Thus, less than 2% of all the mutant alleles in the population are in affected homozygotes and would therefore be exposed to selection in the absence of effective treatment.
Reduction or removal of selection against an autosomal recessive disorder by successful medical treatment (e.g., as in the case of PKU [see Chapter 12]) would have just as slow an effect on increasing the gene frequency over many generations. Thus as long as mating is random, genotypes in autosomal recessive diseases can be considered to be in Hardy-Weinberg equilibrium, despite selection against homozygotes for the recessive allele. Thus the mathematical relationship between genotype and allele frequencies described in the Hardy-Weinberg law holds for most practical purposes in recessive disease.
Selection in Dominant Disorders.
In contrast to recessive mutant alleles, dominant mutant alleles are exposed directly to selection. Consequently, the effects of selection and mutation are more obvious and can be more readily measured for dominant traits. A genetic lethal dominant allele, if fully penetrant, will be exposed to selection in heterozygotes, thus removing all alleles responsible for the disorder in a single generation. Several human diseases are thought or known to be autosomal dominant traits with zero or near-zero fitness and thus always result from new rather than inherited autosomal dominant mutations (Table 9-4), a point of great significance for genetic counseling. In some, the genes and specific mutant alleles are known, and family studies show new mutations in the affected individuals that were not inherited from the parents. In other conditions, the genes are not known, but a paternal age effect (see Chapter 4) has been seen, suggesting (but not proving) that a de novo mutation in the paternal germline is a possible cause of the disorder. The implication for genetic counseling is that the parents of a child with an autosomal dominant but genetically lethal condition will typically have a very low risk for recurrence in subsequent pregnancies because the condition would generally require another independent mutation to recur. A caveat to keep in mind, however, is the possibility of germline mosaicism, as we saw in Chapter 7 (see Fig. 7-18).
TABLE 9-4
Examples of Disorders Occurring as Sporadic Conditions due to New Mutations with Zero Fitness
Disorder | Description |
Atelosteogenesis | Early lethal form of short-limbed skeletal dysplasia |
Cornelia de Lange syndrome | Intellectual disability, micromelia, synophrys, and other abnormalities; can be caused by mutation in the NIPBL gene |
Osteogenesis imperfecta, type II | Perinatal lethal type, with a defect in type I collagen (COL1A1, COL1A2) (see Chapter 12) |
Thanatophoric dysplasia | Early lethal form of skeletal dysplasia due to de novo mutations in the FGFR3 gene (see Fig. 7-6C) |
Mutation and Selection Balance in Dominant Disease.
If a dominant disease is deleterious but not lethal, affected persons may reproduce but will nevertheless contribute fewer than the average number of offspring to the next generation; that is, their fitness, f, will be reduced. Such a mutation will be lost through selection at a rate proportional to the reduced fitness of heterozygotes. The frequency of the mutant alleles responsible for the disease in the population therefore represents a balance between loss of mutant alleles through the effects of selection and gain of mutant alleles through recurrent mutation. A stable allele frequency will be reached at whatever level balances the two opposing forces: one (selection) that removes mutant alleles from the gene pool and one (de novo mutation) that adds new ones back. The mutation rate per generation, µ, at a disease locus must be sufficient to account for that fraction of all the mutant alleles (allele frequency q) that are lost by selection from each generation. Thus,
μ=sq

As an illustration of this relationship, in achondroplasia, the fitness of affected patients is not zero, but they have only approximately one fifth as many children as people of normal stature in the population. Thus their average fitness, f, is 0.20, and the coefficient of selection, s, is 1 − f, or 0.80. In the subsequent generation, then, only 20% of current achondroplasia alleles are passed on from the current generation to the next. Because the frequency of achondroplasia appears stable from generation to generation, new mutations must be responsible for replacing the 80% of mutant genes in the population lost through selection.
If the fitness of affected persons suddenly improved (e.g., because of medical advances), the observed incidence of the disease in the population would be predicted to increase and reach a new equilibrium. Retinoblastoma (Case 39) and other dominant embryonic tumors with childhood onset are examples of conditions that now have a greatly improved prognosis, with a predicted consequence of increased disease frequency in the population. Allele frequency, mutation rate, and fitness are related; thus, if any two of these three characteristics are known, the third can be estimated.
