The Respiratory System
The respiratory system is responsible for the exchange of oxygen and carbon dioxide between the air and the blood. Oxygen is required by all cells so that the life-sustaining energy source, adenosine triphosphate (ATP), can be produced. Carbon dioxide is produced by metabolically active cells and forms an acid that must be removed from the body. For gas exchange to be performed, the cardiovascular and respiratory systems must work together. The cardiovascular system is responsible for perfusion of blood through the lungs. The respiratory system performs two separate functions: ventilation and respiration.
● Physiologic Concepts
ALVEOLUS
The functional unit of the lungs is the alveolus (plural, alveoli). There are more than a million alveoli in each lung. Alveoli are small, air-filled sacs across which oxygen and carbon dioxide and other gases diffuse. The large number of small alveoli ensures that the total area available for the diffusion of gas in each lung is enormous. If the airflow into an alveolus is blocked, it collapses and is unavailable for gas exchange. If airflow into several alveoli is blocked, exchange of gases may be impaired to the extent that the person becomes hypoxic or unconscious or dies.
VENTILATION
The movement of air from the atmosphere into and out of the lungs is called ventilation. Ventilation occurs by bulk flow. Bulk flow is the movement of a gas or a fluid from high to low pressure.
Factors that Affect Ventilation
Ventilation is determined by the variables in the following equation:
F = P/R
where F is the bulk flow of air, P is the difference in pressure between the atmosphere and the alveoli, and R is the resistance offered by the conducting airways.
Pressure
Alveolar pressure varies with each inspiration and drives the flow of air. With the onset of inspiration, the thoracic cavity expands. As the thoracic cavity expands, the lungs also expand. According to the Boyle law, if the volume of an air-filled chamber increases, the pressure of the air in the chamber decreases. Therefore, as the lungs expand, pressure in the alveoli decreases to below atmospheric pressure, and air rushes into the lungs from the atmosphere (from high pressure to low pressure). At the end of inspiration, the thoracic cavity relaxes, causing pressures in the alveoli, which are filled with the air of inspiration, to be higher than in the atmosphere. Air then flows out of the lungs and down the pressure gradient.
Bronchial Resistance
Resistance of the airways is usually low. Resistance is increased when the smooth muscle of the bronchiolar tubes constricts. Constriction of the bronchi results in a decrease in airflow into the lungs. Resistance is inversely proportional to the radius of a vessel to the fourth power. This means that if the radius of a bronchiolar tube decreases by one-half, the resistance to airflow in that tube increases by 16 (i.e., 24). Therefore, when the air passages constrict even slightly, resistance to airflow goes up significantly.
Bronchiolar resistance is determined by parasympathetic and sympathetic nervous system innervation of the smooth muscle of the bronchi and local chemical mediators.
Parasympathetic nerves are carried to the bronchial smooth muscle by way of the vagus nerve and cause contraction or narrowing of the airways, increasing resistance and reducing airflow. Parasympathetic nerves release the neurotransmitter acetylcholine (ACh). ACh acts by binding to cholinergic receptors on the smooth muscle of the bronchi.
Sympathetic innervation of the bronchial smooth muscle occurs by way of nerve fibers from the upper thoracic and cervical ganglia and causes relaxation of the bronchi. This reduces resistance and increases airflow. Sympathetic
nerves release the neurotransmitter norepinephrine. Norepinephrine acts by binding to β2 adrenergic receptors on the smooth muscle of the bronchi.
nerves release the neurotransmitter norepinephrine. Norepinephrine acts by binding to β2 adrenergic receptors on the smooth muscle of the bronchi.
Nervous Control of Respiration
Ventilation is controlled by the respiratory center in the lower brainstem areas of the medulla and pons. In the medulla, there are inspiratory and expiratory neurons that fire at opposite times in a preset pattern of rate and rhythm. Respiratory neurons drive ventilation by exciting motor neurons that innervate the main muscle of inspiration (the diaphragm) and the accessory muscles (the intercostal muscles).
Central Chemoreceptors
Central chemoreceptors in the brain respond to changes in the hydrogen ion concentration of the cerebral spinal fluid. Increased hydrogen ion concentration increases the firing rate of the chemoreceptors, while decreased hydrogen ion concentration decreases the firing rate of the chemoreceptors. Information from the central chemoreceptors is delivered to the respiratory center in the brain, which in response increases or decreases the breathing pattern. Hydrogen ion concentration usually reflects carbon dioxide concentration. Therefore, when carbon dioxide levels rise, hydrogen ion levels rise, and the firing rate of inspiratory neurons is increased, causing an increase in respiratory rate. This is an example of negative feedback, because with an increase in the rate of breathing, the excess carbon dioxide and hydrogen ion will be blown off. With low carbon dioxide and low hydrogen ion levels, the firing rate of the inspiratory neurons returns toward baseline, and respiration slows.
Peripheral Chemoreceptors
Peripheral chemoreceptors exist in the carotid and the aortic arteries, and monitor oxygen concentration in arterial blood. These receptors, called the carotid and the aortic bodies, send their impulses to the respiratory center of the medulla and pons primarily to increase the rate of ventilation when oxygen is low. They are less sensitive than the central chemoreceptors. The peripheral chemoreceptors also respond with an increase in firing rate to increased hydrogen ion dissolved in the blood. This is important because under certain circumstances free hydrogen ion increases without causing a change in carbon dioxide concentration (e.g., during conditions of metabolic acidosis caused by prolonged diarrhea or diabetes mellitus). Free hydrogen ion is relatively impermeable across the blood-brain barrier, so it is unable to activate the central chemoreceptors directly.
