The Hematologic System
The hematologic system includes all the blood cells, the bone marrow in which the cells mature, and the lymphoid tissue where the cells are stored when not in circulation. The hematologic system is designed to:
carry oxygen and nutrients,
transport hormones,
remove waste products,
deliver cells to prevent infection, stop bleeding, and promote healing,
allow the body to feed and heal itself, and
allow the body to communicate between sites.
● Physiologic Concepts
COMPOSITION OF THE BLOOD
The blood is made up of approximately 45% formed elements and 55% plasma. The formed elements are the red blood cells (erythrocytes), the white blood cells (leukocytes), and the platelets (thrombocytes). The red blood cells account for 99% of the formed elements; the white blood cells and platelets constitute the other 1%. The plasma is 90% water, with the other 10% made up of plasma proteins, electrolytes, dissolved gases, various waste products of metabolism, nutrients, vitamins, and cholesterol. Plasma water is responsible for transporting oxygen from the lungs and nutrients from the gastrointestinal tract to the cells of
the body and for delivering waste products to the appropriate excretory organs. The plasma proteins include albumin, the globulins, and fibrinogen. Albumin is the most abundant plasma protein and helps maintain the plasma osmotic pressure and blood volume. The globulins bind insoluble hormones and other plasma constituents to make them soluble. This allows these important substances to be transported in the blood from their site of production to their site of action. Examples of substances that are carried bound to plasma proteins include thyroid hormone, iron, phospholipids, bilirubin, steroid hormones, and cholesterol. Other globulin proteins, the immunoglobulins, are the antibodies that travel in the blood to fight infection. Fibrinogen is a key element in blood clotting.
the body and for delivering waste products to the appropriate excretory organs. The plasma proteins include albumin, the globulins, and fibrinogen. Albumin is the most abundant plasma protein and helps maintain the plasma osmotic pressure and blood volume. The globulins bind insoluble hormones and other plasma constituents to make them soluble. This allows these important substances to be transported in the blood from their site of production to their site of action. Examples of substances that are carried bound to plasma proteins include thyroid hormone, iron, phospholipids, bilirubin, steroid hormones, and cholesterol. Other globulin proteins, the immunoglobulins, are the antibodies that travel in the blood to fight infection. Fibrinogen is a key element in blood clotting.
HEMATOPOIESIS
Red blood cells, white blood cells, and platelets are formed in the liver and spleen in the fetus, and in the bone marrow after birth. The process of blood-cell formation is called hematopoiesis.
Hematopoiesis begins in the bone marrow with pluripotential (meaning “many possible”) stem cells. Stem cells are the source of all blood cells. These cells continually self-renew and differentiate throughout a lifetime; their supply is endless and they are often described as immortal. After several stages of differentiation, a stem cell becomes committed to forming just one type of blood cell. This cell, called a progenitor cell, remains in the marrow and, when instructed by specific growth factors, differentiates into a red blood cell, a white blood cell, or a platelet. The development of the blood cells from original pluripotential stem cells to differentiated cells is shown in Figure 12-1.
Control of Progenitor Cell Differentiation
Progenitor cells are stimulated to proliferate and differentiate by a variety of hormones and locally produced agents that collectively are called hematopoietic growth factors. Each progenitor cell responds only to some of these growth factors, but many growth factors may be acting nonspecifically on
several progenitor cells. Many hematopoietic growth factors are cytokines. Cytokines are released from immune and inflammatory cells and communicate to the progenitor cells the need for more cells to fight infection or help the body heal. Hematopoietic growth factors specific to the line of cells they stimulate are called colony-stimulating factors. For example, granulocyte colony-stimulating factor stimulates the production of white blood cells known as granulocytes (see later), whereas monocyte-macrophage colony-stimulating factor increases the proliferation of monocytes and macrophages. An example of an important colony-stimulating growth factor for red blood cells is the hormone erythropoietin, which is produced by the kidney in response to low oxygen concentration in the blood. Other nonspecific cytokines may act on cells less differentiated than the progenitor cells, stimulating the production of a variety of blood cells.
several progenitor cells. Many hematopoietic growth factors are cytokines. Cytokines are released from immune and inflammatory cells and communicate to the progenitor cells the need for more cells to fight infection or help the body heal. Hematopoietic growth factors specific to the line of cells they stimulate are called colony-stimulating factors. For example, granulocyte colony-stimulating factor stimulates the production of white blood cells known as granulocytes (see later), whereas monocyte-macrophage colony-stimulating factor increases the proliferation of monocytes and macrophages. An example of an important colony-stimulating growth factor for red blood cells is the hormone erythropoietin, which is produced by the kidney in response to low oxygen concentration in the blood. Other nonspecific cytokines may act on cells less differentiated than the progenitor cells, stimulating the production of a variety of blood cells.
