Chapter Seventeen. The cardiovascular system
Introduction
There are three main roles for the cardiovascular system:
1. Delivery of nutrients and oxygen.
2. Removal of metabolic waste and carbon dioxide.
3. Dissipation of heat from active tissues and redistribution of heat around the body.
Circulatory pathways
Blood flows through a network of blood vessels that extend between the heart and peripheral tissues. The circulation of blood can be subdivided into two distinct circuits, which both begin and end in the heart. The pulmonary circulation takes deoxygenated blood from the right side of the heart to the lungs and returns oxygenated blood from the lungs to the left side of the heart. The systemic circulation takes oxygenated blood from the left side of the heart to all the tissues and returns deoxygenated blood to the right side of the heart. Exchange of nutrients and metabolic waste products takes place in the systemic circulation. In a normal adult at rest the amount of blood circulated through the heart is 5 litres per minute (L/min), which is the same as the amount of blood in the circulation.
The force required to move blood around the body comes from the heart, which is essentially two separate pumps: the left side supplies the systemic circulation and the right side supplies the pulmonary circulation. As a general principle, veins carry blood to the heart: oxygenated in the pulmonary circulatory system and deoxygenated in the systemic veins (Fig. 17.1). Arteries carry blood away from the heart: deoxygenated in the pulmonary circulatory system and oxygenated in the systemic circulation.
![]() |
Figure 17.1 A general plan of the circulatory system. (From Montague S E, Watson R, Herbert R A 2005, with kind permission of Elsevier.) |
Anatomy of the heart
Description
The heart lies in the mediastinum of the thoracic cavity between the two lungs enclosed in their pleural sacs. It is positioned with two-thirds of its mass to the left of the body’s midline. It is shaped like a blunt cone with its apex pointing downwards and to the left. The heart covers about 12–14 cm from the second to the fifth intercostal space; its base, which points upwards towards the right shoulder, is about 9 cm wide. The heart of an adult normally weighs about 300 g.
Layers
The myocardium, endocardium and pericardium make up the three layers of the heart.
The myocardium or contractile wall of the heart consists mainly of cardiac muscle. Connective tissue forms a dense fibrous network which reinforces the myocardium and anchors the muscle fibres. This fibrous network limits the spread of electrical action potentials to specific pathways.
The inner lining or endocardium consists of squamous epithelium resting on connective tissue. This also covers the valves and the tendons that hold them in place. It is continuous with the endothelial lining of the blood vessels entering the heart.
The heart is enclosed in a fibroserous sac called the pericardium, which protects it and anchors it to the large blood vessels, diaphragm and sternal wall. It has two layers: an outer fibrous layer and an inner serous pericardium. The serous pericardium is also composed of two layers: the outer parietal layer and the inner visceral layer next to the myocardium called the epicardium. Between the visceral and parietal layers of the serous pericardium is the pericardial cavity, which is filled with pericardial fluid. This provides a friction-free area within which the heart can pump.
Chambers and valves
There are four chambers in the heart: two superior atria and two inferior ventricles. The right ventricle forms most of the anterior surface of the heart while the left and largest ventricle forms the apex and the inferior posterior aspect of the heart. These chambers are separated by valves and septa: the interatrial septum and the interventricular septum. The valves are attached to papillary muscles by the chordae tendinae, which anchor them in the closed position. The valves direct and control the flow of blood through the heart by opening as the associated chamber contracts and closing as the chamber relaxes. The valves ensure a one-way flow of blood through the heart (Fig. 17.2).
![]() |
Figure 17.2 The direction of blood flow within the heart. (From Montague S E, Watson R, Herbert R A 2005, with kind permission of Elsevier.) |
The atrioventricular valves
The tricuspid valve separates the right atrium from the right ventricle. The mitral or bicuspid valve separates the left atrium from the left ventricle.
The semilunar valves
The pulmonary valve separates the right ventricle from the pulmonary artery. The aortic valve separates the left ventricle and the aorta.
The coronary circulation
Oxygen is carried to the cardiac muscle by the right and left coronary arteries, which originate from the aorta just beyond the aortic valve. The right coronary artery supplies the right atrium, right ventricle and portions of the left ventricle. The left coronary artery divides near its origin into:
• The left anterior descending branch, supplying the anterior part of the left ventricle and a small part of the right ventricle.
• The circumflex branch, which supplies blood to the left atrium and upper left ventricle.
Blood returns from the left side of the heart to the right atrium via the coronary sinus and blood returns from the right side of the heart via small anterior cardiac veins.
Pulmonary and systemic circulations
The pulmonary circulation takes deoxygenated blood from the right atrium to the lungs via the pulmonary trunk, which divides into two pulmonary arteries, one directed to each lung. The arteries further subdivide until the capillary level where they unite into venules and then veins. Oxygenated blood is then returned to the left atrium from the lungs via four pulmonary veins.
