2.11.16 Synthesis and Reactivity of Heteroatom-Substituted Vinylzirconocene Derivatives and Hetarylzirconocenes
J. Bruffaerts and A. Vasseur
General Introduction
Alkenylmetals are versatile precursors for the incorporation of vinyl moieties through selective formation of carbon–carbon and/or carbon–heteroatom bonds. Several methods exist to provide almost any type of desired C(sp2)—metal species. Generally speaking, haloalkenes and haloarenes (oxidative metalation, metal–halogen process), alkenes and arenes (metal–hydrogen exchange), alkynes (hydrometalation, carbometalation), and ketones and aldehydes (Shapiro reaction) are the typical precursors to vinylmetals.[1] The extension to a broader range of substrates remains an ongoing challenge. Concurrently, there is a strong demand to develop new, mild, and stereoselective procedures toward stereodefined vinylmetals.
Amid sp2-organometallic derivatives, vinylzirconocenes (also referred to as alkenylzirconocenes) have drawn growing attention since their discovery in the 1970s. They have not been as popular as vinylmagnesium, vinylcopper, or even vinylzinc derivatives despite the unique features organozirconium chemistry offers in comparison to any other transition metal.[2] However, vinylzirconocene intermediates have rapidly proved their utility in synthesis.
Well-established procedures now allow synthetic chemists to access vinylzirconocenes from a broad range of heteroatom-substituted alkenes (▶ Scheme 1). The only negative element is the relative instability of arylzirconocenes obtained through this method, although hetarylzirconocenes are potentially stable. Alkoxy-, siloxy-, sulfanyl-, sulfoxy-, and sulfonyl-substituted alkenes are stereoselectively converted whereas the corresponding halides and carbamates generally react stereospecifically. So, on one hand, E-vinylzirconocenes are available even from mixtures of regioisomers; on the other hand, Z-vinylzirconocenes are trickier to obtain. Indeed, their preparation from vinyl carbamates only reaches a regioisomeric ratio of 85:15 in the best case (see ▶ Section 2.11.16.3.6). To the best of our knowledge, no reports have been published on the synthesis of vinylzirconocenes from Z-alkenyl halides.
As an extension to vinylzirconocene systems, the preparation of dienylzirconocenes has also been described from siloxydienes, dienyl sulfones, and nonconjugated alkenyl enol ethers (see ▶ Section 2.11.16.3.7). In the latter case, a tandem reaction combines allylic C—H bond activation and elimination of the alkoxy moiety.
2.11.16.1 General Preparation of Vinylzirconocene Derivatives
Concerning their preparation, vinylzirconocenes can be generated by numerous methods, summarized in ▶ Scheme 2. These methods can be grouped into four distinct categories: (i) hydrozirconation of alkynes with the Schwartz reagent, (ii) carbozirconation of terminal alkynes, (iii) insertion of a carbenoid equivalent, and (iv) oxidative addition–elimination from heteroatom-substituted alkenes with Negishi-type reagents (see also ▶ Scheme 1).

The first syntheses of vinylzirconocenes were performed by hydrozirconation of terminal alkynes as early as 1971[3] through the addition of chlorobis(η5-cyclopentadienyl)hydridozirconium(IV), first synthesized and characterized in 1969.[4,5] This zirconium hydride would be later known as the Schwartz reagent, as, from 1974 on, Schwartz greatly enhanced the popularity of this reagent in synthesis by publishing a series of pioneering papers.[6–11] Although the syn hydrometalation of alkynes has already been well established,[12] especially through the addition of boranes,[13] alanes,[14] and silanes,[15] hydrozirconation has gained popularity among the synthetic chemistry community. Indeed, the zirconium moiety easily undergoes transmetalation, which is not always true for boron-, aluminum-, or silicon-based intermediates.[16] Furthermore, hydrozirconation has been extended to cross-coupling reactions in one-pot procedures, and has recently been reviewed.[17,18] Further studies have extended the scope of hydrozirconation to heteroatom-substituted alkynes (boron,[19–24] iodide,[25,26] phosphorus,[27] silicon,[28–37] sulfur,[38–42] selenium,[29,43–48] and tellurium[43,49–51]) as well. Therefore, it is not surprising that hydrozirconation has now become a classical and useful synthetic transformation.
