Summary by Thomas J. R. Beveridge, PhD
3
Based on “Principles of Addiction Medicine” Chapter by Thomas J. R. Beveridge, PhD, and David C. S. Roberts, PhD
PRIMER ON NEUROANATOMY
The structures most often mentioned in the context of drug abuse are closely associated with the limbic system, lateral hypothalamus, basal ganglia, and frontal cortical regions.
The limbic system has been characterized as highly interconnected, phylogenetically older regions of the forebrain that appear to form the only major route for information transfer between the neocortex and the hypothalamus. The main structures denoted by “the limbic system” are the limbic lobe (subcallosal area, cingulate, and parahippocampal gyri), amygdala, hippocampus, parts of the basal ganglia, anterior thalamic nucleus, parts of the hypothalamus, the habenula, and olfactory cortex (Fig. 3-1). The structures associated with the limbic system (such as the hypothalamus, hippocampus, and amygdala) are essential not only for learning and memory but also for the emotional context and the affective response to learned associations.
FIGURE 3-1. Regions of the human brain associated with the limbic system, which includes a loop of cortex extending from the subcallosal region through the cingulate cortex to the parahippocampal gyrus. Also shown are the hippocampal formation, septum, amygdala, and mammillary bodies.
Many drugs of abuse have their sites of action within the limbic system, and the neurochemistry within these structures is altered during the addiction process. This may help explain why decisions surrounding drug seeking and drug taking seem to be driven more by emotion and instinct rather than by logic.
The basal ganglia are traditionally thought of as a motor system; however, the idea that this system deals only with motor function while the limbic system deals with reinforcement and emotion is oversimplified. As more is learned about how the basal ganglia and limbic system communicate, it is becoming increasingly clear that the two systems are jointly involved in coordinating motivated behavior. The largest mass associated with the basal ganglia is the striatum (caudate–putamen). The dorsal portion has long been considered part of the basal ganglia, while the ventral striatum (also called the accumbens) is considered to be part of the limbic system. Mogenson famously described the accumbens as “the place where motivation is translated into action.”
The prefrontal cortex (PFC) is thought to be the “hub” of executive function in the brain. The PFC in humans is subdivided into three main regions: (a) the orbitofrontal cortex and the ventromedial areas (vm-PFC), which are thought to be involved in processing reward; (b) the dorsolateral prefrontal cortex (dl-PFC), more broadly involved in decision making; and (c) the anterior and ventral cingulate cortex, which helps to control whether or not a particular behavior will be performed, and to what intensity.
NEUROANATOMY OF DRUG REINFORCEMENT
“Site of action” is a pharmacologic concept that defines the access point for a drug to produce a specific response. If that response is defined behaviorally (e.g., anorexic, convulsant, antidepressant effect), then the site of action identifies the receptors and brain regions responsible for that particular behavioral response. In vitro experiments have shown that many drugs of abuse, particularly psychostimulants, interact with dopamine, noradrenaline, and serotonin (DA, NA, and 5-HT) transporters. Therefore, psychostimulant drugs act as indirect agonists everywhere these transmitters are found. The projections from DA, NA, and 5-HT cell bodies are extensive—indeed, there is hardly a brain region that is not innervated by at least two of the monoamines. Given that psychostimulants have an effect at the terminal regions of each of these systems, every area of the brain would be expected to be affected to some extent by an injection of cocaine or amphetamine. It has been a considerable challenge, therefore, sorting out what transmitter in which particular area produces toxic effects and adverse reactions on the one hand and pleasurable or positive reinforcing effects on the other. Much of these data has been gleaned from preclinical studies of drug reinforcement.
Psychostimulants
Cocaine binds to dopamine, noradrenaline, and serotonin (DA, NA, and 5-HT) transporters and blocks the reuptake of these neurotransmitters. Amphetamine acts additionally as a releasing agent. Both of these actions result in an increased concentration of monoamine neurotransmitters in the synapse.
Figure 3-2 demonstrates how the origins and projections of the main neuronal mass are associated with NA, 5-HT, and DA. All start in the brainstem and project upward. The catecholaminergic NA fibers start in the locus coeruleus and spread diffusely. The DA system is more contained—originating in the ventral tegmental area (VTA) and the substantia nigra.
FIGURE 3-2. Schematic diagram illustrating the distribution of the main central neuronal pathways containing noradrenaline (A), dopamine (B), and serotonin (C). The location of cell bodies of origin is indicated by circles with the projections indicated by arrows.
The extensive reach and overlap of the DA, NA, and 5-TH systems throughout the brain make research complex. While for many years, it was thought that drug use and addiction were confined to humans, and thus attributed to the neocortex, subsequent research on animals demonstrated that self-administration of drugs will occur in other mammalian species such as rat, dog, cat, rabbit, and nonhuman primates. This supports neuroanatomical findings that addiction arises in subcortical, evolutionary residual regions of the brain—that is, limbic and brainstem areas.