THE RED BLOOD CELL
The red blood cell (erythrocyte) contains no nucleus, mitochondria, or ribosomes. It cannot reproduce or undergo oxidative phosphorylation or protein synthesis. The red blood cell contains the protein hemoglobin, which carries oxygen from the lungs to all cells of the body. Hemoglobin takes up most of the red blood cell’s intracellular space. Red blood cells are produced in the bone marrow in response to hemopoietic growth factors, especially erythropoietin, and require iron, folic acid, and vitamin B12 for their synthesis. Once a red blood cell nears maturity, it is released from the bone marrow, completes its maturation in the bloodstream, and lives out its approximately 120-day life span. It then disintegrates and dies. Dying red blood cells are replaced with new ones released from the bone marrow. If red blood cell death is excessive, a larger than normal number of immature red blood cells, called reticulocytes, will be released from the bone marrow; elevated levels of circulating reticulocytes are suggestive of certain types of anemia.
Characteristics of Red Blood Cells
Red blood cells are small, biconcave (two-sided) disks shaped like donuts without holes. Their high surface area allows for rapid diffusion of oxygen and carbon dioxide, while their small size (7 μm in diameter) and relative flexibility allows them to squeeze through even the smallest of capillary beds without damage. In a blood sample, the percentage of the blood that is taken up by red blood cells is called the hematocrit, which usually ranges from approximately 36% to 52% depending on age and sex. The concentration of hemoglobin in a blood sample (grams per 100 mL) usually is approximately one third of the hematocrit. Red blood cells are described clinically by their size and by the amount of hemoglobin present in the cell. The suffix “cytic” refers to size, and the suffix “chromic” refers to the concentration of hemoglobin in the cell. The
mean corpuscular volume (MCV) is a measure in cubic microns of the volume occupied by a single red cell. The MCV is the most commonly used index for identifying whether a cell is of normal, small, or large size and is used clinically to categorize an anemia.
mean corpuscular volume (MCV) is a measure in cubic microns of the volume occupied by a single red cell. The MCV is the most commonly used index for identifying whether a cell is of normal, small, or large size and is used clinically to categorize an anemia.
Normocytic: cells of normal size (MCV 87 to 103 fL/red cell or μm3/red cell)
Microcytic: cells too small in size (MCV < 87 μm3/red cell)
Macrocytic: cells too large in size (MCV > 103 μm3/red cell)
Hypochromic: cells with too little hemoglobin
Normochromic: cells with normal amounts of hemoglobin
Hyperchromic: cells with too dense hemoglobin
Red Blood Cell Antigens
The red blood cell has a variety of specific antigens present on its cell membrane that are not found on other cells. The most important of these antigens are known as A and B and Rh.
ABO Antigens
An individual carries two alleles (genes), each coding for the A antigen, the B antigen, or neither antigen, which is designated O. One allele is received from each parent. The A and B antigens are codominant. Individuals with both the A and B antigen (AB) will have AB blood. Those with two A antigens (AA), or one A and one O (AO), will have A blood. Those with two B antigens (BB), or one B and one O (BO), will have B blood. Individuals with neither antigen (OO) will have O blood. Individuals who have AB blood will accept A, B, or O blood. However, an immune response will develop if an individual without an A or a B antigen is exposed to that antigen in a blood transfusion.
Rh Antigens
Rh antigens are the other main antigen group on the red blood cell and are also passed as genes from each parent. The main Rh antigen is called the Rh factor. An individual who carries the Rh antigen is considered Rh positive (Rh+). An individual who lacks the Rh antigen is considered Rh negative (Rh−). The Rh-positive gene is dominant; therefore, an individual must have two negative Rh factors to be Rh negative. Individuals who are Rh positive will accept Rh-negative blood, but those who do not have the Rh antigen will develop an immune response if exposed to Rh-positive blood.
Universal Blood Recipients and Donors
Universal blood recipients are those with AB-positive blood because their immune system will recognize as self the A or B antigen and the Rh-positive antigen. Therefore, they will accept blood with any ABO or Rh profile. Universal
blood donors are those with O-negative blood. Although their immune systems will attack blood containing the A or B antigen or the Rh factor, their blood can be given for transfusion to any recipient in an emergency (see Table 4-3). Other, weaker antigens on red blood cells exist, however, and may provoke an immune reaction in a recipient.
blood donors are those with O-negative blood. Although their immune systems will attack blood containing the A or B antigen or the Rh factor, their blood can be given for transfusion to any recipient in an emergency (see Table 4-3). Other, weaker antigens on red blood cells exist, however, and may provoke an immune reaction in a recipient.
Hemoglobin
Hemoglobin consists of an iron-containing substance called heme and the protein globulin. There are approximately 300 hemoglobin molecules in each red blood cell. Each hemoglobin molecule contains four binding sites for oxygen. Oxygen bound to hemoglobin is called oxyhemoglobin. Hemoglobin in the red blood cell may be partially or completely bound with oxygen on all four sites. Fully saturated hemoglobin is completely bound with oxygen, while partially saturated or deoxygenated hemoglobin is less than 100% saturated. Systemic arterial blood from the lungs is fully saturated with oxygen. Because hemoglobin releases oxygen to the cells, the hemoglobin saturation in venous blood is approximately 60%. The final job of the hemoglobin is to pick up carbon dioxide and hydrogen ions and carry them to the lungs, where they are exhaled to the air. There are at least 100 types of abnormal hemoglobin molecules that have been recognized in humans, resulting from a variety of different mutations. Most of these mutations cause the hemoglobin molecule to carry oxygen more poorly than normal.