In the systemic circulation, oxygenated blood leaves the left ventricle via the aorta and is diverted to all the tissues and cells around the body through smaller arteries and arterioles. At tissue level the blood reaches capillaries, merging with venules to form veins. These veins unite to return deoxygenated blood to the right atrium through two large veins called the venae cavae: the inferior vena cava collects blood from the lower body and the superior vena cava collects blood from the upper body.
Physiology of the heart
Cardiac muscle combines properties of both skeletal and smooth muscle (see Ch. 25). It is striated like skeletal muscle but individual muscle cell membranes have very low electrical resistance. Structures called intercalated discs (Fig. 17.3) allow action potentials to pass easily from one cardiac muscle cell to another so that the muscle mass can function as a whole. Intercalated discs contain anchoring units called desmosomes to hold the fibres together. Gap junctions between the muscle cells allow easy movement of ions to facilitate the spread of action potentials. The action potential is prolonged, allowing the electrical impulse to travel over the whole atrial and ventricular mass so that the cardiac muscle contracts as a unit. There is then a prolonged refractory period where relaxation phase occurs and no further contraction can begin. This is when the heart chambers refill with blood.
![]() |
Figure 17.3 The structure of cardiac muscle showing the intercalated discs. (From Montague S E, Watson R, Herbert R A 2005, with kind permission of Elsevier.) |
Both atria contract together, propelling blood into each ventricle. Both ventricles then contract together, propelling blood into the pulmonary and systemic circulations. As the atria contract, the ventricles are relaxed so they can fill up with blood. As the ventricles contract, the atria are relaxed and fill up with blood ready for the next cycle.
The electrical conducting system (nodal system)
The electrical conducting system of the heart has the following components:
• Sinoatrial (SA) node.
• Atrioventricular (AV) node.
• Atrioventricular bundle of His.
• Left and right branch bundles.
• Purkinje fibres.
The SA node, located in the right atrium, initiates the action potential that causes contraction. It then spreads through both atria and enters the AV node at the base of the right atrium. This, plus the bundle of His, provide the only conduction link to the ventricles. There is a 0.1 s delay in conduction, allowing the atria to complete contracting and emptying their blood into the ventricles. The wave now spreads via the left and right branch bundles, which lie on either side of the interventricular septum, to the Purkinje fibres and the ventricular muscle. The spread is simultaneous and there is coordinated contraction.
The cardiac cycle
The cardiac cycle is taken from the end of one contraction to the end of the next (Fig. 17.4). It produces two distinct sounds in a single beat, ‘lub-dup’. The first heart sound is produced by the closure of the atrioventricular valves at the beginning of ventricular contraction or systole. The second sound is produced by the closure of the semilunar valves at the beginning of ventricular relaxation or diastole. The heart rate is the number of cycles or beats per minute (bpm). At an average heart rate of 72 bpm, each cardiac cycle lasts approximately 0.8 s with approximately 0.4 s being in systole and 0.4 s in diastole.
![]() |
Figure 17.4 The cardiac cycle. |
Control of the heart rate
Intrinsic control
The intrinsic conduction system of the heart allows the heart muscle to beat on its own with no external control. The heart’s electrical conduction system has autorhythmicity. The SA node acts as a pacemaker and in the absence of any nervous or hormonal influences initiates a rate of 100 bpm. Other parts of the conducting system also have autorhythmicity. The unopposed AV node can initiate a rate of 40–60 bpm and the rest of the system will initiate a rate of 15–40 bpm.
Extrinsic control
The heart rate can also be externally influenced by the autonomic nervous system, hormones such as adrenaline (epinephrine), stretching the atria, temperature and drugs.
Nervous control
In the medulla oblongata the cardiovascular centre (CVC) receives input from baroreceptors, chemoreceptors and higher centres in the brain such as the cortex and hypothalamus (Fig. 17.5). The CVC can be subdivided into the cardiac centre, affecting heart function, and the vasomotor centre, affecting blood vessels, but these probably function interactively. Both sympathetic fibres (from the CVC) and parasympathetic fibres (from the vagus nerve) innervate the SA node. Sympathetic activity causes the heart rate to increase and parasympathetic activity causes the heart rate to decrease (Fig. 17.6). This parasympathetic influence, dominant at rest, is sometimes known as the vagal brake. This explains why a normal resting heart rate averages 70 bpm compared with the unopposed SA node rate of 100 bpm.