Motor Neurons Driving Respiration
The major motor neuron controlling respiration is the phrenic nerve. When activated by the central inspiratory neurons, the phrenic nerve causes the diaphragm muscle to contract and the chest to expand. As the chest expands,
air begins to flow from the atmosphere into the lungs. Airflow into the lungs is called inspiration. As inspiration continues, firing of the central inspiratory neurons slows and firing of the expiratory neurons increases, causing cessation of motor neuron activity and relaxation of the diaphragm. Chest expansion reverses and air flows out of the lungs. Airflow out of the lungs is referred to as expiration and is a passive process.
air begins to flow from the atmosphere into the lungs. Airflow into the lungs is called inspiration. As inspiration continues, firing of the central inspiratory neurons slows and firing of the expiratory neurons increases, causing cessation of motor neuron activity and relaxation of the diaphragm. Chest expansion reverses and air flows out of the lungs. Airflow out of the lungs is referred to as expiration and is a passive process.
RESPIRATION
Respiration refers to the diffusion of gases between an alveolus and the capillary that perfuses it. Respiration occurs by diffusion, which involves the movement of a gas down its concentration gradient.
Factors that Affect Respiration
The rate of diffusion of a gas (e.g., oxygen and carbon dioxide) is determined with the following equation:
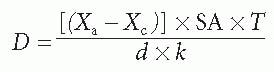
where D is the rate of diffusion, Xa is the concentration of gas in the alveolus, Xc is the concentration of gas in the capillary, SA is the surface area available for diffusion, T is the temperature of the solution, d is the distance across which diffusion must occur, and k is a physical constant that takes into account nonvariable characteristics of the gas such as its molecular weight and its specific solubility coefficient.
Concentration of Oxygen and Carbon Dioxide in the Alveolus and the Capillary
Alveolar oxygen concentration reflects atmospheric oxygen, whereas pulmonary capillary oxygen concentration reflects the oxygen concentration of systemic venous blood. Because systemic venous blood is blood returning from the peripheral circulation, where much of the oxygen has been used by the cells of the body, it has a low oxygen concentration. The atmosphere is typically well supplied with oxygen. Therefore, oxygen is normally in higher concentration in the alveolus than it is in the pulmonary capillary. Values for oxygen concentration are directly proportional to the partial pressure of the gas and are usually expressed in millimeters of mercury (mm Hg).
At sea level, the partial pressure of oxygen is approximately 100 mm Hg in the alveolus and 40 mm Hg in the pulmonary capillary. Because alveolar oxygen concentration in the alveolus is greater than in the capillary, oxygen diffuses down its concentration gradient from the alveolus into the capillary. This is how deoxygenated blood is replenished with oxygen by respiration.
Carbon dioxide normally diffuses in the opposite direction. It is in low concentration in the atmosphere and thus in low concentration in the alveolus
(40 mm Hg). Pulmonary capillary blood reflects systemic venous blood. Because carbon dioxide is a waste product of metabolizing cells, the concentration of carbon dioxide in the capillary is high (46 mm Hg). Therefore, in the lungs, carbon dioxide diffuses down its concentration gradient, from the blood into the alveolus, where it will be expired.
(40 mm Hg). Pulmonary capillary blood reflects systemic venous blood. Because carbon dioxide is a waste product of metabolizing cells, the concentration of carbon dioxide in the capillary is high (46 mm Hg). Therefore, in the lungs, carbon dioxide diffuses down its concentration gradient, from the blood into the alveolus, where it will be expired.
Under some circumstances, concentration gradients of oxygen and carbon dioxide between the blood and the alveolus may be increased or decreased; magnified concentration gradients affect the diffusion rate of the gas. For example, during exercise, oxygen concentration in the blood entering the pulmonary capillaries may be less than 40 mm Hg because the exercising muscles have increased their oxygen usage. Carbon dioxide concentration would be greater in blood flowing to the lungs from exercising tissue because its metabolic production would be increased. In this situation, diffusion rates for both gases would be increased, allowing more oxygen to diffuse into the blood and more carbon dioxide to diffuse out of the blood.
Surface Area
Surface area (SA) refers to the expanse of alveolar and capillary membranes available for gas diffusion. Surface area is normally high in the lungs. Some diseases, including emphysema, tuberculosis (TB), and lung cancer, can decrease the surface area available for diffusion, thus reducing the diffusion rates of oxygen and carbon dioxide.
Distance for Diffusion
The distance (d) across which oxygen and carbon dioxide must diffuse is normally quite small. Alveolar and capillary membranes are close to each other, separated only by a thin layer of interstitial fluid (Fig. 14-1).
Certain conditions, including pneumonia, can increase the distance of diffusion by causing edema and swelling of the interstitial space. This decreases the diffusion rate of the gases (Fig. 14-2). Interstitial fibrosis (scarring) can also
increase the distance between the alveoli and the capillaries and therefore slow diffusion.
increase the distance between the alveoli and the capillaries and therefore slow diffusion.
Temperature
A decrease in temperature (T) would decrease the diffusion rate of oxygen and carbon dioxide. An increase in T would increase the diffusion rate of both gases. An increase in temperature may play a role in meeting the increased metabolic demands during fever.