Breakdown of the Red Blood Cell
When the red blood cell begins to disintegrate at the end of its lifetime, hemoglobin is released into the circulation. Hemoglobin is broken down in the liver and spleen. The globulin molecule is converted into amino acids that are used again by the body. The iron is stored in the liver and spleen until it is reused. The rest of the molecule is converted to bilirubin, which is excreted in the stool as bile or in the urine. Normally, the rate of red cell breakdown is equal to the rate of synthesis. In certain conditions, either synthesis or breakdown may outpace the other.
WHITE BLOOD CELLS
White blood cells are formed in the bone marrow from committed progenitor cells. On further differentiation, the progenitor cells become non-granular-appearing T and B lymphocytes, monocytes, and macrophages, or granular-appearing neutrophils, basophils, and eosinophils. The job of the white blood cells is to recognize and fight microorganisms in immune reactions, and to assist in the inflammation and healing processes. The platelets, which are fragments of bone marrow cells, are essential in the control of bleeding. In addition, they often function with the white blood cells in the inflammatory and healing processes.
Types of White Blood Cells
B lymphocytes develop in the bone marrow and then circulate in the blood until they encounter the antigen to which they are programmed to respond. At this point, B lymphocytes mature further, become plasma cells, and begin secreting antibody, as described later.
T lymphocytes leave the bone marrow and develop during migration through the thymus. After leaving the thymus, they circulate in the blood or reside in the lymphatic tissue until they encounter an antigen to which they are programmed to respond. Once stimulated by an antigen, they produce chemicals to destroy the microorganism and alert other white blood cells that an infection is occurring, as described later.
Monocytes are formed in the bone marrow and enter the bloodstream in an immature form. At the site of injury or infection, the monocytes leave the blood and mature into macrophages in the tissues. Macrophages may remain stored in the tissues, or may be used in an inflammatory reaction as soon as they mature.
Neutrophils, basophils, and eosinophils are granular-appearing white blood cells that assist in the inflammatory response. Macrophages, neutrophils, and eosinophils function as phagocytes, cells that destroy and digest microorganisms and accumulated cell debris. Although the exact function of the basophils is unclear, they appear to act like circulating mast cells (Chapter 4) that release vasoactive peptides that stimulate the inflammatory response.
THE SPLEEN
The spleen is a small organ located in the upper left abdominal cavity. It is considered a secondary lymphoid organ, as opposed to the bone marrow and thymus, which are primary lymphoid organs. Like all lymphoid organs, the spleen is involved in the formation and storage of the blood.
The spleen is the site of hematopoiesis in the fetus. After birth, the spleen contains tissue macrophages and aggregates of lymphocytes. The spleen is well supplied with blood vessels that branch off from the splenic artery, which is a branch of the abdominal aorta. The intricate vasculature of the spleen circulates blood containing microorganisms, dead cells, and other debris past the macrophages and lymphocytes, where they can be acted on or destroyed. After flowing through the splenic capillary networks, the blood vessels rejoin into venules, and blood is delivered to the liver through the hepatic portal blood flow system.
As blood passes through the spleen, residing macrophages act as phagocytes to clear the blood of cell debris (including lysed red blood cells) and digest microorganisms. The macrophages present pieces of digested microorganisms to nearby B and T cells, initiating an immune response. Individuals who have lost their spleen (usually after trauma, although some individuals may have
their spleen removed surgically when platelet count is low and cannot be corrected) are at a disadvantage in fighting certain infections compared to those with a functioning spleen.
their spleen removed surgically when platelet count is low and cannot be corrected) are at a disadvantage in fighting certain infections compared to those with a functioning spleen.
The spleen also serves as a reservoir for blood, capable of holding a few hundred milliliters in the adult. With a decrease in blood pressure, the spleen can expel this blood into the venous circulation to help return pressure. It also serves as a storage site for iron released during the catabolism of hemoglobin. Iron is stored in splenic macrophages until required for production of new red blood cells. Iron deficiency may occur without the spleen. The spleen also stores senescent (aged) red blood cells.
LYMPH NODES
Lymph nodes are small capsules of lymphoid tissue interspersed throughout the lymphatic system, near the lymphatic veins. Lymph flowing in the lymphatic vessels is filtered through many nodes.
Lymph nodes contain many lymphocytes, monocytes, and macrophages. These cells proliferate in the nodes and some are released into the circulation during infection or inflammation. Residing white blood cells filter, detect, entrap, and phagocytize microorganisms that are delivered by the lymph flow, cleansing the lymph before it is returned to the general circulation. The lymph node closest to an infection is exposed to the highest number of microorganisms, which causes the macrophages and lymphocytes to proliferate and the node to enlarge. An active node may become tender and palpable as it fights to contain infection.
HEMOSTASIS
The human body experiences frequent small capillary tears and occasional large blood vessel cuts. While unable to control large vessel bleeding without external support, the body is able to stop small vessel bleeding. Control of bleeding occurs in two steps—the formation of a platelet plug followed by the formation of a blood clot. These processes are interdependent and occur one after the other in rapid succession. The control of bleeding is called hemostasis.
