Fig. 5.1
Schematic illustration of binding specificities of the five PDGF isoforms and the structural organization of the PDGF receptors
PDGF-BB, and PDGF-AA dimers composed of the longer splice variant, includes a basic C-terminal retention signal mediating binding to cell surface and pericellular extracellular matrix and proteoglycans which restricts signaling to neighboring cells [10, 11]. PDGF-CC and PDGF-DD are both secreted as latent proteins which are activated after proteolytic cleavage of a regulatory N-terminal CUB domain [8].
The five PDGF ligands exert their biological effects through two structurally related tyrosine kinase receptors: PDGF alpha-receptors (PDGF-αR) and PDGF beta-receptors (PDGF-βR) (Fig. 5.1). Both these receptors are composed of an extracellular region with five Ig-like domains, a single transmembrane segment and an intracellular part with a split tyrosine kinase domain. Whereas the intracellular parts of the two receptors show a high degree of conservation, larger differences are observed in the extracellular part involved in ligand binding [8].
The different ligands vary in their receptor-binding profiles. Based on cell culture experiments, using purified ligand isoforms, it has been concluded that PDGF-AA, PDGF-AB, PDGF-BB, and PDGF-CC bind PDGF-αR and that PDGF-BB and PDGF-DD are high-affinity ligands for PDGF-βR [4, 8] (Fig. 5.1).
Cellular Responses, Receptor Activation, and Molecular Signaling Induced by PDGFs
Typical responses to PDGF stimulation in tissue culture settings include proliferation, directed migration, and contraction of collagen gels. The molecular basis for these cellular responses has been extensively characterized [4, 5].
Ligand-induced receptor dimerization is the crucial event in receptor activation. Receptor dimerization occurs through binding of one dimeric ligand to Ig domains 1–3 of two receptor molecules. Receptor dimers are also stabilized by Ig-domain-4-mediated receptor-receptor interactions. The ligand-induced receptor dimerization triggers autophosphorylation of tyrosine residues, which act as docking sites for SH2-domain-containing signaling molecules. These include adaptor proteins such as the p85 subunit of PI3K and the Ras-activating Grb2, as well as proteins with intrinsic enzymatic activity like c-Src and PLC-gamma. A third class of SH2-domain-containing proteins activated by PDGF receptors is the STAT transcription factor family. Mechanisms for negative regulation of PDGF receptor signaling include ligand-induced receptor internalization promoted by ubiquitination and dephosphorylation by tyrosine phosphatases.
Detailed cell biology studies indicate that different PDGFR dimers display differences with regard to their signaling activity. However, the physiological significance of these findings remains largely unknown. Genetic studies in mice have demonstrated normal phenotypes of mice expressing chimeric receptors composed of the extracellular domain of the PDGF-αR and the intracellular domain of PDGF-βR [12].
Developmental and Physiological Roles of PDGF
The roles of the PDGF system in development have been extensively studied in mouse models where the effects of knocking out different PDGF ligands and receptors have been analyzed (reviewed in [5]). Collectively these studies have identified PDGFRs as important regulators of mesenchymal cells involved in cross talk with adjacent epithelial or endothelial cells. A theme emerging from these studies is a pattern of paracrine signaling with ligands produced by the endothelial/epithelial cells that stimulate recruitment and proliferation of the PDGF receptor-expressing mesenchymal cells.
Developmental Roles of PDGF-αR
Organs with a particular PDGF-αR dependency include the lungs and the GI tract. These and other developmental roles of PDGF-αR have been extensively reviewed [5].
Knockout of PDGF-A leads to a failure of mesenchymal cell spreading into the walls of alveolar saccules of the lung and an emphysema-like phenotype [13]. Conversely, overexpression of PDGF-αR ligands results in perinatal death associated with lungs displaying a thickened mesenchyme compatible with hyper-proliferation of alveolar smooth muscle cell progenitors [14]. Paracrine PDGF-A/PDGF-αR signaling also controls formation of gastrointestinal villi [15]. PDGF-A knockout mice display misshapen gastrointestinal villi. This phenotype has been concluded to reflect a critical role for PDGF-αR -signaling in the renewal and migration of mesenchymal cells that normally line the basement membrane and control villi formation. Furthermore, PDGF-αR knockout mice also display hypoplasia in the mesenchymal compartment of the skin and kidney.