Permeability Coefficient
Carbon dioxide and, to a lesser extent, oxygen have high permeability. Because the variable k in the equation is fixed for each gas, k does not play an active role in determining respiration.
Oxygen Carrying in the Blood
Oxygen is carried in the blood in dissolved form and bound to hemoglobin. The amount of oxygen dissolved in the blood depends on the partial pressure of oxygen in the air entering the alveoli and the solubility of oxygen. Normally the dissolved amount carried is small (only approximately 3 mL/L). Instead, most oxygen (98%) is carried bound to hemoglobin.
Hemoglobin is a protein molecule composed of four subunits, each combining a globulin molecule with a molecule of iron. Each iron molecule has a binding site for oxygen. Dissolved oxygen combines with hemoglobin until all four sites are saturated. At normal arterial oxygen concentration of 100 mm Hg,
nearly 100% of hemoglobin molecules are saturated with oxygen. Even in the venous blood, with a reduced oxygen concentration of 40 mm Hg, hemoglobin is still at least 75% saturated with oxygen (Fig. 14-3). The ability of hemoglobin to bind oxygen is reduced by increased hydrogen ion concentration, increased temperature, and increased amount of a substance produced by red blood cells during glycolysis, 2,3-diphosphoglycerate (DPG). A reduced affinity for oxygen means that hemoglobin releases oxygen to the tissues more readily. Increases in hydrogen ion, temperature, and DPG occur during periods of increased metabolism; therefore, decreased hemoglobin affinity releases more oxygen to a cell and allows it to meet its elevated metabolic demands.
nearly 100% of hemoglobin molecules are saturated with oxygen. Even in the venous blood, with a reduced oxygen concentration of 40 mm Hg, hemoglobin is still at least 75% saturated with oxygen (Fig. 14-3). The ability of hemoglobin to bind oxygen is reduced by increased hydrogen ion concentration, increased temperature, and increased amount of a substance produced by red blood cells during glycolysis, 2,3-diphosphoglycerate (DPG). A reduced affinity for oxygen means that hemoglobin releases oxygen to the tissues more readily. Increases in hydrogen ion, temperature, and DPG occur during periods of increased metabolism; therefore, decreased hemoglobin affinity releases more oxygen to a cell and allows it to meet its elevated metabolic demands.
PERFUSION
For the respiratory system, perfusion refers to the movement of blood in the pulmonary vascular system, past the alveolar capillaries. Perfusion, like blood flow and ventilation, occurs by bulk flow. In the lungs, perfusion and ventilation usually are well matched. This ensures that there is adequate oxygen available in each alveolus to replenish the blood flowing past it and adequate blood flow to support each alveolus.
CIRCULATIONS THAT PROVIDE BLOOD FLOW TO THE LUNGS
Two separate blood circulations supply blood flow to the lungs from the heart: the pulmonary circulation and the bronchial circulation.
Pulmonary Circulation
The pulmonary circulation consists of deoxygenated blood traveling in the pulmonary artery from the right side of the heart. This blood perfuses the respiratory portions of the lungs and participates in the exchange of oxygen and carbon dioxide across the capillaries and alveoli. After picking up oxygen and releasing carbon dioxide, the blood returns to the heart by way of the pulmonary vein. Pressure and resistance to flow in the pulmonary circulation are usually low, with a mean pulmonary pressure of approximately 12 mm Hg compared with a mean systemic pressure of approximately 90 mm Hg. The pulmonary circulation is compliant and can accommodate large variations in blood volume. Therefore, the pulmonary circulation can act as a reservoir for blood that can be called upon in times of decreased systemic blood volume or pressure.
Bronchial Circulation
The bronchial circulation carries blood from the left side of the heart to the lungs through the thoracic aorta. The bronchial circulation accounts for approximately 8% to 9% of the total cardiac output. Blood in the bronchial circulation is well oxygenated and supplies oxygen to the structures of the lungs not involved in the exchange of gases, including the connective tissue and the large and small bronchi. Blood returns to the left side of the heart through the pulmonary vein. Returning bronchial blood is deoxygenated because it has been used by metabolically active cells of the lungs but has not been involved in gas exchange. The deoxygenated blood mixes with the well-oxygenated blood coming from the pulmonary circulation also back to the left side of the heart, and slightly reduces the overall oxygen concentration of that blood.
VENTILATION:PERFUSION RATIO
Ventilation refers to air moving into and out of the lungs. Perfusion is the blood passing through the pulmonary circulation to be oxygenated. The ventilation:perfusion ratio, V/Q, is the ratio of airflow into the lungs divided by the pulmonary blood flow. In this expression, V is the volume of air moved with each breath, expressed as milliliters per minute (mL/min), and Q is the rate of blood flow in the pulmonary circulation, also expressed as mL/min. Normally, perfusion is slightly greater than ventilation and the V/Q ratio is approximately 0.8. Therefore, the alveoli receiving oxygen are well perfused by blood, allowing optimal conditions for gas exchange.
ELASTICITY
Elasticity of the respiratory system refers to the degree to which the lungs resist inflation or stretching. The alveoli and other lung tissue normally resist stretching and recoil after the force causing the stretch or expansion is removed. This situation is partially caused by the surface tension of each alveolus and partially by the presence of elastic fibers throughout the lungs, which tend to recoil after stretch. Conditions such as emphysema reduce the elastic recoil of the lungs, resulting in chronic overinflation.
