Introduction
Amphetamine (AMPH) and AMPH-type psychostimulants (e.g., methamphetamine, MDMA) are the second most widely abused illicit drug worldwide. Prescription psychostimulants like d -AMPH (Adderall) and methylphenidate (Ritalin) remain widely prescribed for disorders such as attention-deficit/hyperactivity disorder (ADHD) and narcolepsy. Because an increase in brain dopamine (DA) levels is the primary action mediating AMPH’s psychostimulant and abuse-related properties, the mechanisms by which AMPH alters brain DA neurotransmission have been studied extensively. Nevertheless, there remains no accepted or effective pharmacotherapy for AMPH abuse or addiction. Recent work demonstrating novel mechanisms by which AMPH alters DA neuron function suggests that the endocannabinoid (eCB) system represents an important target for controlling AMPH effects on DA neurons and a potential target for treating AMPH abuse and addiction.
Mesolimbic Dopamine
Addiction—or the state of engaging in compulsive behaviors despite adverse consequences—is believed to occur as a result of long-lasting changes in neural circuitry associated with rewards that influence cognition, motivation, and learning. Colloquially known as the “reward circuit,” the mesolimbic pathway consists of DA neurons projecting from the ventral tegmental area (VTA) to the nucleus accumbens (NAc). In the addictive state, these DA neurons exhibit increased activity specifically toward drug-related environmental triggers, with drugs of abuse engaging a variety of cellular processes. Numerous projections throughout the forebrain and midbrain nuclei modulate the activity of VTA DA neurons. Among these inputs, cells releasing glutamate (Glu), γ-aminobutyric acid (GABA), acetylcholine (ACh), and noradrenaline bind to DA neurons to modulate activity and subsequent DA release in the NAc.
Glutamatergic afferents from the prefrontal cortex (PFC), hypothalamus (HY), lateral habenula (LHb), and pedunculopontine (PPT), induce excitatory postsynaptic potentials through ionotropic N -methyl- d -aspartate (NMDA)/α-amino-3-hydroxy-5-methyl-4-isoxazolepropionic acid (AMPA) glutamate receptors. Subsequent depolarization opens voltage-gated Ca 2+ channels (VGCC), which triggers a conformational change in calmodulin (a calcium-binding secondary messenger that binds to intracellular proteins), thereby increasing cellular activity. Metabotropic group I Glu receptors (mGluR 1/5 ) activate phospholipase C (PLC), an enzyme that catalyzes phospholipids. In this case, phosphatidylinositol 4,5-bisphosphate (PIP 2 ) is cleaved into the secondary messengers inositol trisphosphate (IP 3 ) and 1,2 diacyl glycerol (DAG). IP 3 initiates transport of Ca 2+ from internal stores (e.g., endoplasmic reticulum) into the cytosol, wherein DAG is a precursor for 2-arachidonylglycerol (2-AG), the most prevalent eCB in the brain.
Endocannabinoid Modulation of DA
The eCB system is composed of transmembrane receptors, lipid signaling molecules, and proteins that synthesize or degrade these molecules. Following activation, PLC cleaves PIP 2 into DAG, which is then catalyzed by membrane-bound diacylglycerol lipase α (DGLα) into 2-AG. 2-AG is released “on demand,” meaning release is initiated following depolarization, wherein VGCCs allow influx of Ca 2+ , or following G-protein-induced IP 3 production, which Ca 2+ increases from IP 3 -induced depletion of internal stores. DA neurons release 2-AG in retrograde fashion onto presynaptic inputs, reducing GABA and glutamatergic inputs by binding to G-protein-coupled CB 1 receptors. CB 1 receptors are coupled to inhibitory G-proteins that decrease the duration of excitatory potentials by potentiating inwardly rectifying K + channels. 2-AG is then degraded by monoacylglycerol lipase (MAGL).
In this way, DA neurons release eCBs as retrograde messengers, modulating presynaptic inputs during periods of high activity by inhibiting GABAergic inputs (depolarization-induced suppression of inhibition) or inhibiting glutamatergic inputs (depolarization-induced suppression of excitation). In fact, this retrograde signaling mechanism is necessary for reward-related DA signaling in the mesolimbic pathway. CB 1 -mediated suppression of GABA inputs in particular is hypothesized to allow addictive drugs or reward-associated stimuli to disinhibit DA neurons projecting to the NAc. Because CB 1 receptor blockade concurrently reduces reward seeking and DA transients evoked by reward-predicting cues, diminished phasic DA signaling may underlie the suppression of drug reward, reinforcement, and relapse by CB 1 receptor antagonists. Thus eCB-mediated modulation of phasic DA signaling appears to be a common mechanism in reinforcement, and an attractive target for treating drug abuse and addiction.