Other independent studies relying on overexpression of hyperactive variants of PDGF-αR have analyzed the roles of PDGF-αR in mesenchymal precursor cells with perivascular location. Notably, PDGF-αR hyperstimulation prevented adipogenic differentiation and instead promoted development toward a pro-fibrotic ECM secretory phenotype [16].
Together these findings suggest an important role of PDGF-αR signaling for maintenance and function of mesenchymal cells involved in epithelial instructive functions during organ development.
Outside the context of mesenchymal/epithelial interactions, PDGF-αR/PDGF-A has also been shown to participate in CNS development by regulation of oligodendrocytes and astrocytes.
Developmental Roles of PDGF-βR
Analyses of PDGF-βR and PDGF-B knockout mice have identified this ligand-receptor pair as key molecules in recruitment and function of vascular mural cells [5]. Deficiency of these genes is associated with reduced pericyte coverage, endothelial hyperplasia, and abnormally variable capillary diameter [17, 18]. Defects in the formation of kidney glomeruli, including failure in recruitment of mesangial cells and an accompanying defect in capillary branching, also illustrate the importance of PDGF-βR for proper regulatory functions of perivascular cells [19]. Both in the case of angiogenesis and glomeruli formation, endothelial cells are the main source of PDGF-BB whereas the receptor is expressed on the mural cells.
The importance of the PDGF-B/PDGF-βR axis for vascular function has also been supported by human genetics data. This occurred through identifications of loss-of-function mutations of PDGF-B and PDGF-βR in familial idiopathic ganglia calcification and the concomitant demonstration of vascular defects in the etiology of the disease (reviewed in [20]).
Physiological Roles of PDGFs
Roles for PDGFs in wound healing have been implied since the original purification of PDGF from platelets and the associated demonstration of potent mitogenic and chemotactic effects on fibroblasts. These notions have been substantiated by numerous model system studies and clinically validated in phase III studies which demonstrated increased wound closure in chronic diabetic neuropathic ulcers by recombinant PDGF-BB [21]. PDGF-βR signaling in dermal fibroblasts has also been shown to counteract edema formation. The underlying mechanism involves effects of PDGF-βR-regulated fibroblasts on interstitial fluid pressure which, in turn, involves integrin-mediated interactions with extracellular matrix [22].
Tumor Phenotypes Controlled by Stromal PDGF Receptors
As outlined above, studies in cell and developmental biology have identified PDGF receptors as important regulators of fibroblasts and pericytes. These findings have prompted a number of experimental studies exploring how PDGF signaling in these cells impact on different aspects of tumor biology. As detailed in the following paragraphs, these experimental studies indicate that PDGF receptor signaling in fibroblasts and pericytes can stimulate primary tumor growth and metastasis and also negatively regulate uptake and efficacy of systemically delivered drugs (Fig. 5.2).


Fig. 5.2
Schematic illustration of tumor biology processes regulated by PDGFR-positive fibroblasts (brown star-shaped cells) and perivascular cells (green elongated cells) (modified from Pietras and Ostman, Exp Cell Res, 2010)
PDGF Receptors in Fibroblasts
The stimulatory effects of stromal PDGF receptors on tumor growth were first demonstrated in experiments where melanoma cells, lacking PDGF receptors, showed increased tumor formation upon overexpression of PDGF-BB [23]. Although these initial studies did not provide detailed mechanistic information, it was concluded that the growth advantage was related to increased angiogenesis and recruitment of tumor-supportive fibroblasts.
Studies using similar experimental approaches, with overexpression of PDGF ligands in receptor-negative cells, demonstrated the stimulatory effects of different PDGF ligands in models of, e.g., skin, breast, and lung cancer types, where increased PDGF-dependent recruitment of fibroblasts was implied as the underlying mechanism [24–28]. Further support for this concept has also been obtained in studies where pharmacological inhibitors of PDGF receptor signaling displayed therapeutic effects in a genetic mouse model of skin cancer [29].
Pro-metastatic effects of PDGF-activated fibroblasts have been observed in different animal models. The PDGF inhibitor imatinib significantly reduced metastasis in an orthotopic model of colorectal cancer, with PDGF receptor expression restricted to the tumor stroma, which occurred in the absence of major effects on primary tumor growth [30]. Furthermore, tissue culture studies have demonstrated that PDGF-BB stimulation of fibroblasts enhances their ability to stimulate, in a paracrine manner, colorectal cancer cell migration and invasion [31]. PDGF-induced secretion of stanniocalcin 1 (STC1) was identified as a critical component of this paracrine pathway. Animal studies provided independent evidence for pro-metastatic effects of fibroblast-derived STC1.