Role of the Platelets in Hemostasis
Platelets play an important role in both steps of hemostasis. Platelets normally circulate throughout the bloodstream without sticking to vascular endothelial cells. However, within seconds after damage to a blood vessel, platelets are drawn to the area in response to exposed collagen on the subendothelial layers of the damaged blood vessel. Platelets attach to proteins (called von Willebrand factors [vWF]) expressed on the damaged surface of a blood vessel, and release
several vasoactive chemicals, including serotonin and adenosine diphosphate (ADP). Serotonin causes vasoconstriction, helping to reduce blood flow to the area and limit bleeding. Serotonin and other chemicals, including ADP, also cause the platelets to change shape and become sticky, beginning the formation of what is called a platelet plug inside the damaged blood vessel. Other platelets are drawn to the area and further build up the plug. Thromboxane A2 is produced by the platelets and contributes to the attraction of more platelets to the area. Fibrinogen, a circulating plasma protein, connects between exposed sites on the platelets, serving like a bridge to add stability to the plug. The platelet plug effectively seals the damaged area. Deficiencies in any of the involved factors will result in excessive bleeding of even small capillary tears.
several vasoactive chemicals, including serotonin and adenosine diphosphate (ADP). Serotonin causes vasoconstriction, helping to reduce blood flow to the area and limit bleeding. Serotonin and other chemicals, including ADP, also cause the platelets to change shape and become sticky, beginning the formation of what is called a platelet plug inside the damaged blood vessel. Other platelets are drawn to the area and further build up the plug. Thromboxane A2 is produced by the platelets and contributes to the attraction of more platelets to the area. Fibrinogen, a circulating plasma protein, connects between exposed sites on the platelets, serving like a bridge to add stability to the plug. The platelet plug effectively seals the damaged area. Deficiencies in any of the involved factors will result in excessive bleeding of even small capillary tears.
Limits on Platelet Function
Unimpeded platelet aggregation could cause a prolonged decrease in blood flow to the tissue or result in a plug becoming so enlarged that it may break off from the original site and travel downstream as an embolus, blocking downstream flow. To prevent either of these occurrences from happening, neighboring undamaged endothelial cells release other substances that limit the extent of platelet aggregation. The main substances released by neighboring endothelial cells to limit platelet aggregation are prostaglandin I2, also called prostacyclin, and nitric oxide, an important vasodilator.
Ultimately, the balance between proclotting and anticlotting factors serves to keep the platelets active at the site of injury while preventing excessive platelet aggregation and spread of the platelet plug to uninjured vascular tissue. Platelet aggregation and the production of the platelet plug are shown in Figure 12-2.
Blood Clot
The platelet plug becomes a true clot as it enlarges and traps circulating red cells and macrophages. The entire clot is stabilized and strengthened by a network of fibrin strands, produced from the fibrinogen bridges mentioned earlier. The production of stabilized fibrin is the final step in the other essential component of hemostasis, the coagulation cascade.
Coagulation Reactions
Coagulation reactions involve a series of coagulation factors or proteins activated in domino fashion, leading to the coagulation (clotting) of the blood. There are a total of 13 proteins involved in the coagulation pathways; some of these are activated in what is called the intrinsic pathway and some are activated in the extrinsic pathway. Under most physiologic conditions, coagulation occurs first through the extrinsic pathway; activation of the extrinsic pathway then turns on the more powerful intrinsic pathway. Both pathways ultimately
merge and function by activating one protein, factor X; the merging of the intrinsic and extrinsic pathways at factor X is called the final common pathway. Factor X is responsible for converting the plasma protein prothrombin to thrombin. Thrombin is the key catalyst that drives the conversion of fibrinogen to fibrin and causes coagulation. Thrombin also acts in a positive feedback manner to stimulate the proteins involved in its own production, furthering the coagulation cascade. Both pathways are shown in Figure 12-3.
merge and function by activating one protein, factor X; the merging of the intrinsic and extrinsic pathways at factor X is called the final common pathway. Factor X is responsible for converting the plasma protein prothrombin to thrombin. Thrombin is the key catalyst that drives the conversion of fibrinogen to fibrin and causes coagulation. Thrombin also acts in a positive feedback manner to stimulate the proteins involved in its own production, furthering the coagulation cascade. Both pathways are shown in Figure 12-3.
The intrinsic pathway begins with the activation of the circulating coagulation factor, factor XII, also called the Hageman factor. Factor XII is activated when it comes into contact with damaged vascular tissue. Ultimately, activation of factor XII leads to the conversion of prothrombin to thrombin. Factors XI and IX are important intermediate steps in the cascade, and factors V and VIII are important cofactors. Lack of any of these factors could interfere with coagulation.
The extrinsic pathway, the usual way of stimulating coagulation, begins when damaged vascular endothelial cells release factor III, also called tissue factor or thromboplastin, into the circulation. When tissue factor encounters another coagulation factor circulating in the plasma, factor VII (also called serum prothrombin conversion factor), the extrinsic cascade is stimulated, again resulting in the production of factor X. The extrinsic pathway also can turn on the intrinsic pathway through the activation of factor IX.