The reciprocal of elasticity of the lungs is termed lung compliance. Compliance refers to the ease of inflation or stretching of the lungs. Lung compliance is reduced by fibrosis, infection, or adult respiratory distress syndrome (ARDS).
PLEURAL PRESSURE
The lungs are surrounded by a thin membrane called the pleura. The outer layer of the pleural membrane is attached to the wall of the thoracic cavity. The inner layer of the pleura is attached to the lungs. With expansion of the thoracic cavity during inspiration, the outer layer is pulled out; this force is transmitted to the inner layer, which expands the lungs. In between the inner and outer layers of the pleura is the pleural space. This space is filled with a few milliliters of fluid that surround and lubricate the lungs. The pleural fluid is at negative pressure and opposes the elastic recoil (collapse) of the lungs. This negative pressure helps keep the lungs expanded.
SURFACE TENSION
Surface tension refers to the tendency of water molecules to pull toward each other and to collapse a sphere. Because each alveolus is lined with a thin water layer, the surface tension within each alveolus could be high, making it extremely difficult to expand an alveolus. With each breath, a certain pressure must be exerted to overcome the surface tension of the water layer. The amount of pressure needed to expand the alveolus is described by Laplace law in the following equation:
P = 2T/r
where P is the pressure needed to expand the alveolus, T is the surface tension of the water molecules, and r is the radius of the alveolus. As shown in this equation, the smaller the alveolus, the greater the pressure required to expand it. An inability to overcome the surface tension of an alveolus could lead to alveolar collapse. Normally, however, the surface tension of an alveolus is kept low by the presence of surfactant.
SURFACTANT
Certain cells inside the alveolus, called type II alveolar cells, produce an important substance called surfactant that helps reduce the surface tension of the alveolus, making it easier to inflate. Surfactant is a phospholipid that acts like a detergent to intersperse between water molecules in the alveolus, thereby weakening the bonds between them. This reduces surface tension and the tendency of the sphere to collapse.
When surfactant is present, a small alveolus actually requires less pressure to inflate than a large one because the surfactant is packed tightly together, greatly reducing the surface tension of the alveolus. This serves to compensate for the effect of small radius in Laplace law.
TESTS OF PULMONARY FUNCTIONING
Lung Volumes
Spirometry is the measurement of the volume of air moving into and out of the lungs and is measured as an individual inhales and exhales into a closed chamber. It is used to determine lung volumes, including tidal, inspiratory reserve, expiratory reserve, and residual volumes, and, calculated from these, vital capacity (Fig. 14-4). The average values presented in the figure for each of these volumes are for an adult male. Values for adult females are approximately 20% to 25% less.
Tidal Volume
The amount of air entering or leaving the lungs during a single breath is the tidal volume. The amount of air inspired at rest (inspiratory volume) usually equals the amount expired (expiratory volume). Tidal volume averages approximately 500 mL at rest.
Inspiratory Reserve Volume
The amount of air above the normal inspiration that can be maximally inspired with each breath is the inspiratory reserve volume. It averages approximately 3000 mL.
Expiratory Reserve Volume
The maximum amount of air that can be exhaled beyond normal exhalation is the expiratory reserve volume. This value averages approximately 1000 mL.
Residual Volume
The air remaining in the lungs after maximum exhalation is the residual volume. The normal value is approximately 1000 mL.
Vital Capacity
The maximum amount of air that an individual can inspire and expire during a single breath is the vital capacity. It is the sum of the normal tidal volume and the inspiratory reserve volume and the expiratory reserve volume. It is measured by having an individual take a maximum breath and then exhale as much as possible into the measurement chamber. In restrictive pulmonary disorders (e.g., resulting from neuromuscular disease, fibrosis, or loss of surfactant-producing cells), vital capacity is reduced.
A common test of pulmonary function is to plot the volume of air an individual can expire in the first second of expiration, called the forced expiratory volume in one second (FEV1). A healthy individual can expire approximately 80% of vital capacity as fast as possible in the first second (FEV1/vital capacity). In obstructive pulmonary diseases such as asthma and emphysema, expiration is particularly affected, and the amount of air an individual can forcefully expire in the first second is reduced. In patients who have restrictive airway disease, expiration is usually normal. Therefore, whereas overall vital capacity is reduced in those who have restrictive airway disease, FEV1 is normal.
Anatomic Dead Space
The amount of air in each breath that is measured as part of the tidal volume but does not actually participate in gas exchange is the anatomic dead space. This air fills the conducting passages of the nose, mouth, pharynx, larynx, trachea, bronchi, and the bronchioles. With rapid, shallow respirations, a greater percentage of each breath is wasted simply moving air in and out of the anatomic dead space compared with that seen with slow, deeper breathing.
Pulse Oximetry
Pulse oximetry is a noninvasive method that uses infrared technology to measure the percentage of hemoglobin that is saturated with oxygen. A transducer placed on an extremity detects light absorption by hemoglobin during pulsatile arterial waves and a computer translates it into a percentage. The normal range for oxygen saturation (SPO2) is 95% to 100%. Inaccurate readings may be caused by excessive movement, nail polish, and conditions that alter perfusion such as shock or hypovolemia.