Only a few years after the discovery of the hydrozirconation reaction and its potential, the carbozirconation of alkynes was reported by Negishi[52] and later studies by Suzuki[53–55] and Nishihara[56] followed. It has been especially relevant for the preparation of zirconacyclopentadiene intermediates.[57] However, carbozirconation is less popular because it has largely been outshone by the many other well-established carbometalation processes.[1,58]
The formation of vinylzirconocenes through the insertion of carbenoid equivalents has been extensively studied by Whitby and co-workers.[59] This method requires the in situ preparation of the organozirconium species, typically by hydrozirconation of a terminal alkyne or alkene.
All of the methods described above are limited to the exclusive use of alkynes or alkenes as substrates. However, since 1995, heteroatom-substituted alkenes (halides, enol sulfonates, enol ethers, silyl enol ethers, sulfides, sulfoxides, sulfones, and carbamates) and arenes have been potential precursors of vinyl- and arylzirconocenes derivatives as well. These advances considerably expanded the scope of vinylzirconocene precursors, because they allow the selective cleavage of C(sp2)—heteroatom bonds, even of the relatively inert C—O and C—S bonds.
2.11.16.2 General Reactivity of Vinylzirconocene Derivatives
Vinylzirconocenes react in the presence of various electrophiles to provide polysubstituted alkenes. Their reactivity has already been well established through the hydrozirconation of alkynes. First of all, hydrolysis (or deuterolysis) typically in an acidic medium affords the corresponding alkene (▶ Scheme 3). Halogenolysis is another way to trap vinylzirconocene intermediates (▶ Scheme 3). Disulfide reagents also react with vinylzirconocenes (▶ Scheme 3).[60] Nonetheless, one major drawback of zirconium is its inability to create C—C bonds directly; however, transmetalation provides an effective solution to this problem. Because the Zr—C bond is relatively polarized due to the low electronegativity of the metal, the vinyl moiety is easily transferred to other metals (e.g., boron,[61,62] tin,[63] and zinc[64]) to react potentially in palladium- or nickel-catalyzed cross-coupling reactions (▶ Scheme 3). A series of examples of nucleophilic addition of vinylzirconocenes has also been reported through transmetalation in the presence of either stoichiometric or catalytic amounts of copper,[65–68] zinc,[69–74] or rhodium[75–78] salts (▶ Scheme 3). In addition to all these methods for the formation of C(sp2)—C(sp2) and C(sp2)—C(sp3) bonds, enamines have also been synthesized using 1,2-disubstituted vinylzirconocenes prepared by the hydrozirconation of alkynes (▶ Scheme 3).[79–81] The formation of C(sp2)—P bonds has been explored for the synthesis of various alkenylphosphanes (▶ Scheme 3).[82,83] It is important to note that all these methods do not generally suffer from isomerization of the double bond; stereodefined vinylmetal species therefore lead to stereodefined polysubstituted alkenes.
The impressive amount of research mentioned above has definitely established vinylzirconocene derivatives as versatile intermediates in synthesis.
2.11.16.3 Preparation of Vinylzirconocene Derivatives from Heteroatom-Substituted Alkenes
All transformations of heteroatom-substituted alkenes into vinylzirconocenes use low-va-lent zirconocenes, mostly the Negishi reagent 1. It isusually prepared in situ by treating commercially available chlorobis(η5-cyclopentadienyl)zirconium(IV) with 2 equivalents of butyllithium to afford the active zirconocene 1 by elimination of butane (▶ Scheme 4).[84] Formally, the Negishi reagent 1, which is also represented by formula 2, can be viewed as a 16-electron bis(η5-cyclopentadienyl)zirconium(II) complex. This species should be formed at low temperature (typically from −78 to −50 °C), before further thermal decomposition occurs.[85] The reactivity of this reagent has already been largely reviewed and discussed.[2,86,87]
In the presence of an alkene, the Negishi zirconocene 1 affords the corresponding zirconcyclopentane 3 (▶ Scheme 5).[1] After elimination of butene, the formed zirconacyclopropane intermediate 4 can eventually eliminate the leaving group to reform the C=C bond. Alternatively, intermediate 3 can directly fragment to afford the vinylzirconocene derivative 5. However, the existence of such species still remains unproven and detailed mechanistic information about this transformation is missing. Depending on the nature of the heteroatom substituent, this transformation is either stereospecific in the case of halides and carbamates, or stereoselective in the case of enol ethers, silyl enol ethers, sulfides, sulfoxides, and sulfones. Dienyl systems, involving either conjugated or remote dienes, can also be used.