The blood does not continually and excessively clot even though factors XII and VII are always present in the circulation because healthy endothelial cells are smooth and intact. Therefore, they do not directly activate factor XII or produce tissue factor and activate factor VII. Healthy vascular endothelium repels coagulation factors and platelets. It is only when the endothelium is damaged by trauma, infection, forces of chronic hypertension, or accumulation of fat and cholesterol (Chapter 13) that a clot begins to develop.
Because several coagulation factors are produced in the liver in reactions involving vitamin K, liver disease or vitamin K deficiency can impair the production of coagulation factors and cause bleeding.
Anticoagulants
Anticoagulants are present in the blood to prevent clots from developing. For example, antithrombin proteins are released by undamaged endothelial cells and function to inactivate thrombin. The most important of these, antithrombin III, is itself activated by heparin, an anticoagulant produced by mast cells and basophils in response to tissue injury and inflammation. Other substances, called
tissue factor inhibitors, circulate in the plasma and bind to tissue factor (factor III), directly blocking its activation and interfering with the extrinsic pathway. Finally, as mentioned earlier, nondamaged endothelial cells secrete prostacyclin and nitric oxide, which limit platelet aggregation and thus reduce coagulation.
tissue factor inhibitors, circulate in the plasma and bind to tissue factor (factor III), directly blocking its activation and interfering with the extrinsic pathway. Finally, as mentioned earlier, nondamaged endothelial cells secrete prostacyclin and nitric oxide, which limit platelet aggregation and thus reduce coagulation.
Anticoagulation drugs are available and include the prostaglandin inhibitor aspirin, which in low doses inhibits the production of thromboxane A2 but not prostaglandin I2, and oral anticoagulants such as warfarin. Other drugs are available that do not prevent clotting, but serve to break down previously formed clots. Examples of these drugs, called thrombolytic agents, include streptokinase and t-PA; thrombolytic agents play an important role in the early treatment of myocardial infarct and thrombotic stroke.
LABORATORY TESTS OF THE BLOOD
The Complete Blood Count with Differential and Platelet Count
The blood is frequently tested for adequacy of cell number and function. The most common test is the complete blood count (CBC), which provides information on the number, concentration, and physical characteristics of red blood cells, white blood cells, and platelets present in a venous blood sample. The CBC with differential is age dependent and, to a lesser extent, sex dependent. Exercise, reproductive status, and many drugs may cause test deviations. The CBC with differential is used as part of well physical examinations, to screen for specific conditions, and to determine preoperative health. The CBC is also used to evaluate treatment success.
A description of red cell size as indicated by the mean corpuscular volume (MCV) and mean corpuscular hemoglobin concentration (MCHC) provides additional information when evaluating patients with anemia. Red cells also are described by the red cell size distribution width (RDW) in a blood sample. If the RDW is high, it means there is a wide range of RBC sizes in the sample. The RDW is useful in distinguishing between similar types of anemia. For example, a patient with microcytic (small) red cells who has a normal RDW may have a hemoglobin abnormality such as thalassemia, while a patient with similarly microcytic cells but a high RDW is more likely experiencing iron deficiency. Other combinations of red cell values provide different clues to the etiology of blood disorders.
Other common blood tests include blood typing of ABO and Rh antigens and tests to identify the presence of microorganisms and antibody titers. The erythrocyte sedimentation rate (SED rate) is a test that evaluates the tendency of red blood cells to settle out of unclotted blood in 1 hour. This test is based on the fact that inflammation and similar processes stimulate the liver to release an increased number of proteins into the blood, which cause red cells to aggregate together, becoming heavier and thus settling to the bottom of a container. Because of this, the SED rate is often increased nonspecifically with inflammatory disease.
The Normal CBC with Differential and Platelet Count (Adult)
Red blood cell count: 4.0 to 5.5 million/mL of blood
White blood cell count: 5000 to 10,000/mL of blood
Platelet count: 140,000 to 400,000/mL of blood
Hematocrit (% of red blood cells): 42% to 52% for males; 36% to 48% for females
Hemoglobin: 14.0 to 17.5 g/100 mL for males; 12.0 to 16.0 g/100 mL for females
Neutrophils: 50% to 62%
Eosinophils: 0% to 3%
Basophils: 0% to 1%
Lymphocytes: 25% to 40%
Monocytes: 3% to 7%
Tests of Red Blood Cell Size and Hemoglobin (Adult)
MCV: 82 to 98 fL/red cells
MCHC: 32 to 36 g/dL
RDW: 11.5 to 14.5 coefficient of variation of red cell size
Sedimentation Rate
SED rate: 0 to 20 mm/hour
Bleeding Time
Bleeding time refers to the length of time bleeding occurs after a standardized puncture wound to the skin. Bleeding time is measured in minutes and indicates the functioning status of the platelets, specifically the effectiveness of the platelet plug. Bleeding time should not exceed 15 minutes (normal: 3.0 to 9.5 minutes) for a forearm stick.
Partial Thromboplastin Time/Prothrombin Time
Partial thromboplastin time (PTT) and prothrombin time (PT) detect deficiencies in the activity of various clotting factors. Both tests evaluate clotting in a venous blood sample.