Arterial Blood Gas
Arterial blood gases (ABGs) offer information about pH and the amount of oxygen, carbon dioxide, and bicarbonate in the blood. Normal values are:
• | pH | 7.35 to 7.45 |
• | PaO2 | 80 to 100 mm Hg |
• | PaCO2 | 35 to 45 mm Hg |
• | HCO3− | 22 to 26 mEq/L |
Capnography
Measurement of the amount of exhaled carbon dioxide may be done by treated paper which changes color in the presence of acid or via spectography. This test is useful in determining the effectiveness of ventilation and may actually help identify impending respiratory depression.
Radiographs
A chest x-ray offers information about structural abnormalities of the lung. For example, atelectasis, consolidation, densities, hyperinflation, edema, or collapsed areas offer valuable information for the diagnosis of respiratory problems.
● Pathophysiologic Concepts
ATELECTASIS
The collapse of either a lung or an alveolus is called atelectasis. Collapsed alveoli are airless, and therefore do not participate in gas exchange. This results in a reduction in the surface area available for diffusion, and respiration is decreased. Newborns may be born with alveoli collapsed at birth. This condition is called primary atelectasis. The collapse of previously expanded alveoli is called secondary atelectasis. The two main types of atelectasis are compression atelectasis and absorption atelectasis.
Compression Atelectasis
Compression atelectasis occurs when a source outside the alveolus exerts enough pressure on the alveolus to collapse it. This occurs if the chest wall is punctured or opened because atmospheric pressure is greater than the pressure holding the lungs expanded (pleural pressure), and with exposure to atmospheric pressure the lungs will collapse. Compression atelectasis can also occur if there is pressure exerted on the lungs or alveoli from a growing tumor, abdominal distention, or edema and swelling of the interstitial space around an alveolus.
Absorption Atelectasis
Absence of air in the alveolus results in absorption atelectasis. If flow of air into an alveolus is blocked, the air currently inside eventually diffuses out and the alveolus collapses. Blockage usually occurs after mucus buildup and obstruction of airflow through a bronchus supplying a given group of alveoli. Any situation that results in mucus accumulation, such as cystic fibrosis, pneumonia, or chronic bronchitis, increases the risk of absorption atelectasis. Surgery is also a risk factor for absorption atelectasis because of the mucus-producing effects of anesthesia as well as a resultant hesitancy to cough up accumulated mucus after surgery. This is especially true if the surgery was in the abdominal or thoracic area, where pain associated with coughing is intense. Prolonged bed rest after surgery increases the risk of developing absorption atelectasis, because lying down causes a pooling of mucus secretions in dependent areas of the lung, decreasing ventilation to those areas. Mucus accumulation increases the risk of pneumonia because mucus can act as a breeding ground for growth of microorganisms.
Absorption atelectasis can also be caused by anything that reduces the production or concentration of surfactant. Without surfactant, surface tension in the alveolus is high, increasing the likelihood of alveolar collapse. Premature birth is associated with a reduction in surfactant and a high incidence of
absorption atelectasis. Near drowning may dilute out surfactant and thus may be associated with absorption atelectasis as well.
absorption atelectasis. Near drowning may dilute out surfactant and thus may be associated with absorption atelectasis as well.
Damage to the type II alveolar cells that produce surfactant can also lead to absorption atelectasis. These cells are destroyed by the breakdown of the alveolar wall that occurs during some forms of respiratory disease, as well as by high oxygen therapy for a period longer than 24 hours. With loss of these cells, surfactant production is reduced.
HYPOXEMIA
The condition of reduced oxygen concentration in arterial blood is called hypoxemia. There are many causes of hypoxemia. Hypoxemia can occur if there is decreased oxygen in the air (hypoxia) or if hypoventilation occurs because of decreased lung compliance or atelectasis. Hypoxemia related to hypoperfusion (decreased blood flow past the alveoli) can occur from pulmonary hypertension, a pulmonary embolus, or a myocardial infarct. Hypoxemia may also occur if there is a problem with diffusion of oxygen across the alveolus into the capillary. This may happen with destruction of the alveolar-capillary interface or with edema of the alveolar-capillary interstitial space.
Because oxygen is carried in the red blood cell bound to hemoglobin, any decrease in hemoglobin concentration or carrying capacity can result in hypoxemia. Hemoglobin concentration is reduced in certain types of anemia. Binding sites for oxygen on the hemoglobin molecule may be occupied by other gases (e.g., carbon monoxide) that would also decrease the oxygen-carrying capacity of hemoglobin.
CYANOSIS
Bluish discoloration of the blood that occurs if large amounts of hemoglobin in the blood are not completely bound with oxygen molecules is called cyanosis. There are four sites on hemoglobin where oxygen may bind. Hemoglobin fully bound with oxygen at all four sites is called saturated. Desaturated or deoxygenated hemoglobin is not fully bound with oxygen.
If the hemoglobin concentration in the blood is normal, but the availability of oxygen to bind to hemoglobin is reduced, the hemoglobin molecules will be deoxygenated. Normally there is approximately 15 g of hemoglobin per 100 mL of blood. In arterial blood, more than 98%, or 14.7 g per l00 mL of blood, will be saturated with oxygen. Venous blood normally has an oxygen saturation of 75%; this comes to 11.3 g of hemoglobin per 100 mL of blood saturated with oxygen and about 4 g per 100 mL of unsaturated blood. If arterial hemoglobin oxygen saturation falls below 70%, resulting in 5 g or more of unsaturated hemoglobin per 100 mL of blood, cyanosis will be apparent.