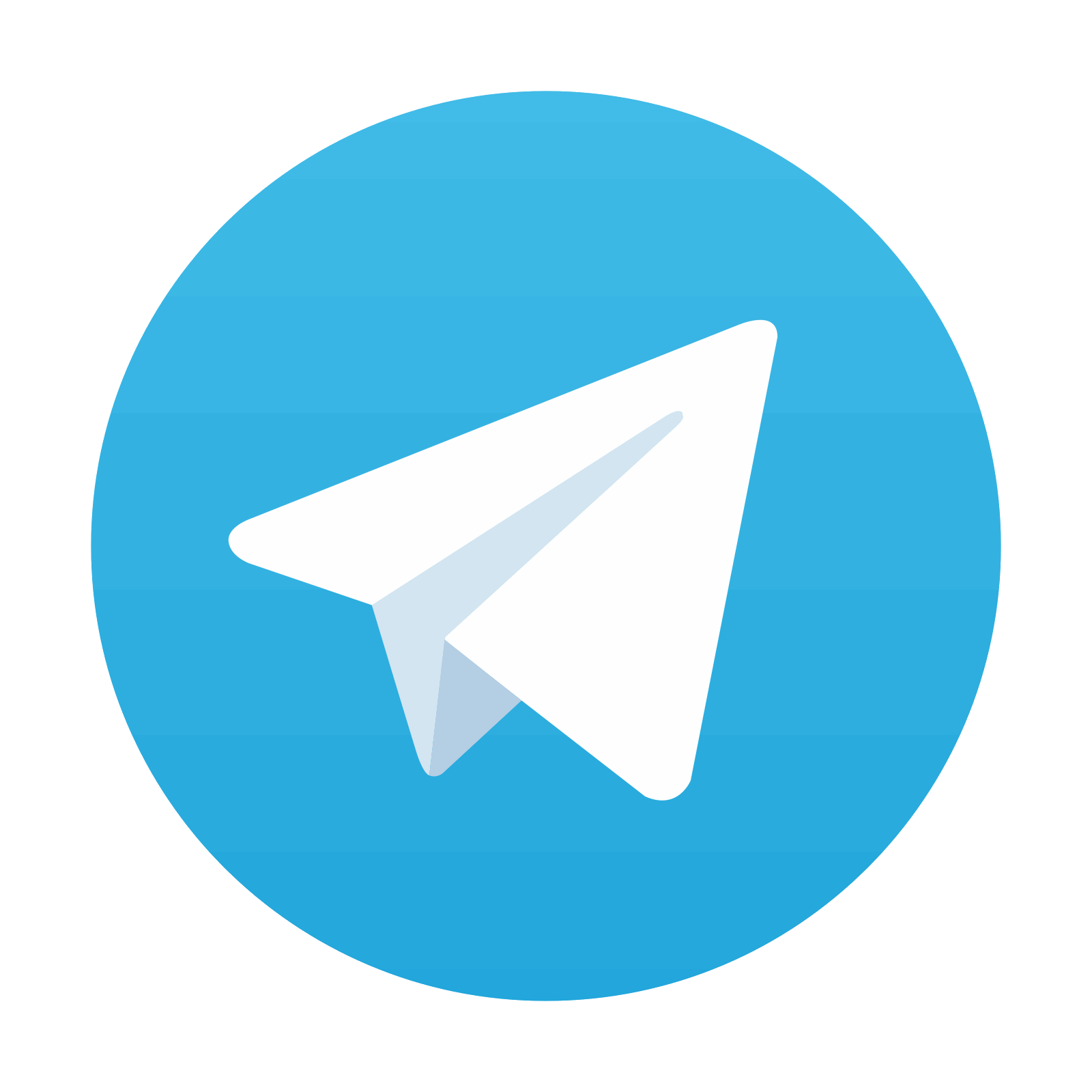
Stay updated, free articles. Join our Telegram channel

Full access? Get Clinical Tree
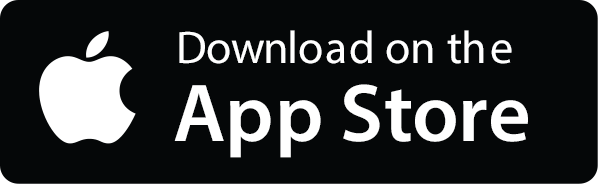
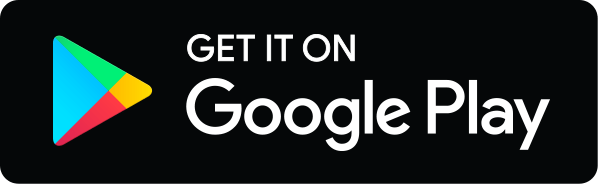