Important roles for stromal PDGF receptors in determining drug efficacy have also been postulated by findings from mouse cancer model studies. These studies were prompted by initial observations in edema models, referred to above, which demonstrated an ability of PDGF-βR signaling in fibroblasts to increase interstitial fluid pressure (IFP) and thereby reduce edema (reviewed in [22]). These findings prompted studies which tested the hypothesis that increased tumor IFP, associated with reduced tumor drug uptake, could be overcome by targeting of stromal PDGF receptors. A series of studies, using different combinations of tumor models and PDGF inhibitors collectively provided strong evidence that blocking of stromal PDGF receptors indeed reduced tumor IFP, increased tumor drug uptake and enhanced the therapeutic efficacy of systemically delivered drugs [32–35]. Notably, the efficacy-enhancing effect of PDGF inhibitors was observed in studies using standard chemotherapy agents including 5-FU and Taxol, as well as macromolecules such as radiolabeled tumor-targeted antibodies.
PDGF Receptors on Perivascular Cells
The recognition of the importance of tumor angiogenesis, together with the developmental biology-derived evidence linking PDGF-βR to pericyte function, suggested that perivascular PDGF receptors could regulate tumor angiogenesis and thereby affect tumor growth and progression.
The first study exploring this concept used the PDGF-Bret/ret mice, expressing a truncated hypomorphic form of PDGF-BB, which earlier had been shown to display reduced pericyte coverage. Experiments with xenograft tumors in these mice established that attenuated PDGF-βR signaling reduced tumor angiogenesis [36]. Subsequent studies demonstrated that overexpression of PDGF-βR ligands in cancer cells enhanced growth of melanoma xenografts [37]. Analyses of the tumor stroma implied increased pericyte coverage, occurring in the absence of changes in vessel density, as the mechanism underlying the tumor-supportive effect. Later, studies in genetic mouse cancer models combined VEGF- and PDGF-βR-targeting agents and promoted a concept suggesting tumor vessel-stabilizing and pro-tumoral effects of PDGF-βR-dependent perivascular cells [38]. The general significance of these findings have been challenged in more recent studies where perivascular PDGF-βR instead was associated with reduced tumor growth, suggesting stage- and tumor-type-specific effects [39, 40].
The impact of perivascular PDGF-βR on metastasis has been experimentally explored in studies, which have used suicide-gene-mediated depletion of PDGF-βR-positive particular cells [41, 42]. Both studies indicated that loss of PDGF-βR-positive perivascular cells induced a pro-metastatic tumor phenotype, including increased hypoxia, c-MET-dependent tumor cell stimulation, and increased angiopoietin2-dependent angiogenesis.
Additionally, links have also been made between perivascular status and tumor immune surveillance. Analyses of the “low-pericyte tumors” in the PDGF-Bret/ret mice noted an increased tumor infiltration of immune-inhibitory MDSCs [43]. This occurred together with a reduced T-cell infiltration and an immune signature implying reduced antitumoral immune activity. An interesting implication of these studies, which should be further explored, is that perivascular status might determine response to immune therapy. Relationships between perivascular PDGF-βR status and response to treatment remain unclear. Efficacy of anti-VEGF agents has been reported to be either unaffected or increased in models where perivascular PDGF-βR status has been manipulated [38, 44].
PDGF Receptor Status and Prognosis
As outlined above, experimental studies have implicated PDGF receptors as important stimulatory molecules for fibroblasts and perivascular cells. In parallel, tumor microenvironment studies have demonstrated that stromal cells contribute to tumor progression and drug response. Together, these research areas have prompted a series of studies which have analyzed potential associations between stromal PDGFR status and survival in different tumor types. Differential expression of the two PDGF receptors has indeed been identified in tumor stroma of clinical samples (Fig. 5.3).


