soln of SmI2/HMPA described above. Upon completion, the reaction was quenched with sat. aq NaHCO3 and the aqueous layer was extracted with Et2O. The combined organic layers were washed with H2O and brine, dried (MgSO4), and the solvent was removed under reduced pressure. Final purification involved filtering through a short column of Florisil to remove residual HMPA, and Kugelrohr distillation or flash chromatography.
2.12.17.2.1.8.2 Variation 2: Sequential Intramolecular Cyclization with Intermolecular Electrophilic Addition
Molander has shown that samarium(II) iodide promoted intramolecular ketone–alkene cyclization (▶ Section 2.12.17.2.1.8.1) can be expanded by introduction of an electrophile that undergoes addition to produce complex diastereoselective carbocycles 24.[63] The versatility of this approach is exemplified in ▶ Scheme 37.
Electrophile | R1 | E | Isolated Yield (%) | Ref |
cyclohexanone | H | ![]() | 80 | [63] |
PhCHO | H | CH(OH)Ph | 83a | [63] |
Ac2O | Ac | Ac | 85 | [63] |
CO2 | H | CO2H | 65 | [63] |
a dr 3:1. |
Carbocycles 24; General Procedure:[63]
CAUTION:
Hexamethylphosphoric triamide is a possible human carcinogen and an eye and skin irritant.
To a suspension of SmI2 (2.25 mmol) in THF (16 mL) was added HMPA (3.6 mL, 20.7 mmol). The resulting soln was allowed to stir for 5 min, followed by the addition of the ketone (0.98 mmol) in THF (20 mL) over 20 min. During this time a precipitate formed, which immediately went into soln upon addition of a soln of the electrophile (0.150 mmol) in THF (3 mL). After an additional 30 min, the reaction was quenched with sat. aq NaHCO3 and the aqueous layer was extracted with Et2O. The combined organic layers were washed with H2O and brine, dried (MgSO4), and the solvent was removed under reduced pressure. Final purification involved filtering through a short column of Florisil to remove residual HMPA, and Kugelrohr distillation or flash chromatography.
2.12.17.2.1.8.3 Variation 3: Intermolecular Ketone–Allene Coupling
The coupling of methoxyallene to ketyl radicals to provide 4-hydroxyalk-1-enyl ethers was reported in 2003 by Reissig.[64] Good to moderate yields of products from both cyclic (▶ Scheme 38) and acyclic ketones are obtained although acyclic ketones provide lower yields. The presence of hexamethylphosphoric triamide is reported to be necessary in order to increase the reducing power of samarium(II) iodide and promote the reaction.
n | Yield (%) | Ratio (E/Z) | Ref |
1 | 85 | 60:40 | [64] |
2 | 79 | 60:40 | [64] |
4-Hydroxyalk-1-enyl Methyl Ethers 25; General Procedure:[64]
CAUTION:
Hexamethylphosphoric triamide is a possible human carcinogen and an eye and skin irritant.
Sm (2.4–2.5 equiv) and 1,2-diiodoethane (2.2 equiv) were suspended in freshly distilled anhyd THF (10 mL per mmol Sm) under argon, and the mixture was stirred for 2 h at rt. To the resulting dark blue soln was added HMPA (18 equiv). The ketone (1.0 equiv), methoxyallene (2.0–3.0 equiv), and t-BuOH (2.0 equiv) were dissolved in anhyd THF (15 mL per mmol of ketone) and then added to the deep violet soln. After 16 h, the mixture was quenched with sat. aq NaHCO3 and H2O, the organic layer was separated, and the aqueous layer was extracted with Et2O (3 ×). The combined organic layers were washed once with H2O and twice with brine, dried (MgSO4), filtered, and concentrated. The resulting crude oil was purified by column chromatography [alumina (activity III), hexane/EtOAc].
2.12.17.2.1.8.4 Variation 4: Sequential Intramolecular Cyclization with Electrophilic Addition to 1H-Indole Derivatives
In another report, Reissig has illustrated the utility of the aforementioned ketone–alkene cyclization process in the synthesis of substituted 1H-indole derivatives, which are relevant to the synthesis of a variety of natural products.[65] In ▶ Scheme 39, it is shown that this method can be used to generate a substituted indolyl polycycle with three stereocenters with high selectivity depending on the proton donor. Reissig has also demonstrated that these molecules can undergo subsequent addition of an electrophile, similar to that described in ▶ Section 2.12.17.2.1.8.2.