GABAergic afferents local to the VTA and originating from midbrain and forebrain nuclei—namely the rostromedial tegmentum (RMTg), ventral pallidum (VP), HY, and NAc—inhibit DA neurons through GABAR activation, hyperpolarizing DA neurons through Cl – influx (GABA A R) and K + efflux (GABA B R). ACh released from brainstem nuclei—namely the pedunculopontine (or PPT) and laterodorsal tegmentum (LDT)—functions similarly to excitatory afferents, with depolarizing current flow through nicotinic ACh receptors (AChRs) and metabotropic AChR activation of PLC .
In contrast to other projections, noradrenaline (NA) afferents from locus coeruleus (LC) affect both DA neurons and presynaptic inputs. Metabotropic α 1 adrenergic receptors (ARs) located postsynaptically on DA neurons activate PLC, while α 2 ARs are found on presynaptic inputs and are coupled to inhibitory G-protein cascades. Given that AMPH functions on the NA reuptake transporter similarly to DAT, extended NA release can serve to further extend PLC activation in DA neurons.
With modulation from these various afferents, VTA neurons release DA in the NAc to drive reward-guided associative learning. How DA regulates the associative learning mechanisms that underlie drug-seeking behaviors depends on the temporal dynamics, location, and context of action potential-dependent DA release. DAergic signaling operates on multiple time-scales including very rapid events in vesicular DA release (phasic signaling) riding on top of a low, slowly changing DA tone (tonic signaling). Tonic signaling is thought to be predominantly maintained by low frequency cell firing, and may have an enabling influence on postsynaptic mechanisms. In contrast, phasic release is thought to occur when DA neurons respond to motivationally significant stimuli with a rapid burst of spikes. These signals are important for encoding temporally specific information such as associating cues with as well as approach behaviors directed at obtaining rewards. Critical temporal requirements also exist for DA signaling to promote synaptic plasticity associated with learning at the cellular level similar to the precise timing requirements for learning on the basis of reinforcement.
Phasic DA signaling also plays an intricate role in addiction. Several abused substances, such as cocaine, nicotine, ethanol, and opioids directly elevate phasic DA release. In addition, phasic release occurs in response to cues indicating drug availability are time-locked to the operant response for drug self-administration ; and facilitate reinstatement of an extinguished instrumental response for drug reward. Moreover, the well-known ability of abused substances to preferentially increase DA in the NAc shell may be directly attributable to a selective increase in phasic release events in this region.
Amphetamine
AMPH and its many derivatives are a diverse group of synthetic compounds originating from the naturally occurring substance phenethylamine. Modifications to the benzene ring or side chain of the phenethylamine compound produce a variety of powerfully active drugs such as AMPH, methamphetamine (METH), 3,4-methylenedioxy- N -methylamphetamine (“ecstasy”, or MDMA), and methylphenidate (MPH). AMPHs produce feelings of euphoria, relief from fatigue, improved performance on some simple tasks, increased activity levels, and anorexia. After marijuana, AMPHs are the most widely used illicit drugs worldwide, nearly equaling the number of cocaine and heroin users combined (25 million vs. 28 million). Nonetheless, the medical utility of AMPHs is unequivocal. AMPH’s psychostimulant effects are clinically indicated for treating narcolepsy, attention-deficit/hyperactivity disorder, obesity, and traumatic brain injury. The widespread use and abuse of this powerful compound necessitates a further understanding of the mechanisms by which the AMPHs affect cognition and behavior.
Amphetamine at Dopamine Terminals
AMPH’s reinforcing and behavioral properties arise from its ability to increase extracellular DA concentrations ([DA] EC ) at VTA terminals projecting to the striatum. Of particular relevance here, AMPH acts similar to other drugs of abuse and elicits relatively large increases in extracellular DA levels in the NAc. Drug-evoked alterations in DA neurotransmission are implicated in virtually all stages of addiction, from induction to maintenance and then to relapse after a period of abstinence. Addictive drugs are a unique type of reward in that they generally lack rewarding sensory properties, but are rather rewarding due to their direct interactions with brain physiology. By greatly elevating brain DA, drugs of abuse are thought to usurp normal reward learning mechanisms, which promotes their relatively powerful arousing and incentive-learning properties compared to natural rewards.
AMPH has traditionally been thought to elevate brain DA levels through interactions with the plasma membrane DA transporter (DAT), located on DA neuron terminals ( Fig. 10.1A ). The DAT tightly regulates the duration and strength of DAergic neurotransmission through high-affinity uptake of released DA, thus terminating DA action at pre- and postsynaptic sites. DAT functions by binding extracellular substrate along with Na + and Cl – , which promotes translocation of substrate and a conformational change in the DAT, exposing the binding site to the intracellular milieu. By acting as a substrate for DAT, AMPH competitively inhibits DA reuptake, increasing inward Na + transport, and also extending the duration of DA signals. This promotes a reversal of DAT conformation leading to an increase in the proportion of inward-facing DAT. Intracellular DA and Na + are then able to bind DAT, which promotes DAT-mediated reverse DA release (efflux) through a facilitated exchange-diffusion mechanism that also induces an extended duration of DA neurotransmission. A channel-like transporter mechanism and/or rapid mobilization of the DAT to and from the cell surface also appear to regulate AMPH’s effects on DA uptake and efflux.