This anatomical understanding may help us to understand the complex nature of reinforcement—while patients may describe the alterations in consciousness associated with a specific substance as the “reason” for ongoing use, the mechanics behind use, abuse, and addiction are more complex and involve subcortical, unconscious factors.
Dopamine plays a central role in reward and drug addiction. Studies by Roy Wise demonstrated that treatment with DA antagonists produced an increase in cocaine or amphetamine intake in animals previously trained to self-administer these drugs. The use of the dopaminergic neurotoxin 6-OH-DA has been a useful tool in highlighting the role of DA. Removing the DA input to the NAcc (nucleus accumbens—now called the ventral striatum)—or destroying the DA cell bodies in the VTA resulted in diminution or abolition of cocaine self-administration in animals. Denervation of NA systems to the entire forebrain had no effect. The data demonstrate that the DA projects from the VTA to limbic areas are critical for the reinforcing effects of cocaine and amphetamines.
Both ablation and stimulation studies confirm that the striatum is responsible for motor behavior: the ventral area for locomotion and the dorsal for stereotypy. The appearance of parkinsonian side effects of neuroleptics confirms the involvement of DA in motor pathways involved in stereotypic repetitive motor behaviors. Any behavior can become reinforced, and the more frequently an action occurs, in the setting of drug use, the more likely it will become stereotyped. As such behaviors increase, others are excluded—thus limiting the behavioral range of the animal. In the case of a person with addiction, the frequent behaviors associated with drug seeking and drug taking become repetitive and ritualistic.
The great body of research showing VTA-DA neurons’ role in drug use and with primary reinforcing stimuli such as food, drinking, and sex has led to the term “Mesolimbic Reward Pathway.” Interestingly, there is no direct connection between this region and visual, auditory, or somatosensory systems. It appears instead that the VTA is a part of, and receives inputs from, a widespread collection of neurons that belong to the “isodendritic core.” This system is a network of neurons stretching from the brainstem to telencephalon. It would appear that this network serves an integrative function and responses to changes in the environment that are biologically significant. At present, it remains unclear whether the VTA-DA neurons respond differentially than others in the network and whether they are specifically activated by reward or more generally by any other important stimulus.
Opioids
Opioid receptors are expressed throughout the brain, especially in limbic and limbic-related structures. There are three different types of G protein–coupled opioid receptors: μ, κ, and δ, which are acted on by both endogenous and exogenously applied opioids. Selective μ agonist drugs, such as morphine, heroin, and most clinically used opioid analgesics, produce analgesia, euphoria, respiratory depression, emesis, and antidiuretic effects. Selective κ agonist drugs, such as the experimental compounds ethylketazocine and bremazocine, produce analgesia, dysphoria, and diuretic effects, but no respiratory depression. There is less known about the direct role of δ receptors. Agonists at μ receptor are more likely to have abuse liability than κ agonists.
Almost all that is known about the neurobiology of opioid reinforcement is derived from animal models. Three approaches have been used to investigate the involvement of various brain regions in opioid reward: (a) intracerebral self-administration of opioid agonists, (b) blockade of IV heroin self-administration by intracerebral injections of opioid antagonists, and (c) disruption of IV heroin self-administration by lesions. Generally, the focus has been on areas associated with the mesolimbic DA system (ventral striatum and VTA). Self-administration of drugs directly into various brain regions would seem to be the most straightforward test of their involvement in reinforcement processes; however, the procedures have a number of technical problems that limit their appeal. The main areas supporting opioid self-administration are the ventral striatum, NAcc, and VTA—with the latter being the most responsive. Interestingly, while injection of μ and δ opioid agonists into the VTA is strongly reinforcing, injection of antagonist into the VTA has little effect. This is especially surprising given that intracranial injections of antagonists into other areas of the brain can produce strong and predictable reactions.
When systemic naloxone is given, a compensatory increase in opioid self-administration is noticed. Similarly, increase in IV heroin self-administration is noticed when low-dose opioid antagonists are injected into the NAcc, periaqueductal gray, stria terminalis, and lateral hypothalamus, but not the PFC.
Lesion studies offer further support of the key role played by the NAcc in opioid reinforcement—kainic acid injected into the NAcc correlated with impaired heroin self-administration, yet did not have this effect when injected into other areas. Further studies suggest that the NAcc core is far more responsive than the shell, viz., opioid self-administration. Similar site-specific effects have been found when β-FNA (an irreversible opioid antagonist) is injected into the caudal, but not rostral NAcc.