In hypoxemia caused by low hemoglobin concentration, such as with microcytic hypochromic anemia, cyanosis will not develop because there will not be greater than 5 g of deoxygenated hemoglobin per 100 mL of blood. Cyanosis will
not occur with carbon monoxide poisoning because the hemoglobin-binding sites will still be saturated, although with the carbon monoxide molecule rather than oxygen. In both of these situations, hypoxemia would be present in the absence of cyanosis.
not occur with carbon monoxide poisoning because the hemoglobin-binding sites will still be saturated, although with the carbon monoxide molecule rather than oxygen. In both of these situations, hypoxemia would be present in the absence of cyanosis.
ALTERATIONS IN VENTILATION:PERFUSION RATIO
A decrease in ventilation may occur when the delivery of air to some alveoli is obstructed, for example, with mucus or by foreign-body aspiration. With decreased ventilation (V), the ventilation:perfusion ratio is decreased, because the blood flow (Q) will pass by underventilated alveoli. This mismatch in ventilation and perfusion is not beneficial for gas exchange, and is an example of a right-to-left shunt of blood. A right-to-left shunt, described in Chapter 13, is characterized by delivery of deoxygenated blood to the systemic circulation. A decrease in the ventilation:perfusion ratio, however, will not last long in the lungs because of the pulmonary arteriolar response when exposed to low oxygen.
Pulmonary arterioles vasoconstrict in response to low oxygen concentration in underventilated alveoli. This serves to decrease blood flow to those alveoli, returning the ventilation:perfusion ratio back toward 1.0. This response is called hypoxic vasoconstriction. Hypoxic vasoconstriction is effective only if the extent of underventilated alveoli is limited. In conditions such as chronic bronchitis, alveolar obstruction is so widespread that a normal ventilation:perfusion ratio cannot be maintained. Hypoxic vasoconstriction of the pulmonary arterioles can lead to pulmonary hypertension as described later.
Under some circumstances, it is possible for ventilation of an alveolus to be adequate but capillary perfusion to be compromised. The result is a decrease in Q and an increase in the ventilation:perfusion ratio. This situation could occur as a result of a pulmonary embolus. A myocardial infarct would also cause decreased perfusion of the alveoli.
PULMONARY HYPERTENSION
Elevated blood pressure in the pulmonary vascular system is called pulmonary hypertension. It is a common condition in serious respiratory or cardiovascular disease. It is a progressive condition characterized by elevated pulmonary artery pressure and increased pulmonary vascular resistance. Although a definitive diagnosis is based on findings from cardiac catheterization, echocardiography is a valuable way to assess for, and monitor the progression of pulmonary hypertension.
Causes of Pulmonary Hypertension
The pulmonary circulation is usually a low-pressure, low-resistance circulation. Anything that causes (1) a prolonged increase in pulmonary blood flow, (2) an increase in pulmonary resistance to flow, or (3) an impediment in pulmonary vascular outflow can result in pulmonary hypertension.
Increased Pulmonary Blood Flow
If excessive blood volume is delivered to the lungs, increased pulmonary blood flow occurs. For example, with a left-to-right shunt, blood from the left side of the heart goes back to the lungs rather than to the systemic circulation, thus overloading the lungs.
Increased Pulmonary Resistance to Flow
Anything that obstructs the passage of blood into or through the lungs causes increased pulmonary resistance to flow. This includes pulmonary fibrosis (scarring) and the changes in the structure of the lungs that accompany chronic obstructive pulmonary disease (COPD). Long-term pulmonary hypoxic vasoconstriction is also a significant cause of increased pulmonary resistance and hypertension. Hypoxic vasoconstriction of the pulmonary arterioles occurs when the pulmonary circulation is exposed to low oxygen, causing the vascular smooth muscle of the pulmonary arterioles to constrict. This can be useful because it allows the ventilation:perfusion ratio to return toward 1.0. However, if the condition is chronic or extensive, hypertrophy of the arterioles and increased pulmonary resistance can result.
Impediment to Outflow
This condition occurs with left-heart failure, as blood backs up in the left side of the heart, opposing continued flow out of the lungs. Other causes of impediment to outflow are mitral or aortic stenosis or incompetence, which also interfere with blood leaving the heart.
Consequences of Pulmonary Hypertension
Pulmonary hypertension can make it more difficult for the right side of the heart to pump. A type of heart failure called cor pulmonale can result. Cor pulmonale is right-sided heart failure caused by chronic lung disease. Pulmonary hypertension can also result in pulmonary edema because the capillary hydrostatic force favoring filtration is increased. Edema of the pulmonary interstitial space leads to a decreased diffusion rate of oxygen from the alveolus to the capillary because of increased distance for diffusion.
BRONCHIECTASIS
Bronchiectasis is abnormal dilation of a bronchus or bronchi. Bronchiectasis occurs from long-standing pulmonary obstruction of the lower airways by tumors, chronic infections, mucus accumulation as seen in cystic fibrosis, and exposure to toxins. The bronchi fill with mucus, resulting in atelectasis and development of abnormal connections between the bronchi. Ventilation of the alveoli is impaired.