Scheme 5 General Proposed Mechanism for the Oxidative Addition–Elimination of the Negishi Reagent Applied to Heteroatom-Substituted Alkenes[1]

2.11.16.3.1 Method 1: From Alkenyl Halides
In 1995, the very first report on the preparation of vinylzirconocenes from heteroatom-substituted alkenes, by Takahashi and co-workers, showed that 1,1-disubstituted alkenyl chlorides or bromides 6 can undergo an oxidative addition–elimination process in the presence of Negishi-type reagents to provide the corresponding 1,1-disubstituted vinylzirconocenes 7 (▶ Scheme 6).[88] Those intermediates were characterized by NMR analysis. The crystal structure of vinylzirconocene 7 (R1 = Me; R2 = η5–tert-butylcyclopentadienyl; X = Cl) was also determined. This method represents a convenient access to 1,1-disubstituted alkenylzirconocene derivatives, which are not accessible by hydrozirconation (only affording 1,2-di- or 1,1,2-trisubstituted vinylzirconocenes). The procedure is compatible with a copper transmetalation to perform an SN2′ reaction with allyl chloride to provide 2-benzylpenta-1,4-diene (8, R1 = Bn; R3 = CH2CH—CH2). Exchange with zinc salts allows a Negishi cross-coupling reaction in the presence of iodobenzene or benzoyl chloride (▶ Scheme 6).
Scheme 6 Preparation of Vinylzirconocenes from 1,1-Disubstituted Chloro- and Bromoalkenes and Their Subsequent Cross Coupling[88]

R1 | X | R2 | Yielda (%) | Ref |
Bn | Cl | Cp | 98 | [88] |
Me | Cl | Cp | 80 (54) | [88] |
Me | Br | Cp | 93 (73) | [88] |
(CH2)4Me | Cl | Cp | 70 | [88] |
![]() | Cl | Cp | 92 (61) | [88] |
Me | Cl | ![]() | 83 (62) | [88] |
a NMR yields; isolated yields are given in parentheses. |
R1 | X | R2 | R3 | Z | Conditions | Yielda (%) | Ref |
Bn | Cl | Cp | CH2CH=CH2 | Cl | CuCl/LiCl | 83 | [88] |
Bn | Cl | Cp | Ph | Cl | ZnCl2, Pd(PPh3)4 (5 mol%) | 78 (56) | [88] |
(CH2)4Me | Cl | Cp | Bz | Cl | ZnCl2, Pd(PPh3)4 (5 mol%) | 63 (42) | [88] |
a GC yields; isolated yields are given in parentheses. |
With respect to the stereochemical outcome of the zirconocene-mediated dehalogenation of vinyl halides, the Marek group showed that successive treatment of a mixture of 2-bromo-1-phenylethene regioisomers with Negishi reagent 1 and iodine leads to the exact same E/Z ratio. Thus, this transformation is stereospecific, proceeding with retention of the original configuration of the alkene (▶ Scheme 7).[89]
The zirconocene-mediated dehalogenation was applied to vinyl chloride 9 and combined with a cross-coupling reaction in the presence of silver(I) phosphate and catalytic amounts of palladium(II) acetate and tri(2-tolyl)phosphine (▶ Scheme 8).[90] This C—C bond formation represents a key step toward the convergent total synthesis of (–)-diazonamide A and B.
Scheme 8 Zirconocene-Mediated Dehalogenation Reaction as a Key Step in the Total Synthesis of (–)-Diazonamide A and B[90]

1,1-Disubstituted Vinylzirconocenes 7; General Procedure:[88]
A 1.6 M soln of BuLi in hexane (1.25 mL, 2 mmol) was added to a soln of Zr(Cp)2Cl2 (292 mg, 1 mmol) in THF (5 mL) at −78 °C and the soln was stirred for 1 h. After removal of the cooling bath, an alkenyl halide 6 (1 mmol) was added and the mixture was stirred for another hour.