Fig. 5.3
Microphotograph illustrating variable expression of PDGF-αR and PDGF-βR in tumor stroma of human colorectal/pancreatic cancer samples (pictures courtesy of A. Mezheyeusky)
Most of these studies have used conventional immunohistochemistry with antibodies recognizing PDGF-αR or PDGF-βR, together with manual semiquantitative scoring of stromal expression. Other studies have also been performed in which these antibodies have been used together with digital-image-analysis-based scoring [45]. These novel methods, in addition to giving quantitative data, have also allowed differential analyses of PDGF receptor expression in perivascular areas and in fibroblast-dominated tumor regions.
Some studies have employed pPDGFR antibodies to monitor expression of activated receptors [46], although concerns have been raised regarding the specificity of these reagents. A proximity ligation assay for detection of phosphorylated PDGFRs has also been described [47–49], but not yet used to report on stromal PDGFR status. A shared problem for these two approaches is the sensitivity to artifacts caused by tissue handling affecting protein phosphorylation.
PDGF-βR status in clinical samples has also, in addition to these protein-based assays, been determined by bioinformatics-based approaches. In these analyses a “PDGF-βR signature,” derived from PDGF-BB-activated cultured fibroblasts, was used to analyze a number of different breast cancer gene expression datasets [50]. The following paragraphs discuss results from these analyses with subsections focusing on, firstly, fibroblast expression of PDGFRs and, secondly, perivascular PDGFR expression.
PDGFR Expression in Stromal Fibroblasts
The first major study on the prognostic relevance of stromal PDGF-βR expression was done in breast cancer and uncovered significant associations between high stromal expression and shorter recurrence-free and breast cancer-specific survival based on univariate analyses [51]. The study, based on approximately 290 cases, also identified strong correlations between stromal PDGF-βR and poor prognosis markers such as high grade, high proliferation, and HER2 amplification.
Strong and robust signals linking stromal PDGF-βR signaling to poor prognosis in breast cancer were also obtained in the gene signature study, in which a tissue-culture-derived PDGF-βR signature was used [50]. In this study, gene expression data from tumors of four different breast cancer cohorts was used to dichotomize cases into “high-signature-score” and “low-signature-score” groups. The “high-signature-score” group showed consistent associations with poor prognosis in the different cohorts in analyses using recurrence-free survival or disease-specific survival/overall survival as end points. Importantly, significant prognostic association was also detected in multivariate analyses using standard clinico-pathological characteristics (HR 1.2–1.3) or other stroma-related signatures (HR 1.3–1.6). Based on subset analyses, the prognostic impact was strongest in low-grade and Luminal A tumors. As in the IHC study, strong correlations were noted between high stromal PDGF-βR and poor prognosis markers such as high grade, high proliferation, and HER2 amplification.
The impact of stromal PDGF-βR has also been analyzed in prostate cancer. This study relied on analyses of individuals subjected to “watchful waiting” and thus represents the natural course of the disease [52]. The analysis of 266 tumors demonstrated significantly shorter cancer-specific survival in the group with high PDGF-βR expression (HR 2.4 in univariate analyses). Scoring was also done on histologically normal adjacent tissue. Interestingly, similar associations with survival were detected in this dataset. Whether these findings reflect that prognosis is affected by “constitutional” inter-individually variable PDGF-βR expression or rather reflect tumor “field-effects” remains unresolved.
Other conventional IHC studies have also demonstrated associations between high PDGF-βR and poor prognosis in gastric, colorectal, and pancreatic cancer [53–55]. Analyses of rhabdomyosarcoma have provided the first evidence that stromal PDGF-βR is also relevant for prognosis in sarcomas through findings of significant associations between stromal PDGF-βR and development of distant metastasis [56].
More recently a series of studies have been performed where stromal PDGF-βR status has been determined following IHC analyses and digital-image-analyses-supported automated scoring. These studies have provided novel data from ovarian and renal cell cancer showing that, also in these tumor types, high stromal/fibroblast PDGF-βR expression is associated with poor prognosis [45, 57]. In the case of ovarian cancer, the signal from univariate analyses was maintained in multivariate analyses.
As outlined above, high PDGF-βR expression appears to be consistently associated with poor prognosis. Concerning PDGF-αR less information is available [58]. Some tissue culture studies have shown that PDGF-αR is downregulated upon activation of fibroblasts by TGF-beta [59]. It is therefore possible that PDGF-αR expression marks a resting, and possibly growth restraining, fibroblast population. Further studies are therefore warranted to explore the possibility that the two PDGF receptors mark different fibroblast subsets which might show differential associations with outcome.
