from the small intestine. The stimulus for the release of CCK is the presence of a mixture of food particles entering the duodenum from the stomach. This food mixture coming from the stomach is called chyme. The pancreatic enzymes are secreted as inactive proenzymes that are activated when they reach the duodenum. The activated enzymes include:
trypsin, which is responsible for the digestion of proteins to amino acids
amylase, which is responsible for the digestion of carbohydrates to simple sugars
lipase, which is responsible for the digestion of fats to free fatty acids and monoglycerides
insulin serves to provide glucose to our cells, build protein, and maintain low plasma glucose levels.
that occurs during fasting periods in between meals. Its functions are mainly catabolic (breaking down). In most respects, the functions of glucagon are opposite to that of insulin. For example, glucagon acts as an insulin antagonist by inhibiting glucose movement into cells. Glucagon also stimulates liver gluconeogenesis and causes the breakdown of stored glycogen to be used as an energy source instead of glucose. Glucagon stimulates the breakdown of fats and the release of free fatty acids into the bloodstream so they may be used as an energy source instead of glucose. These functions serve to increase blood glucose levels. The release of glucagon by the pancreas is stimulated by sympathetic nerves.
TABLE 16-1 Counter-Regulatory Hormones | ||||||||
---|---|---|---|---|---|---|---|---|
|
Body mass index (BMI) ≥ 25 kg/m2 in adults
Sedentary lifestyle
Blood pressure ≥ 140/90 mm Hg or currently taking antihypertensives
First-degree relative with diabetes
HDL < 36 mg/dL or triglycerides > 250 mg/dL
Females with a history of polycystic ovarian syndrome, giving birth to an infant > 9 lb, or gestational diabetes mellitus
Previous impaired fasting glucose (IFG) or impaired glucose tolerance (IGT) tests
Clinical findings consistent with insulin resistance such as Acanthosis nigricans or skin tags
African-American, American Indian, Asian American, Pacific Islander, or Hispanic
History of cardiovascular disease or other factors specific to cardiovascular risk
TABLE 16-2 Diabetes Mellitus: A Classification Scheme | ||||||||||||||||||||||||
---|---|---|---|---|---|---|---|---|---|---|---|---|---|---|---|---|---|---|---|---|---|---|---|---|
|
1 diabetes are part of the histocompatibility complex genes (see Chapter 4). The histocompatibility complex controls the recognition of self-antigens by the immune system; loss of self-tolerance is core to developing autoantibodies. The histocompatibility genes are primarily coded on chromosome 6. Another specific insulin-related gene on chromosome 11 has been implicated in the development of type 1 diabetes through its effects on beta cell development and replication. Siblings of individuals who have type 1 diabetes and children of a parent who has type 1 diabetes have an increased risk of developing the disease compared with those without an affected first-degree relative. In clinical studies, nonsymptomatic siblings show a higher incidence (2% to 4%) of antibodies against pancreatic beta cells compared to those who do not have a first-degree relative with diabetes; the earlier the onset of antibodies and the higher the level, the greater the likelihood of those siblings developing the disease later in life.