COUGH
In 2006, the American College of Chest Physicians’ updated the guidelines for the diagnosis and management of cough and reclassified it as a symptom rather than a defense mechanism. As one of the most common reasons for visiting health care providers in the United States, chronic cough interferes with the quality of life (i.e., sleep deprivation, urinary incontinence). Based on the new guidelines, idiopathic cough is any unexplained cough, and cough due to postnasal drip is referred to as upper airway cough syndrome.
Irritating substances stimulate receptors in the tracheobronchial wall sending afferent impulses through the vagus nerve to the medullary center where the cough response is initiated. Allergens, postnasal drip, smoking, heart failure, esophageal reflux, and some medications, such as angiotensin converting enzyme (ACE) inhibitors, are possible stimulants that initiate the cough response.
CENTRAL NERVOUS SYSTEM DEPRESSION
Central nervous system depression is a depressed respiratory drive resulting from alteration in function of the respiratory centers of the brain. Central nervous system depression can occur with hypoxemia or chronic elevation of carbon dioxide concentration, both of which occur when there is decreased pulmonary ventilation or perfusion. The respiratory center of the medulla and pons, which normally drives respiration, requires adequate oxygenation to function. Although the normal stimulus to breathe is the carbon dioxide concentration of the cerebral spinal fluid (as reflected in hydrogen ion concentration), high carbon dioxide levels can depress the respiratory center enough to cause a cessation of breathing.
● Conditions of Disease or Injury
UPPER RESPIRATORY TRACT INFECTIONS
Infections caused by any microorganism of the non-gas-exchanging upper structures of the respiratory tract, including the nasal passages, the pharynx, and the larynx, are known as upper respiratory tract infections. Upper respiratory tract infections include the common cold, otitis media, sinusitis, pharyngitis or sore throat, laryngitis, and uncomplicated influenza. Most upper respiratory tract infections are caused by viruses, although bacteria may also be involved either initially or secondary to a viral infection. All types of infections activate the immune and the inflammatory responses, leading to swelling and edema of the infected tissue. The inflammatory reaction leads to increased mucus production, contributing to the symptoms seen with upper respiratory tract infections, including congestion, excess sputum, and nasal discharge. Headache, low-grade fever, and malaise may also occur as a result of the inflammatory reaction.
Respiratory Defenses against Infection
Although the upper respiratory tract is directly exposed to the environment, infections are uncommon and seldom progress to lower respiratory tract infections involving the lower airways and alveoli. Protective mechanisms abound throughout the respiratory tract to prevent infection. The cough reflex expels foreign bodies and microorganisms, and removes accumulated mucus. The mucociliary blanket consists of cells, located upwards from the level of the bronchi, which make mucus, and cilia cells that line the mucus-producing cells. The mucus-producing cells trap foreign particles, and the cilia beat rhythmically to propel the mucus and any trapped particles up the respiratory tree to the nasopharynx, where they can be expelled as sputum, blown out the nose, or swallowed. This complex is sometimes referred to as the mucociliary escalator system. The cilia are delicate structures that can be paralyzed or injured by a variety of noxious stimuli, including cigarette smoke, as described later.
If microorganisms evade these defense mechanisms and colonize the upper respiratory tract, a third important line of defense, the immune system, is in position to prevent their passage to the lower respiratory tract. This response is mediated by lymphocytes, but also involves other white blood cells such as macrophages and neutrophils brought to the area by the inflammatory process. If there is a breakdown of a defense mechanism of the respiratory system or if the microorganism is especially virulent, a lower respiratory tract infection can result.
Effects of Cigarette Smoking on Respiratory Defenses
Cigarette smoke is known to alter the effectiveness of some respiratory defense mechanisms. Products of cigarette smoke stimulate mucus production while paralyzing the cilia. This leads to the accumulation of thick mucus and any trapped particles or microorganisms in the airways, decreasing the movement of air and increasing the risk of microbial growth. A smoker’s cough is an attempt to expel this thick mucus out of the respiratory tract. Lower respiratory tract infections are more common in smokers and in those exposed to second-hand smoke, especially infants and children.
Clinical Manifestations
Clinical indications of upper respiratory tract infections depend on the infection site as well as the microorganism responsible for the infection. All clinical manifestations result from the inflammatory processes and any direct damage the microorganism inflicts. Clinical manifestations include:
Diagnostic Tools
A good history and physical examination will assist diagnosis.
Complete blood cell count and sputum culture to rule out bacterial infection.
Complications
Sinusitis and acute otitis media may develop.
Lower respiratory tract infections, including pneumonia and bronchitis, may follow an upper respiratory infection.
Treatment
Rest to reduce the body’s metabolic demands.
Extra hydration helps liquefy the thick mucus, making it easier to move it out of the respiratory tract. This is important because mucus accumulation offers a breeding ground for secondary bacterial infection.
Decongestants, antihistamines, and cough suppressants may provide some symptom relief.
Some studies suggest zinc lozenges or increased vitamin C consumption may reduce the severity or likelihood of certain viral infections.
Antibiotics are required if the infection is bacterial either initially or secondary to a viral infection.
LOWER RESPIRATORY TRACT INFECTIONS
Pneumonia
Pneumonia, an acute infection of the lung tissue by a microorganism, is a lower respiratory tract infection. Most pneumonias are bacterial in origin, occurring as a primary condition or secondary to a previous viral infection. Pneumonia is classified as community acquired (CAP) when it occurs outside a health care facility or in someone other than a health care provider. It is more prevalent in smokers and has a much higher incidence in the winter months. Pneumonia diagnosed at least 48 hours after being admitted to a health care facility is referred to as hospital-acquired (or nosocomial) pneumonia and has a mortality rate of up to 50%. Almost 90% is bacterial in nature.