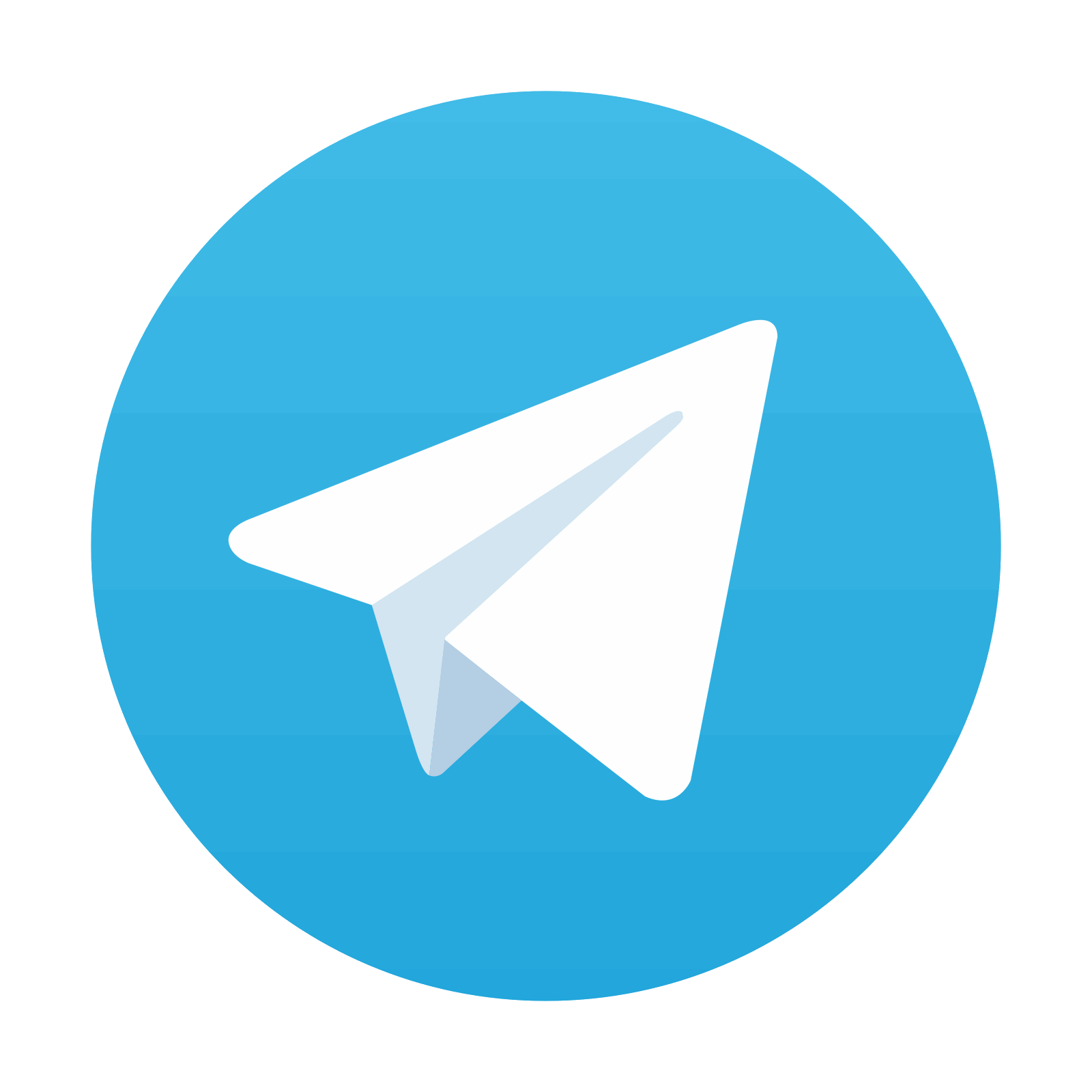
Stay updated, free articles. Join our Telegram channel

Full access? Get Clinical Tree
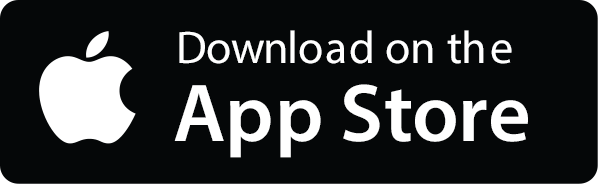
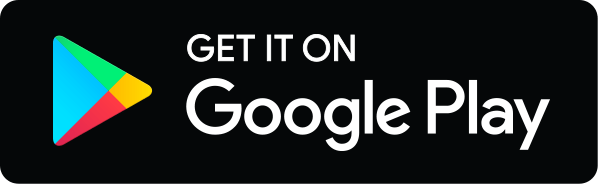