PTT especially demonstrates the effectiveness of the intrinsic pathway of coagulation and should not exceed 90 seconds (normal: 30 to 45 seconds). This test is important in determining the effectiveness and safety of heparin therapy.
PT demonstrates the effectiveness of the vitamin K-dependent coagulation factors, especially the extrinsic and common pathways of coagulation.
PT should not exceed 40 seconds, or 2 to 2.5 times a control level (normal: 11 to 13 seconds). PT is used to determine the effectiveness of warfarin (Coumadin) therapy.
PT should not exceed 40 seconds, or 2 to 2.5 times a control level (normal: 11 to 13 seconds). PT is used to determine the effectiveness of warfarin (Coumadin) therapy.
The international normalized ratio (INR) is a worldwide standard for evaluating the extrinsic pathway of coagulation. This test allows for standardization regardless of the reagents, unlike the PT, which may vary depending on the laboratory reagent. The INR should be less than 2.0 for anyone not taking anticoagulants. For patients receiving anticoagulant therapy, values up to 3.5 are considered in the normal range depending on the reason for therapy (venous thrombosis, mechanical heart valve, recurrent systemic embolus).
● Pathophysiologic Concepts
ANEMIA
Anemia is a decrease in the quantity of circulating red blood cells, an abnormality in the hemoglobin content of red blood cells, or both. Anemia can be caused by a disorder in red blood cell production or an elevated loss of red blood cells through chronic bleeding, sudden hemorrhage, or excessive lysis (destruction). All anemias result in decreased values for hematocrit and hemoglobin but may vary in values of MCV, MCHC, and RDW. For example, using the MCV as an index, a microcytic anemia has a MCV < 82 fL/red cell; a normocytic anemia has a MCV between 82 and 98 fL/red cell; and a macrocytic anemia has a MCV > 98 fL/red cell. The symptoms associated with anemia depend on its duration, its severity, and the host’s age and prior health status. All symptoms ultimately relate to a reduction in the delivery of oxygen to host cells and organs, thereby interfering with function and compromising health. Clinical manifestations may include tachycardia, orthostatic hypotension, murmurs, dyspnea, tachypnea, headache, lightheadedness, fatigue, pallor, and intermittent claudication.
Anemia Caused by a Disorder in Red Cell Production
Anemias that result from a disorder in red cell production occur if there is inadequate or inaccessible iron, or a lack of folic acid, vitamin B12, or globulin. Red blood cell production may also be insufficient if there is bone marrow disease, as would occur in leukemia, after radiation exposure, or with other diseases of the marrow. A deficiency in erythropoietin, as would occur in renal failure, would also lead to a decrease in red cell production. Anemias due to disorders in RBC production may result in a red cell that is too small (microcytic) or too large (macrocytic), and hemoglobin content that is abnormally low (hypochromic).
TABLE 12-1 Common Anemias | ||||||||||||||||||||||||||||||||||||||
---|---|---|---|---|---|---|---|---|---|---|---|---|---|---|---|---|---|---|---|---|---|---|---|---|---|---|---|---|---|---|---|---|---|---|---|---|---|---|
|
Anemia Caused by Sudden or Chronic Hemorrhage or Lysis
Anemias caused by sudden hemorrhage, a slow chronic hemorrhage, or lysis result in a decrease in the total number of circulating red cells. This type of anemia may be associated with an increased percentage of circulating immature red cells (reticulocytes). Normal red blood cells live approximately 120 days. Red cell destruction or loss occurring before 100 days is abnormal. Common anemias, their causes, and laboratory profiles are shown in Table 12-1, and some are discussed more fully in the final section of this chapter.
POLYCYTHEMIA
Polycythemia is an increase in the number of red blood cells. Primary polycythemia (polycythemia vera) is characterized by an increase in platelets and granulocytes as well as red blood cells, and is believed to be the result of a precursor cell abnormality.
Polycythemia may occur secondarily to chronic hypoxia. Chronic hypoxia causes increased release of the renal hormone erythropoietin, which stimulates the production of red blood cells. Individuals who live at high altitude or suffer from chronic lung disease frequently experience secondary polycythemia.
Athletes who blood dope, meaning they accept self-transfusions of previously collected packed red cells, demonstrate polycythemia. And finally, polycythemia may be relative, rather than absolute. During dehydration, for instance, a decrease in plasma volume is reflected as an increase in the concentration of red cells. Polycythemia from any cause is associated with an increased risk of thrombus formation and an increase in the workload of the heart. Clinical manifestations are the result of increased blood viscosity and may include hypertension, headache, inability to concentrate, and impaired hearing and vision secondary to decreased cerebral blood flow.
Athletes who blood dope, meaning they accept self-transfusions of previously collected packed red cells, demonstrate polycythemia. And finally, polycythemia may be relative, rather than absolute. During dehydration, for instance, a decrease in plasma volume is reflected as an increase in the concentration of red cells. Polycythemia from any cause is associated with an increased risk of thrombus formation and an increase in the workload of the heart. Clinical manifestations are the result of increased blood viscosity and may include hypertension, headache, inability to concentrate, and impaired hearing and vision secondary to decreased cerebral blood flow.