Conditions | Combined Yield (%) of 26A and 26B | dr (26A/26B) | Ref |
HMPA (10.0 equiv), t-BuOH (10.0 equiv), rt | 88 | 95:5 | [65] |
HMPA (10.0 equiv), PhOH (10.0 equiv), rt | 50 | 90:10 | [65] |
HMPA (10.0 equiv), PhOH (10.0 equiv), 0 °C | 89 | 95:5 | [65] |
PhOH (10.0 equiv), rt | 80 | 60:40 | [65] |
t-BuOH (10.0 equiv), rt | 80 | 80:20 | [65] |
(9S*,9aR*,10R*)- and (9S*,9aR*,10S*)-9-Hydroxy-9-methyl-6-oxo-6,7,8,9,9a,10-hexahydropyrido[1,2-a]indole-10-carbonitrile (26A and 26B):[65]
CAUTION:
Hexamethylphosphoric triamide is a possible human carcinogen and an eye and skin irritant.
To a 0.1 M soln of SmI2 in THF (12 mL, 1.20 mmol) was added HMPA (0.9 mL, 5.00 mmol) under argon. In a second flask, the 1H-indole (120 mg, 0.50 mmol) was dissolved in THF (8 mL) and argon was bubbled through the soln for 10–20 min. The soln was added to the deep purple soln of SmI2 in THF/HMPA in one portion. After the soln color changed to yellow-green, in most cases after less than 1 min, the reaction was quenched with sat. aq NaHCO3 soln (30 mL), diluted with Et2O (25 mL), and extracted with Et2O (3 ×). The combined organic phases were washed with H2O and brine, dried (MgSO4), and concentrated. The crude mixture was purified by column chromatography (silica gel, hexane/EtOAc 3:1, then 1:1, then 1:3). In some cases additional purification by HPLC was necessary.
2.12.17.2.1.9 Method 9: Intramolecular Pinacol Coupling of Carbonyl Compounds
Tu and co-workers have employed hexamethylphosphoric triamide in a samarium(II) iodide promoted intramolecular coupling of a ketone and aldehyde moiety in a total synthesis of (+)-alopecuridine and (+)-sieboldine A (▶ Scheme 40). The use of hexamethylphosphoric triamide provides cis-diastereoselectivity in the resulting diol product.[66]
Scheme 40 Intramolecular Pinacol Coupling in a Synthesis of (+)-Alopecuridine and (+)-Sieboldine A[66]

4-(tert-Butoxycarbonyl)-13-(methoxymethoxy)-11-methyldodecahydro-1H-4λ4-indeno[1,7a-e]azonine-7a,8(8H)-diol (27):[66]
CAUTION:
Hexamethylphosphoric triamide is a possible human carcinogen and an eye and skin irritant.
In a round-bottomed flask, a stirred soln of the substrate (90 mg, 0.21 mmol) in THF (6 mL) was prepared under argon at 0 °C. HMPA (0.37 mL, 2.1 mmol, 10 equiv) was added followed by a 0.1 M soln of SmI2 in THF (6.3 mL, 0.63 mmol, 3 equiv). The mixture was stirred at 0 °C for 3 min. After quenching the reaction with sat. aq NH4Cl, the mixture was extracted with Et2O/EtOAc (1:1; 2 ×). The combined organic phases were washed with sat. aq NH4Cl and brine, dried (Na2SO4), and concentrated under reduced pressure. The residue was chromatographed (EtOAc/petroleum ether 1:2) to give a white solid; yield: 54 mg (60%).
2.12.17.2.1.10 Method 10: Intramolecular Pinacol-Type Coupling of Ketones and Imines
Although the use of hexamethylphosphoric triamide in pinacol coupling is limited (see ▶ Section 2.12.17.2.1.9), Wang has been able to carry out asymmetric intramolecular reductive coupling of ketones and imines to yield trans-1,2-amino alcohols with good selectivity (▶ Scheme 41).[67] Optimization of the reaction shows that stereoelectronic effects play a significant part in the reaction and that hexamethylphosphoric triamide is necessary for the coupling of alkyl, but not aryl, ketones. Yields are typically good for a variety of ketone appendages; some examples are highlighted in ▶ Scheme 41.