Bis(η5–tert-butylcyclopentadienyl]chloro(prop-1-en-2-yl)zirconium(IV) [7, R1 = Me; R2 = η5–tert-Butylcyclopentadienyl; X = Cl]; Typical Procedure:[88]
A 1.6 M soln of BuLi in hexane (2.5 mL, 4 mmol) was added to a soln of bis(tert-butyl-η5-cyclopentadienyl)chlorozirconium(IV) (813 mg, 2 mmol) in Et2O (10 mL) at −78 °C and the soln was stirred for 1 h. The mixture was warmed to 0 °C, and stirring was continued for 3 h at 0 °C to complete the alkylation. To this mixture was added 2-chloropropene (6, R1 = Me; X = Cl; 170 μL, 2 mmol). The mixture was stirred overnight at rt. LiCl was removed by a frit filtration. Removal of the solvent under reduced pressure followed by recrystallization (hexane) afforded the product as light yellow crystals; yield: 539 mg (62%).
Prop-2-ene-1,2-diyldibenzene (8, R1 = Bn; R3 = Ph); Typical Procedure:[88]
A 1.6 M soln of BuLi in hexane (1.25 mL, 2 mmol) was added to a soln of Zr(Cp)2Cl2 (292 mg, 1 mmol) in THF (5 mL) at −78 °C and the soln was stirred for 1 h. After removal of the cooling bath, (2-chloroallyl)benzene (6, R1 = Bn; X = Cl; 153 mg, 1 mmol) was added and the mixture was warmed to rt and stirred for 3 h. After addition of iodobenzene (204 mg, 1 mmol), anhyd ZnCl2 (136 mg, 1 mmol), and Pd(PPh3)4 (58 mg, 0.05 mmol), the mixture was stirred at rt for 6 h. The reaction was quenched with 3 M aq HCl and the mixture was extracted with hexane (15 mL). The combined organic layers were washed with sat. aq NaHCO3 and dried (MgSO4). Kugelrohr distillation (1 Torr) afforded the product; yield: 109 mg (56%).
2.11.16.3.2 Method 2: From Aryl Halides
Selective dehalogenation reactions are a challenging topic in synthesis.[91] Richmond and co-workers reported the selective monodefluorination of perfluoroaryl substrates using zirconocene reagents in 1996.[92,93] Similarly, Takahashi and co-workers have reported that the Negishi reagent 1 can be used for the dehalogenation of aryl halides (▶ Scheme 9).[94]
Ar1 | X | Time (h) | Yield (%) | Ref |
Ph | Br | 12 | 94 | [94] |
2-MeOC6H4 | Br | 1 | 90 | [94] |
2-naphthyl | Cl | 6 | 96 | [94] |
2-ClC6H4 | Br | 1 | 98 | [94] |
![]() | Br | 3 | 98 | [94] |
In addition, the same type of reaction using a catalytic amount of chlorobis(η5-cyclopentadienyl)zirconium(IV) gives the best yields in the presence of butylmagnesium chloride, amongst the Grignard reagents tested, for the dehalogenation of aryl halides 10 to provide arenes 11 (▶ Scheme 10).[94]
Scheme 10 Dehalogenation of Aryl Halides Using a Catalytic Amount of a Zirconium Salt and Butylmagnesium Chloride[94]

Ar1 | X | Time (h) | Yield (%) | Ref |
2-ClC6H4 | Br | 6 | 94 | [94] |
4-ClC6H4 | Br | 6 | 97 | [94] |
4-ClC6H4 | I | 1 | 98 | [94] |
4-BrC6H4 | I | 1 | 98 | [94] |
4-Tol | I | 3 | 95 | [94] |
2-MeOC6H4 | Br | 12 | 98 | [94] |
4-HOC6H4 | Br | 6 | 94 | [94] |
4-HOC6H4 | I | 3 | 98 | [94] |
1-naphthyl | Cl | 6 | 93 | [94] |
2-naphthyl | Cl | 20 | 98 | [94] |
![]() | Br | 1 | 94 | [94] |
3-Br-4-MeOC6H3 | Br | 3 | 72a | [94] |
a 1-Bromo-2-methoxybenzene was also obtained in 22% yield. |
This dehalogenation method seems obsolete compared to other dehalogenation processes,[91] although, interestingly, it shows an almost perfect selectivity concerning the deiodination of 4-bromo-1-iodobenzene (10, Ar1 = 4-BrC6H4; X = I) and the monodebromination of 2,3-dibromothiophene (10, Ar1 = 2-bromo-3-thienyl; X = Br). But this reaction fails for the selective debromination of 2,4-dibromoanisole (10, Ar1 = 3-Br-4-MeOC6H3). Regarding its scope, functional groups such as alcohols, ethers, and thiophenes are tolerated.