LEUKOPENIA
Leukopenia is an absolute decrease in the number of white blood cells. Leukopenia may be caused by a variety of conditions, including prolonged stress, viral infection, bone marrow disease or destruction, radiation, or chemotherapy. Severe systemic diseases such as lupus erythematosus, thyroid disease, and Cushing syndrome may cause a decrease in white blood cells. All or one type of white cells may be affected although neutrophils, as the most prominent type of granulocytes, are most often affected. Leukopenia may predispose the individual to infection.
LEUKOCYTOSIS
Leukocytosis is an increase in the number of circulating white blood cells. Leukocytosis is a normal response to infection or inflammation. It can be seen after emotional disturbance, after anesthesia or exercise, and during pregnancy. Abnormal leukocytosis is observed in certain malignancies and bone marrow disorders. Usually, only one type of white blood cell is affected. For example, allergic responses and asthma are specifically associated with increased numbers of eosinophils. Leukemia is characterized by an abnormally high level of one type of white cell and deficiencies in the others.
SHIFT TO THE LEFT
Shift to the left is a term used to describe an increased proportion of immature leukocytes (usually neutrophils) seen in the blood of an individual fighting an infection. In a shift to the left, neutrophils will be released from the bone marrow before their final maturation, when the demand for white blood cells is excessive. The immature neutrophils are frequently referred to as bands or stabs. As the infection or inflammation begins to recede, the release of immature neutrophils stops and the blood is said to show a return shift to the right, as mature neutrophils again dominate a blood smear. The returning mature neutrophils are frequently referred to as segmented neutrophils.
THROMBOCYTOPENIA
Thrombocytopenia is a decrease in the number of circulating platelets. It is associated with increased risk of severe bleeding, even with small injuries or small spontaneous bleeds. Thrombocytopenia is characterized by small spots of subcutaneous bleeding, called petechiae, or larger areas of subcutaneous bleeding, called purpura.
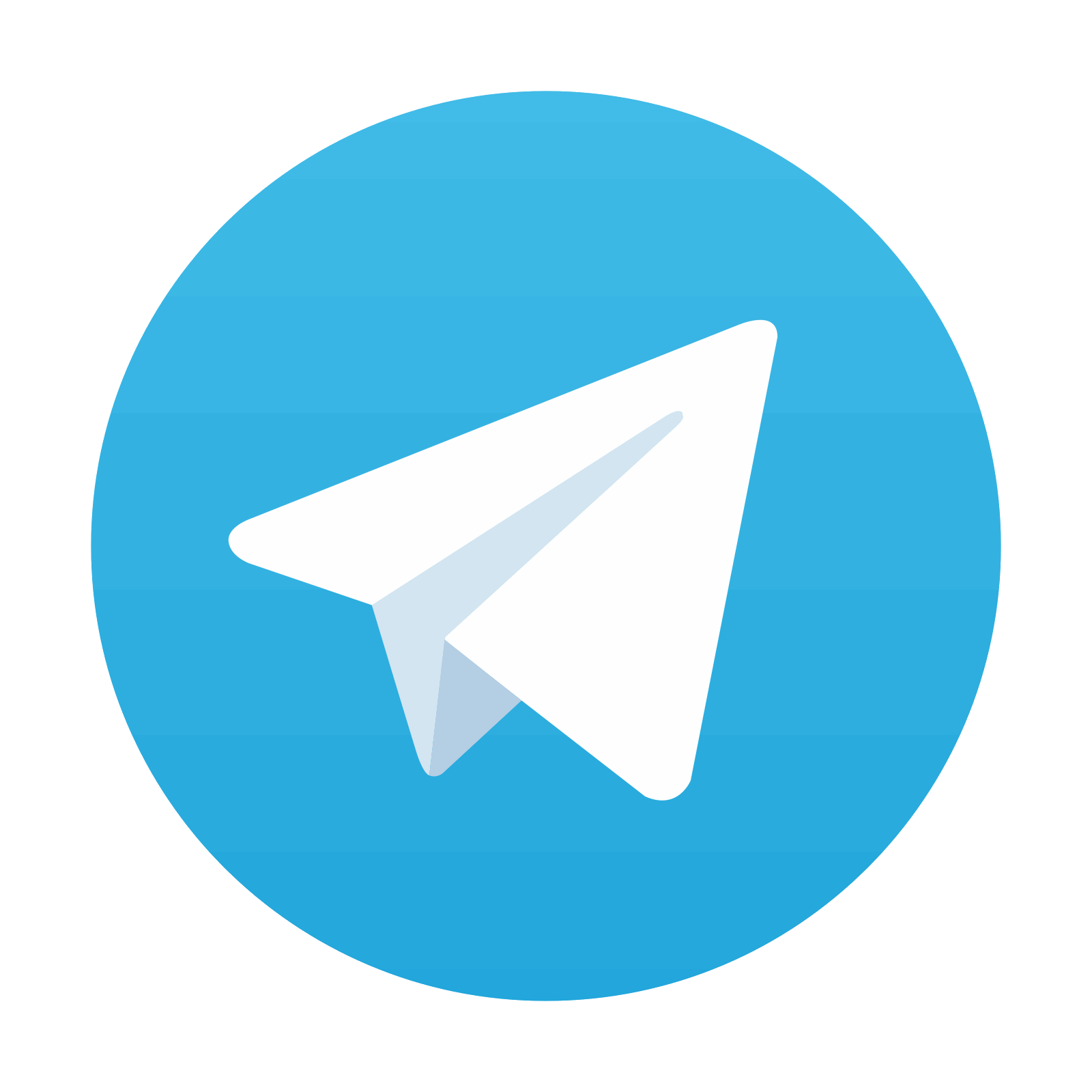
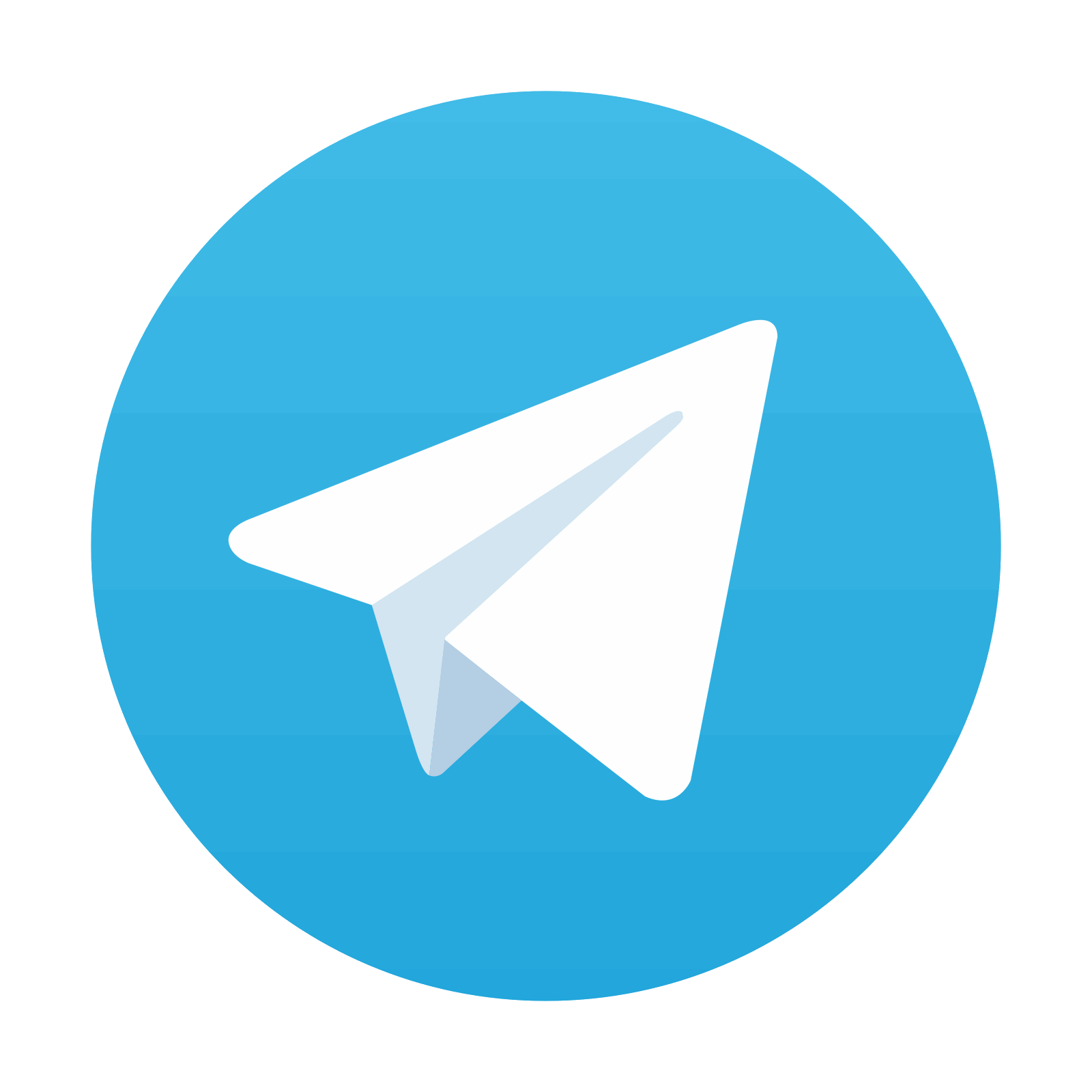
Stay updated, free articles. Join our Telegram channel

Full access? Get Clinical Tree
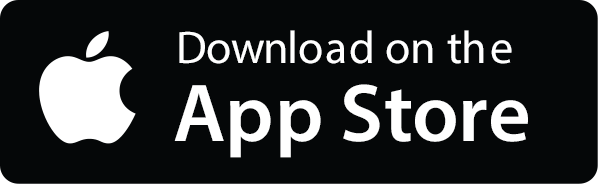
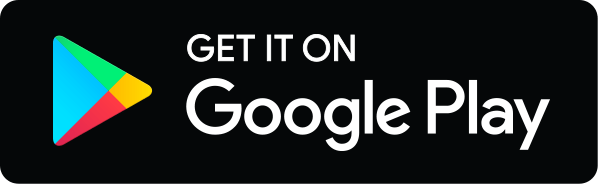