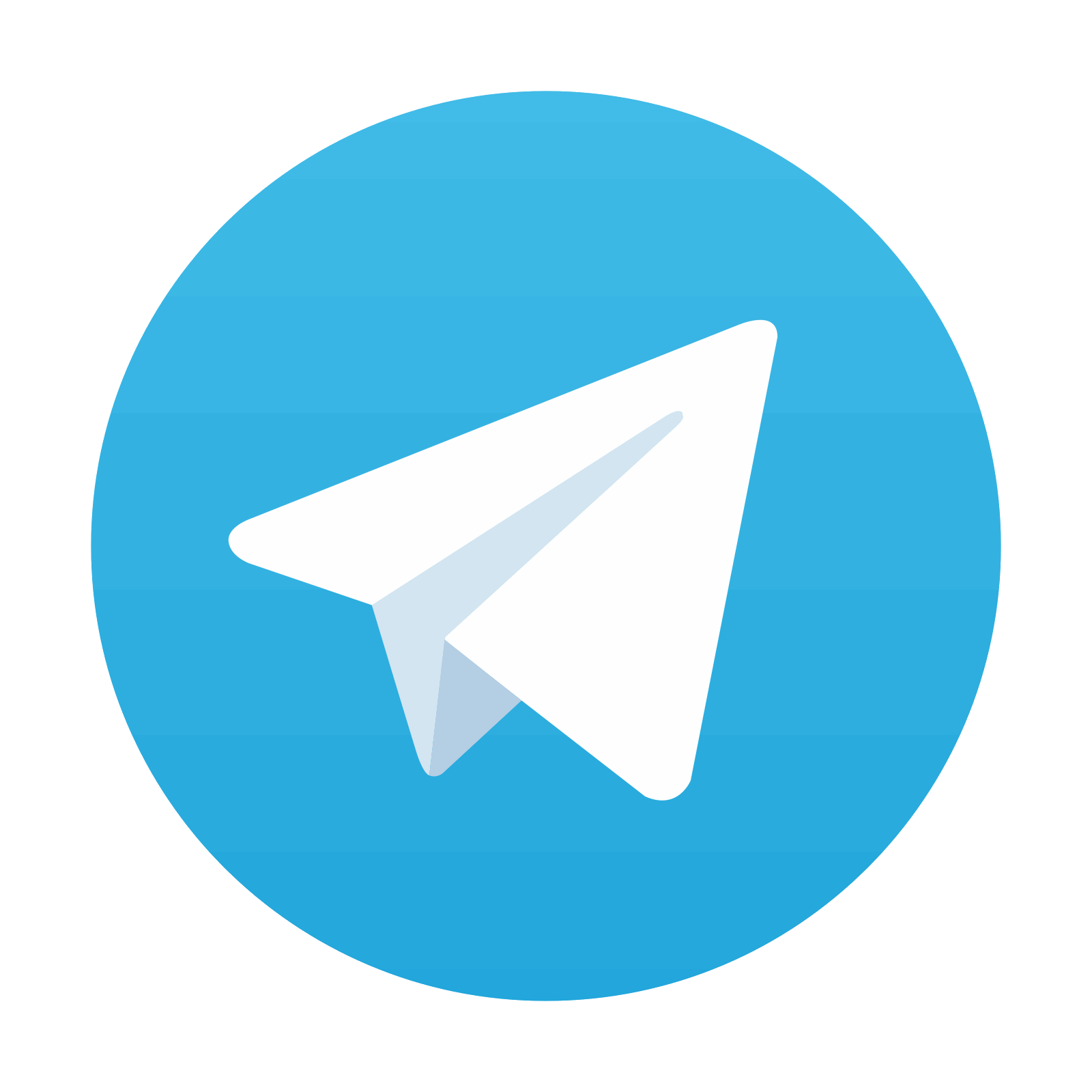
Stay updated, free articles. Join our Telegram channel

Full access? Get Clinical Tree
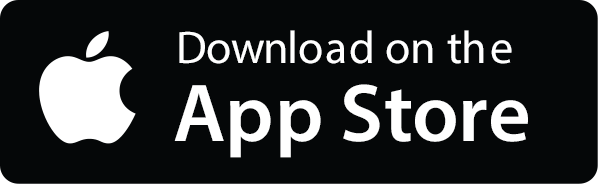
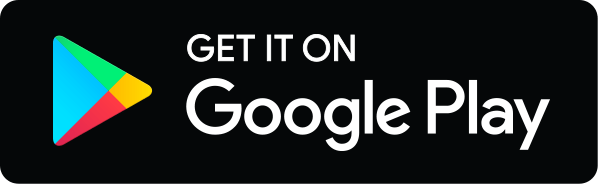