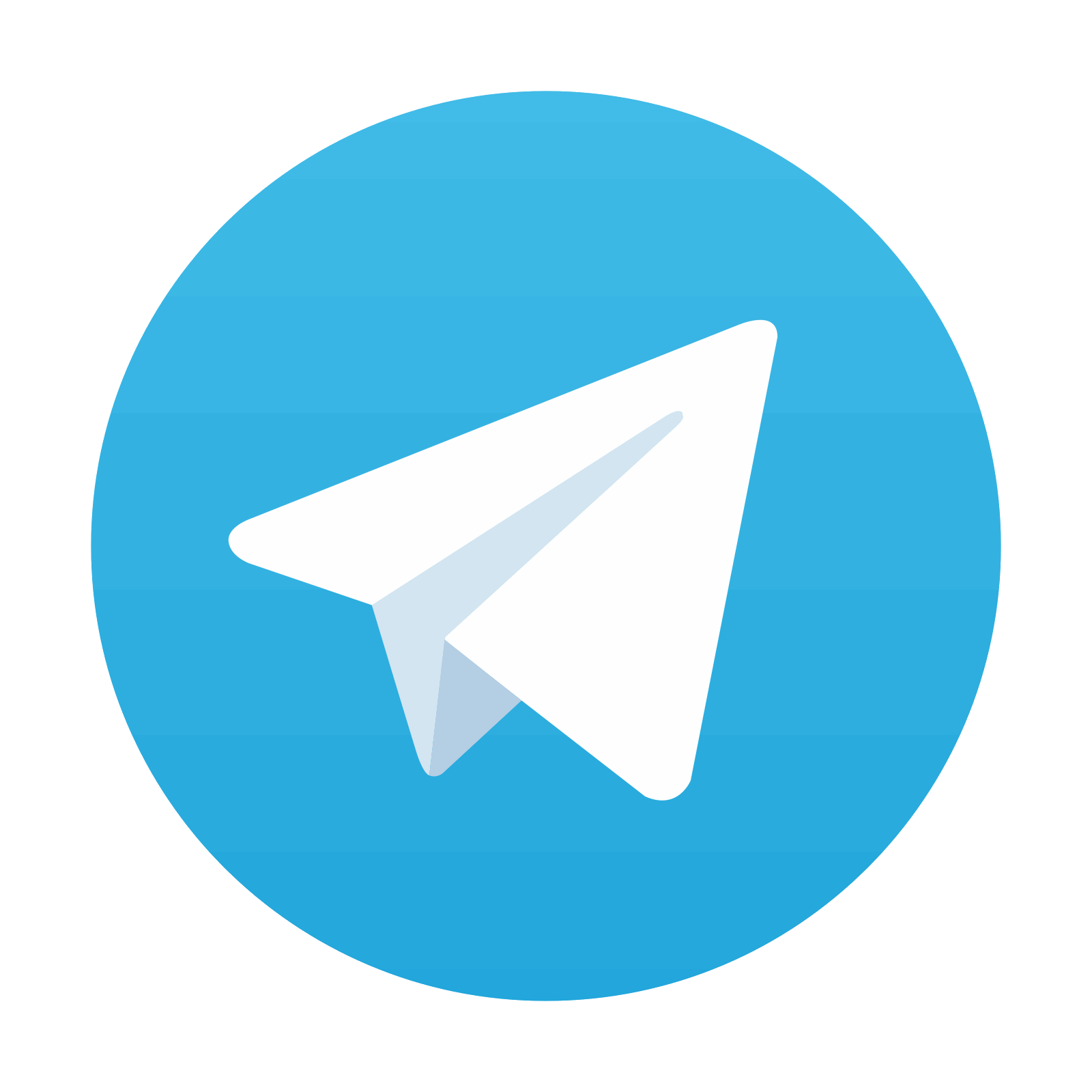
Stay updated, free articles. Join our Telegram channel

Full access? Get Clinical Tree
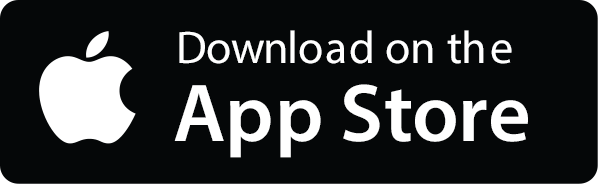
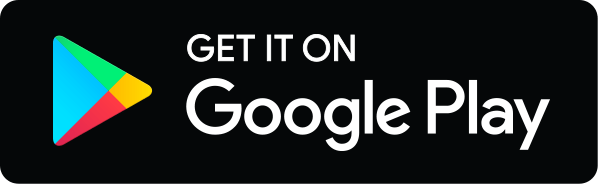