However, deuterolysis of bromobenzene pretreated with the Negishi-type zirconocene does not give the expected deuterated benzene. A possible explanation is illustrated in ▶ Scheme 11.[94] The arylzirconocene 12 cannot undergo a bromide–alkyl exchange in the presence of butyllithium or butylmagnesium chloride. Instead, the arylzirconocene undergoes a hydrogen shift, affording benzene. So, no stable arylzirconocene intermediate is formally generated through this method.
Hetaryl halides have also been subjected to zirconocene oxidative addition with Negishi reagent 1 (▶ Scheme 12). In sharp contrast to what can be observed with arylzirconocenes, it appears that most hetarylzirconocene derivatives are stable.[95] In the deuterolysis of some representative heteroaromatic halides, 2-chloroquinoline (13) and 5-bromo-2,2′-bithiophene (15) give the deuterated adducts 14 and 16 in high conversion (80 and 90%, respectively), whereas 1-chloroisoquinoline (17) only provides 25% conversion under same conditions (▶ Scheme 12). The reasons why some hetarylzirconocenes are stable and some are not are still unclear, but the electronic effect of the adjacent heteroatom definitely plays a key role in avoiding this hydrogen shift.
The in situ formed hetarylzirconocene derivatives 19, obtained from hetaryl halides 18, can be used in a subsequent cross-coupling reaction with aryl halides in the presence of copper(I) chloride and a catalytic amount of tetrakis(triphenylphosphine)palladium(0) to create a Chetaryl—Caryl bond efficiently (▶ Scheme 13). The yields of the products 20 are generally very good, although some discrepancies exist depending on the relative position of the halide on the heteroaromatic ring.
Ar1 | X | Ar2 | Z | Yield (%) | Ref |
2-quinolyl | Cl | 4-MeO2CC6H4 | Br | 84 | [95] |
2-quinolyl | Cl | 3-EtO2CC6H4 | Br | 91 | [95] |
2-quinolyl | Cl | 4-NCC6H4 | Br | 95 | [95] |
2-quinolyl | Cl | 4-AcC6H4 | Br | 85 | [95] |
1-isoquinolyl | Cl | 4-NCC6H4 | Br | 32 | [95] |
2-pyridyl | Br | 4-NCC6H4 | Br | 56 | [95] |
![]() | Cl | 4-NCC6H4 | Br | 49 | [95] |
2-thienyl | Br | 2-pyridyl | Br | 93 | [95] |
2-thienyl | Br | 2-quinolyl | Cl | 82 | [95] |
![]() | Br | 4-NCC6H4 | Br | 98 | [95] |
Arenes 11; General Procedure:[94]
To a mixture of an aryl halide 10 (1 mmol) and Zr(Cp)2Cl2 (0.1 mmol) in anhyd THF (5 mL) was slowly added BuMgCl (3 mmol) at −78 °C. The mixture was stirred at rt for several hours (see ▶ Scheme 10) and monitored by GC.
