Chapter 23 The Metabolism of Fatty Acids and Triglycerides
Fatty acids differ in their chain length and number of double bonds
In saturated fatty acids, the carbons are linked exclusively by single bonds. Of the fatty acids listed in Table 23.1, acetic acid does not occur in natural fats and oils, but vinegar contains about 5% of free (unesterified) acetic acid. Butyric acid is also rare in natural fats except milk fat. It is notorious for its smell, which resembles that of malodorous feet. In the production of some types of cheese, butyric acid is released from milk fat by the action of microbial lipases and contributes to the flavor of the product. Myristic acid is abundant in nutmeg, coconut, and palm kernel oil. Palmitic acid and stearic acid are the most common saturated fatty acids in animal fat, accounting for 30% to 40% of the fatty acids in human adipose tissue.
The latter designation is useful because fatty acids can be elongated and shortened only at the carboxyl end. For example, if oleic acid (Table 23.2) is elongated by two carbons at the carboxyl end, the product is no longer a Δ9 fatty acid but Δ11, but it is still an ω9 fatty acid. Humans cannot introduce new double bonds beyond Δ9. Therefore some of the polyunsaturated fatty acids, notably linoleic acid and possibly α-linolenic acid, are nutritionally essential. The structures of unsaturated fatty acids can be described by a formula indicating chain length, number of double bonds, and locations of the double bonds (see Table 23.2).
Chylomicrons transport triglycerides from the intestine to other tissues
The main products of fat digestion are 2-monoacylglycerol and free fatty acids (see Chapter 19). After their absorption, the fatty acids are activated to acyl–coenzyme A (acyl-CoA) in the endoplasmic reticulum (ER) of the intestinal mucosal cell:
The synthesis of acyl-CoA is made irreversible by hydrolysis of the inorganic pyrophosphate that is formed in the reaction. The acyl-CoA then reacts with 2-monoacylglycerol to form triglyceride (Fig. 23.1).
Adipose tissue is specialized for the storage of triglycerides
The glycerol of the triglycerides is derived from glycerol-3-phosphate. Adipose tissue has low levels of glycerol kinase. Therefore most glycerol phosphate is made not from free glycerol but from the glycolytic intermediate dihydroxyacetone phosphate. The NADH-dependent glycerol phosphate dehydrogenase that catalyzes this reaction is the same enzyme that participates in the glycerol phosphate shuttle (see Chapter 21) and in gluconeogenesis from glycerol (see Chapter 22). Dihydroxyacetone phosphate is derived from glucose after a carbohydrate meal or from lactate during fasting and after a high-fat, low-carbohydrate meal (Fig. 23.2).
Fat metabolism in adipose tissue is under hormonal control
Norepinephrine (noradrenaline) from sympathetic nerve terminals and epinephrine (adrenaline) from the adrenal medulla are released during physical exercise and stress. They stimulate lipolysis through β-adrenergic receptors and cyclic AMP (cAMP). Figure 23.3 shows the mechanism for stimulation of the hormone-sensitive diglyceride lipase by the catecholamines and other cAMP-elevating hormones. The catecholamines also stimulate the adipose tissue triglyceride lipase by unknown mechanisms.
Insulin increases the uptake of glucose into the cell by the GLUT4 transporter. The metabolism of glucose stimulates fat synthesis by providing the precursor glycerol phosphate. In addition, insulin stimulates LPL in the capillaries of adipose tissue and induces glycerol phosphate-acyltransferase, the enzyme that adds the first fatty acid to glycerol phosphate in the biosynthetic pathway (see Fig. 23.2).
Fatty acids are transported into the mitochondrion
Acyl-carnitine crosses the membrane in exchange for free carnitine. In the mitochondrial matrix, the fatty acid is transferred back to CoA. The reversible enzymatic reactions are catalyzed by two carnitine-acyltransferases (Fig. 23.4).
β-Oxidation produces acetyl-coa, nadh, and fadh2
The major pathway of fatty acid oxidation is called β-oxidation because it oxidizes the β-carbon (carbon 3). Eventually, it slices the whole fatty acid into two-carbon fragments in the form of acetyl-CoA. Each cycle of β-oxidation produces NADH and the reduced form of flavin adenine dinucleotide (FADH2) in addition to acetyl-CoA (Fig. 23.5). These reduced coenzymes feed into the respiratory chain.