The role of the mesolimbic DA system in opioid reinforcement is unclear. DA antagonists reduced self-administration of cocaine but not of heroin, and opioid antagonists reduce heroin but not cocaine use. However, it has been shown that opioids indirectly affect DA cell firing through inhibition of GABA interneurons in the VTA. This disinhibition can result in enhanced DA release in the NAcc. Heroin self-administration increases DA in the accumbens, and this has been argued to be the mechanisms of action for heroin reinforcement. It appears that DA innervation to the VTA is very important to opioid reinforcement, while altering such innervation to the NAcc has little effect. In contrast, reducing DA innervation to the NAcc results in dramatic reduction in cocaine reinforcement.
Cannabinoids
Two cannabinoid receptors (CB1 and CB2) have been identified. Both are G protein–coupled receptors and function to inhibit adenylate cyclase. They are acted on by endogenous cannabinoids and exogenous activators such as marijuana. There remains limited understanding about the reinforcing neuroanatomy of cannabinoids—this being due to difficulties in study designs with inhaled marijuana. The use of IV Δ9-tetrahydrocannabinol for self-administration studies on animals and the use of synthetic cannabinoid agonists have shown some reinforcing effects. It appears that both opioid and DA mechanisms may interact with cannabinoid reinforcement.
NEUROANATOMY OF DRUG ADDICTION
The research questions that can be addressed by using animal models are necessarily different than those that can be asked with human subjects. The former allow for invasive interventions, surgical manipulations not possible with humans, while the latter provides insight into the uniquely human aspects of addiction. Addiction is a disease that lives in the real world and thus encompasses many different facets, such as polydrug use, comorbidity with other disorders, predisposition, drug use history, and environmental context. Thus, the literature on human drug abuse offers quite different insights.
The neuroanatomy of addiction in human subjects has been studied using positron emission tomography (PET) and functional magnetic resonance imaging (fMRI). PET uses a radioisotope that is introduced into the body and binds to specific receptors, transporters, and enzymes. Specific ligands can be visualized, thereby offering insights into drug distribution and changes in receptor mechanism in vivo. fMRI offers much greater temporal resolution. Changes in the fMRI signal can be assessed on the order of seconds rather than minutes, making it possible to detect metabolic changes associated with transient cognitive demands or craving states.
Volkow et al. used PET to demonstrate decreased relative cerebral blood flow in the PFC of chronic cocaine users and metabolic changes varying with time from last use (increased in the 1st week of withdrawal). PET has also been used to show high cocaine binding in the corpus striatum in non–drug-using human subjects and to show that striatal dopamine D2 receptor binding is reduced in cocaine, heroin, and methamphetamine abusers and also in alcohol dependence. This area of work is in good concordance with nonhuman primate PET studies showing decreased D2 receptor availability in animals that are more susceptible to the reinforcing aspects of cocaine.
fMRI studies have demonstrated increased activity in the NAcc and decrease in the amygdala in cocaine users during cravings and increase in activity in the ventral tegmentum (VTA and substantia nigra), pons, basal forebrain, caudate, and cingulate that correlated with self-reported feelings of “rush” following cocaine use. Drug users also show increases in brain activity in limbic areas and the PFC following the presentation of drug-associated cues (such as pictures of drugs and drug paraphernalia) when compared with nondrug users and decreased responsiveness when presented with nondrug reinforcers (e.g., sexually evocative cues).
One brain area, the insula, has been recently recognized as having an essential role in the detection of interoceptive cues. These cues can also provoke powerful cravings for drugs and are a key component in the addiction process. A recent study by Bechara et al. discovered that nicotine-dependent patients with lesions to their insula reported disruption of smoking at a far greater frequency than did patients with lesions to other brain areas. Furthermore, smokers who acquired insula damage were more likely to quit smoking easily and immediately and to remain abstinent. Remarkably, drug-associated cues can produce limbic activation in cocaine users even when these stimuli are not consciously perceived. Childress et al. presented stimuli for only 33 ms. Although this short presentation was too brief for the image to be correctly identified, the drug-related stimuli nonetheless produced a strong increase in activity in the ventral pallidum and amygdala. The intensity of this response strongly predicted the magnitude of the subject’s affective response when later shown visible versions of the same cues. These data suggest that drug cues can stimulate drug craving even before there is conscious awareness. Childress et al. speculate that “by the time the motivational state is experienced and labeled as conscious desire, the ancient limbic reward circuitry already has a running start.” Medical imaging technology has thus provided concrete evidence of limbic involvement in drug craving that fits well with the wealth of evidence for animal studies.
KEY POINTS
1. To understand the neuroanatomy and psychopharmacology of addiction, we must understand the normal processes and structures involved in motivation, reward, decision making, and impulse control.
2. From here, we can examine the compulsivity, excessive consumption, and the resultant harm, which are central to addiction.