Quinoline-2-d (14); Typical Procedure:[95]
To a dry, argon-flushed, 25-mL, three-necked, round-bottomed flask equipped with a condenser and a magnetic stirrer bar were charged Zr(Cp)2Cl2 (702 mg, 2.4 mmol), THF (3 mL), and Et2O (3 mL). The mixture was cooled to −78 °C with a dry ice/iPrOH bath. A 1.6 M soln BuLi in hexane (3 mL, 4.8 mmol) was added dropwise and the mixture was stirred for 1 h at −78 °C. A soln of 2-chloroquinoline (13; 327 mg, 2 mmol) in THF (1.5 mL) and Et2O (1.5 mL) prepared in a separate dry, argon-flushed, 25-mL, round-bottomed flask was transferred via syringe to the mixture at −78 °C. After the addition, the dry ice bath was removed and the mixture was heated at 50 °C for 45 min. All the 2-chloroquinoline (13)had been consumed as monitored by GC analysis of the mixture. The mixture was allowed to cool to rt. AcOD (0.5 mL) was added and the mixture was stirred at rt for 3 h. After workup, the deuterated quinoline was isolated and purified by column chromatography (silica gel, CH2Cl2/EtOAc 10:1); yield: 230 mg (89%).
Methyl 4-(Quinolin-2-yl)benzoate (20, Ar1 = 2-Quinolyl; Ar2 = 4-MeO2CC6H4); Typical Procedure:[95]
A dry, argon-flushed, three-necked, 25-mL round-bottomed flask equipped with a condenser and a magnetic stirrer bar was charged with Zr(Cp)2Cl2 (702 mg, 2.4 mmol), THF (3 mL), and Et2O (3 mL). The mixture was cooled to −78 °C in a dry ice/iPrOH bath. A 1.6 M soln of BuLi in hexanes (3.0 mL, 4.8 mmol) was added dropwise and the mixture was stirred for 1 h at −78 °C. A soln of 2-chloroquinoline (18, Ar1 = 2-quinolyl; X = Cl; 327 mg, 2 mmol) in THF (1.5 mL) and Et2O (1.5 mL) prepared in a separate dry, argon-flushed, 25-mL round-bottomed flask was transferred via syringe to the mixture at −78 °C. After the addition, the dry ice bath was removed and the mixture was heated at 50 °C and stirred for 45 min. All the 2-chloroquinoline (18, Ar1 = 2-quinolyl; X = Cl) had been consumed, as monitored by GC analysis of the mixture. Methyl 4-bromobenzoate (215 mg, 1 mmol), Pd(PPh3)4 (58 mg, 0.05 mmol), and CuCl (99 mg, 1 mmol) were added all at once. The mixture was heated in an oil bath set at 50 °C for 48 h. GC analysis showed that all methyl 4-bromobenzoate had been consumed. The mixture was allowed to cool to rt. H2O (10 mL) was added and the mixture was filtered. The solid was washed with EtOAc (2 × 5 mL). The filtrate was extracted with CH2Cl2 (3 × 20 mL) and the combined organic layers were dried (MgSO4), filtered, and concentrated under reduced pressure. The crude product was purified by column chromatography (silica gel, hexane/EtOAc 5:1) to provide the product as a tan solid; yield: 218 mg (84%).
2.11.16.3.3 Method 3: From Enol Sulfonates
A C—O bond is cleaved in the oxidative addition–elimination transformation of 2,2-difluorovinyl 4-toluenesulfonate (21) (▶ Scheme 14).[96] This method represents an especially convenient way to access a stable, but tricky to prepare, 2,2-difluorovinylmetal species 22, which can then be used in cross-coupling reactions with aryl iodides to synthesize 1-aryl-2,2-difluoroethene derivatives 23.