The stoichiometry for β-oxidation of palmitate is as follows:
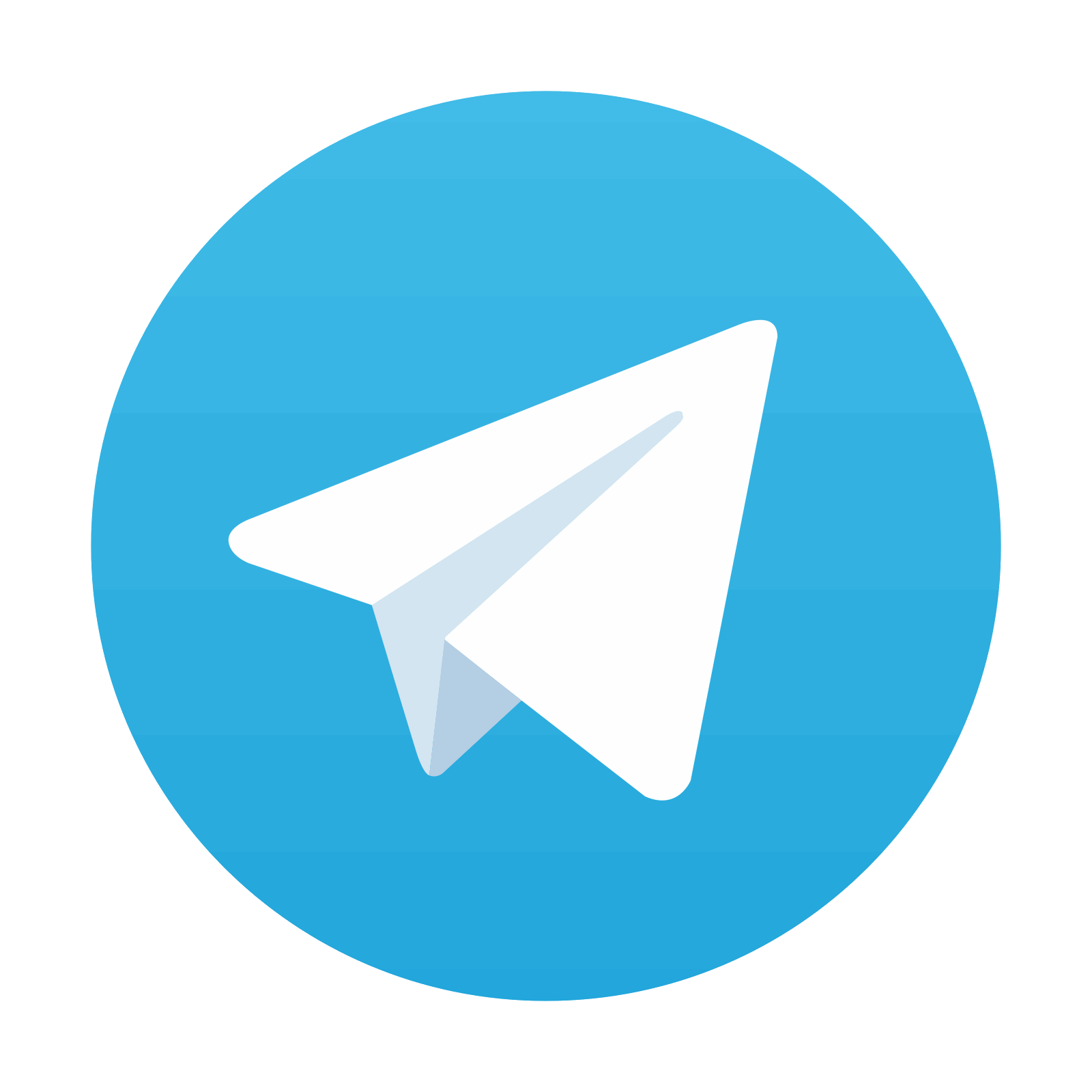
Stay updated, free articles. Join our Telegram channel

Full access? Get Clinical Tree
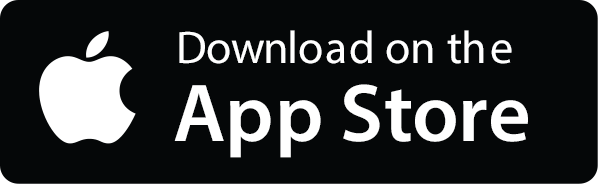
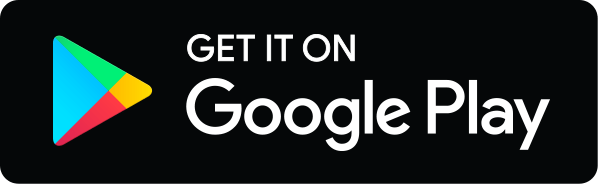