REVIEW QUESTIONS
1. Which part of the brain is most associated with the affective response to learned associations?
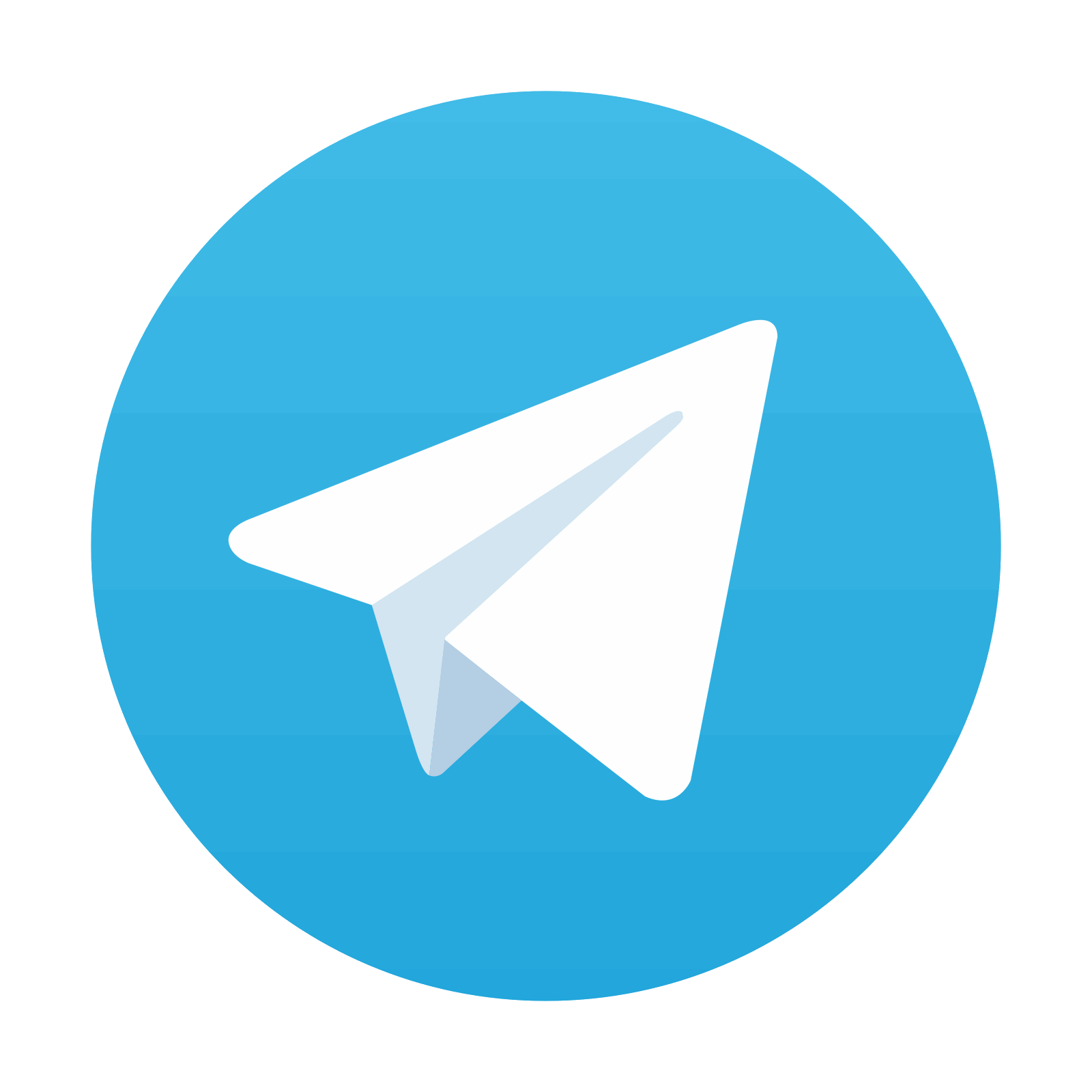
Stay updated, free articles. Join our Telegram channel

Full access? Get Clinical Tree
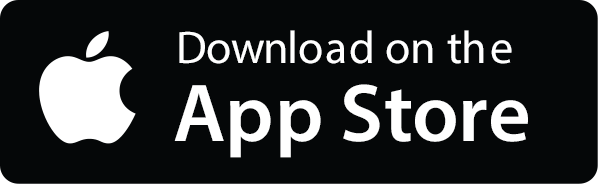
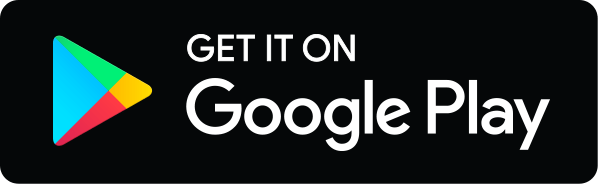