![]() |
Figure 17.5 Diagrammatic representation of afferent and efferent pathways associated with the cardiovascular control centre. (From Hinchliff S M, Montague S E 1990, with permission.) |
![]() |
Figure 17.6 Sympathetic and parasympathetic innervation of the heart. (based on Tortora & Anagnostakos 1981) |
Hormonal control
Adrenaline (epinephrine) stimulates β 1 receptors in cardiac muscle and causes the heart rate to increase in response to stress. The hormones noradrenaline (norepinephrine) and thyroid hormone also enhance the effect of the sympathetic nervous system to increase heart rate.
Stretch
Stretching of the atrial walls can be caused by increased venous return or increased blood volume. Atrial stretching can increase the heart rate by 10–15%. This is the Bainbridge reflex and occurs because the stretch receptors in the atrial walls send impulses to stimulate sympathetic output.
Stroke volume
Excess blood also stretches the ventricles ( ventricular end-diastolic volume (VEDV)). The more the ventricles are stretched before contraction, the greater the force of contraction and the greater the amount of blood leaving the heart. This is Starling’s law of the heart. The amount of blood leaving each ventricle during one contraction is called the stroke volume (SV) and is normally 70 ml. A ventricle does not empty completely when it contracts. The blood left in the ventricle at the end of systole is the ventricular end-systolic volume (VESV). Typical values for an adult at rest are SV = 70 ml, VEDV = 135 ml and VESV = 65 ml and can be represented by the following equation:

(17.1)

In health, adding the atrial contents to the remaining blood in the ventricle brings about the extra VEDV of the next cycle. This increases the contraction force, causing the ventricle to empty more completely and thus maintaining SV at a constant level.
Cardiac output
The volume of blood pumped by each ventricle per minute is called cardiac output (CO), usually expressed in L/min. It is also the volume of blood flowing through either the systemic or pulmonary circuit per minute. The CO is determined by multiplying the heart rate, the number of beats per minute (bpm), by the stroke volume, the blood ejected by each ventricle with each beat:

(17.2)

Thus if each ventricle has a rate of 72 bpm and ejects 70 ml of blood with each beat, from Eqn (17.2) the cardiac output is:


These are approximate values for a healthy adult at rest. Since the total blood volume is also approximately 5 litres, this means that essentially all the blood is pumped around the circuit once each minute. Cardiac output may reach 35 L/min in well-trained athletes during periods of strenuous exercise (i.e. total blood volume pumped around the circuit seven times a minute). Sedentary individuals can reach cardiac outputs of 20–25 L/min. The difference between the cardiac output at rest and the potential cardiac output is called the cardiac reserve.
In response to being stretched the atria secrete a hormone called atrial natriuretic factor (ANF) or atrial natriuretic peptide (ANP). This is a potent diuretic that causes the kidney to excrete excess sodium and water, resulting in a decrease of blood volume and blood pressure.
Other influences (Fig. 17.7)
• Alterations in core body temperature influence heart rate: there will be an increase in heart rate with an increase in temperature, whereas lowering of core body temperature will decrease the heart rate. This latter is seen in people with hypothermia. The changes in body temperature alter the rate of electrical discharge (Fig. 17.7).
• A raised arterial blood pressure may decrease SV because the ventricles must exert force against a greater load. A normal heart will self-adjust to counter this by increasing the force of ventricular contraction. If the blood pressure is chronically raised, the left ventricle will hypertrophy and fail.
![]() |
Figure 17.7 Main factors that can alter cardiac output. An increased arterial blood pressure (i.e. an increased afterload) causes a decrease in stroke volume and consequently a decrease in cardiac output. CO, cardiac output; SV, stroke volume; HR, heart rate; VEDV, ventricular end-diastolic volume; RAP, right atrial pressure; VR, venous return; Ad, adrenaline; NAd, noradrenaline. (From Hinchliff S M, Montague S E 1990, with permission.) |
The vascular system
The vascular system delivers blood to all tissues as needed and returns blood to the heart (Figure 17.8 and Figure 17.9). To achieve this the system must be able to adapt to local needs. A change from pulsatile arterial blood flow to a steady capillary flow is necessary to allow the effective exchange of nutrients and waste to occur at the capillary beds.
![]() |
Figure 17.8 Major arteries of the human body (anterior view). (From Hinchliff S M, Montague S E 1990, with permission.) |
![]() |
Figure 17.9 Major veins of the human body (anterior view). (From Hinchliff S M, Montague S E 1990, with permission.) |
In the systemic circulation, blood leaves the left side of the heart via the aorta, which subdivides into smaller arteries. The smallest are arterioles, branching into capillaries where the exchange of gases, nutrients and metabolic wastes occurs. Capillaries unite to form venules and these unite to form larger veins. Finally, the two largest veins, the inferior vena cava returning blood from the lower part of the body and the superior vena cava returning blood from the upper part of the body, enter the right atrium of the heart.