The most common cause of bacterial pneumonia is the gram-positive bacterium Streptococcus pneumoniae, which is responsible for pneumococcal pneumonia. The bacteria Staphylococcus aureus and group A beta-hemolytic streptococci are also frequent causes of pneumonia, as is Pseudomonas aeruginosa. Other pneumonias are caused directly by viruses, such as that seen occasionally with influenza. Young children especially are susceptible to viral pneumonia, usually from infection with respiratory syncytial virus (RSV), parainfluenza, adenovirus, or rhinovirus. Mycoplasmal pneumonia, a relatively common pneumonia, is caused by a microorganism that is, in some respects, between a virus and a bacterium. Individuals who have acquired immunodeficiency syndrome (AIDS) frequently develop an otherwise rare pneumonia called Pneumocystis carinii pneumonia. Individuals exposed to aerosols of previously standing water, for instance, from air-conditioning units or dirty humidifiers, may develop Legionella pneumonia. Finally, individuals who aspirate stomach contents after vomiting or who aspirate water in an experience of near-drowning may develop aspiration pneumonia. For these individuals, the aspirated material itself rather than a microorganism may cause pneumonia by stimulating an inflammatory reaction. Subsequent bacterial infection may also develop.
The risks of developing the pneumonias described above are greater for the young, the old, or for anyone immunocompromised or weakened by another disease or disability. Risk of death after pneumonia is also stratified based on age (over 50 or young, especially the newborn) and the presence of coexisting illness such as congestive heart failure, neoplastic disease, or renal disease.
Much of the damage to the lung tissue after successful colonization of the lungs by a microorganism is the result of the usually vigorous immune and inflammatory reaction mounted by the host. In addition, toxins released by the bacteria causing bacterial pneumonia can directly damage cells of the lower respiratory system, including the surfactant-producing type II alveolar cells. Bacterial pneumonia results in the most striking immune and inflammatory response, the course of which has been well described for pneumococcal pneumonia.
Stages of Bacterial Pneumonia
For pneumococcal pneumonia, four stages of disease have been described. These four stages are similar for the other types of pneumonia.
Stage 1, called hyperemia, refers to the initial inflammatory response occurring in the area of lung infection. It is characterized by increased blood flow and increased capillary permeability at the site of infection. It occurs as a result of inflammatory mediators released from mast cells after immune cell activation and tissue injury. These components include histamine and prostaglandin. Mast cell degranulation also activates the complement pathway. Complement acts with histamine and prostaglandin to vasodilate the pulmonary vascular smooth muscle, leading to increased blood flow to the area and increased
capillary permeability. This results in movement of plasma exudate into the interstitial space, causing swelling and edema between the capillary and the alveolus. Fluid buildup between the capillary and the alveolus increases the distance over which oxygen and carbon dioxide must diffuse, thereby decreasing the rate of gas diffusion. Because oxygen is less soluble than carbon dioxide, its movement into the blood is most affected, often leading to a decrease in hemoglobin oxygen saturation. During this first stage of pneumonia, infection spreads to neighboring tissue as a result of increased blood flow and breakdown of neighboring alveolar and capillary membranes as the inflammatory processes continue.
capillary permeability. This results in movement of plasma exudate into the interstitial space, causing swelling and edema between the capillary and the alveolus. Fluid buildup between the capillary and the alveolus increases the distance over which oxygen and carbon dioxide must diffuse, thereby decreasing the rate of gas diffusion. Because oxygen is less soluble than carbon dioxide, its movement into the blood is most affected, often leading to a decrease in hemoglobin oxygen saturation. During this first stage of pneumonia, infection spreads to neighboring tissue as a result of increased blood flow and breakdown of neighboring alveolar and capillary membranes as the inflammatory processes continue.
Stage 2 is called red hepatization. It occurs when the alveoli fill with red blood cells, exudate, and fibrin, produced by the host as part of the inflammatory reaction.
Stage 3, called gray hepatization, occurs as white blood cells colonize the infected part of the lung. Then, fibrin deposits accumulate throughout the area of injury and phagocytosis of cell debris occurs.
Stage 4, called the resolution stage, occurs when the inflammatory and immune responses wane; cell debris, fibrin, and bacteria are digested; and macrophages, the cleanup cells of the inflammatory reaction, dominate.
Clinical Manifestations
Symptoms are similar for all types of pneumonia but are usually most pronounced for those of bacterial origin.
Significantly increased respiratory rate. Normal and abnormal respiratory rates vary with age, with young infants and children having more rapid normal rates of breathing than older children and adults.
Grunting, intercostal and substernal retractions, and nasal flaring may be experienced by infants in an attempt to improve airflow.
Fever and chills from the inflammatory processes and a cough that is often productive, purulent, and present throughout the day.
Chest pain as a result of pleural irritation. The pain may be diffuse or referred to the abdominal area.
Sputum that is rust colored (for S. pneumoniae), pink (for S. aureus), or greenish with a particular odor (for P. aeruginosa).Stay updated, free articles. Join our Telegram channel
Full access? Get Clinical Tree