Scheme 14 Synthesis of 2,2-Difluorovinylzirconocene Derivatives and Their Subsequent Cross Coupling with Aryl Iodides[96]

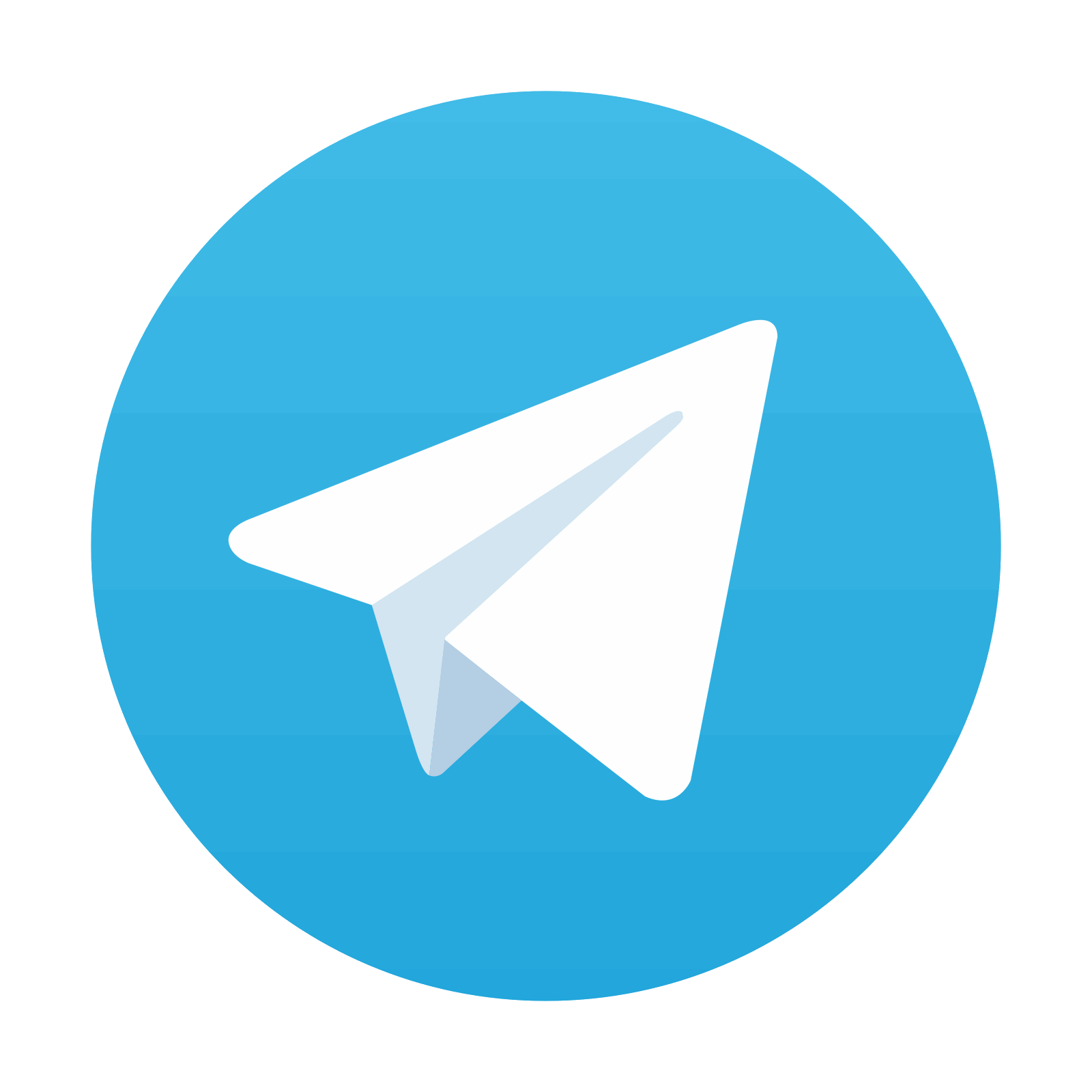
Stay updated, free articles. Join our Telegram channel

Full access? Get Clinical Tree
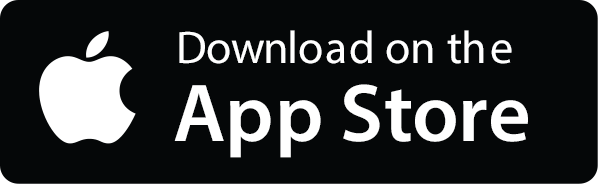
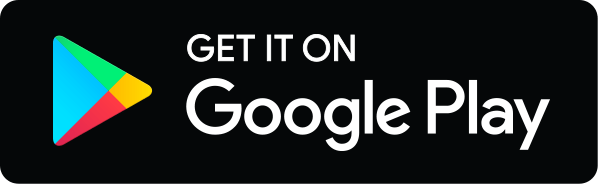