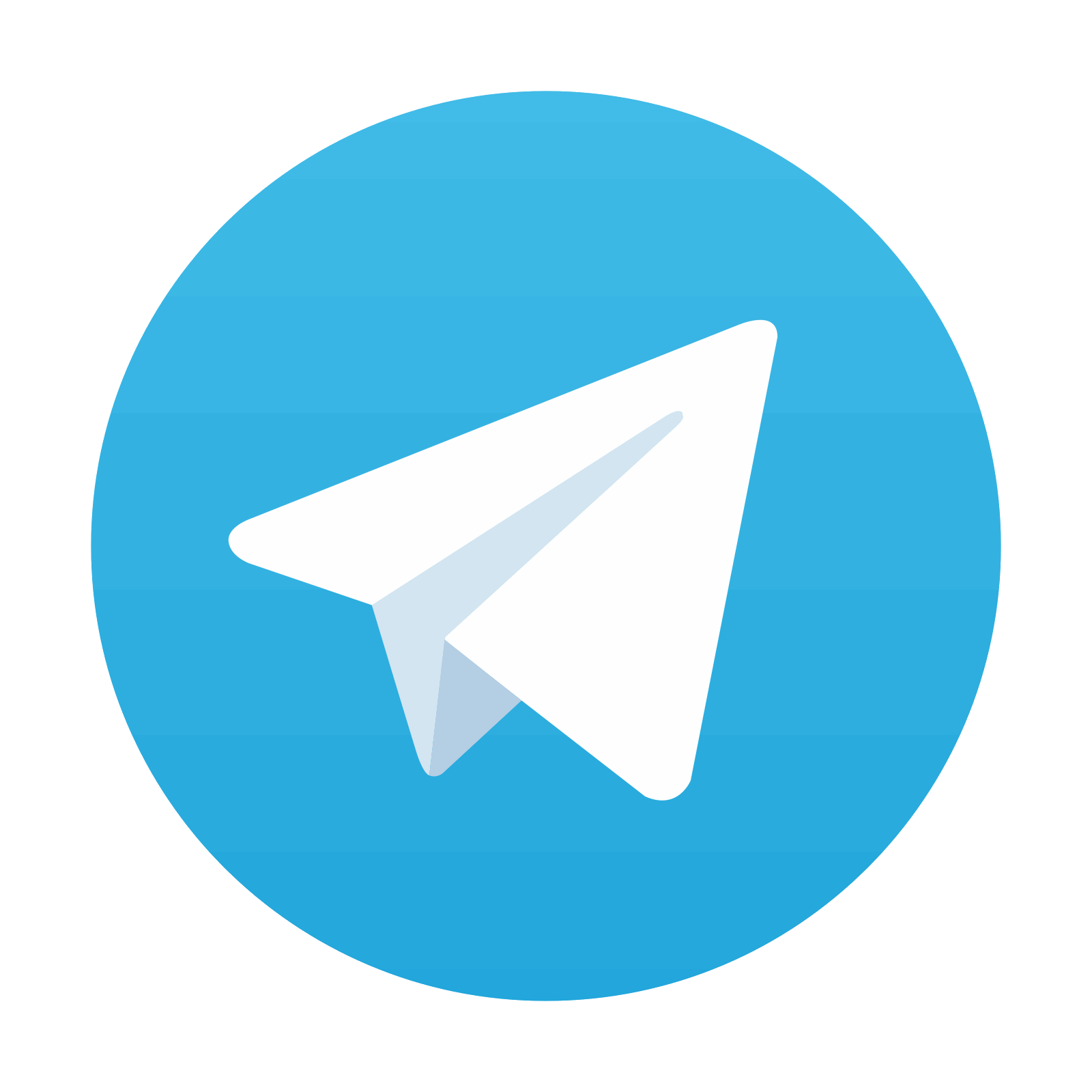
Stay updated, free articles. Join our Telegram channel

Full access? Get Clinical Tree
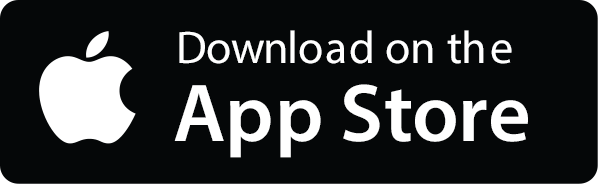
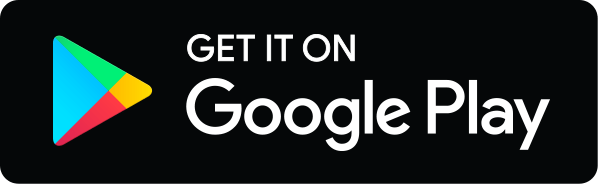