Perivascular PDGF-βR Expression
Developmental biology and experimental tumor biology studies have established that perivascular cells exert important regulatory functions affecting vascular biology which in turn impact on normal physiology and the pathophysiology of tumors. Based on the established role of PDGF-βR as a key regulator of pericytes (see above), studies have been initiated exploring potential inter- and intra-case heterogeneity of perivascular PDGF-βR status and associations with other vascular features, clinico-pathological characteristics, and survival.
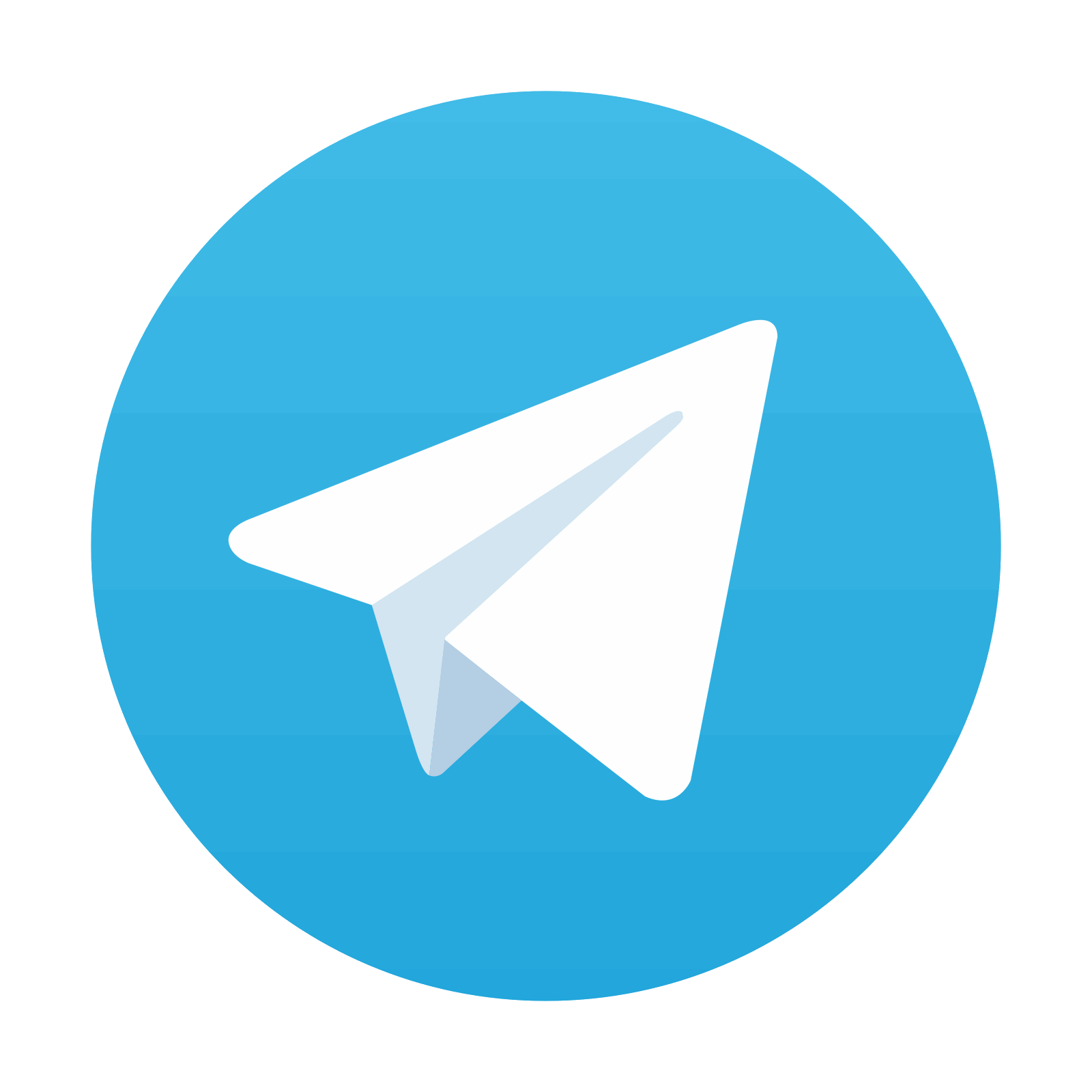
Stay updated, free articles. Join our Telegram channel

Full access? Get Clinical Tree
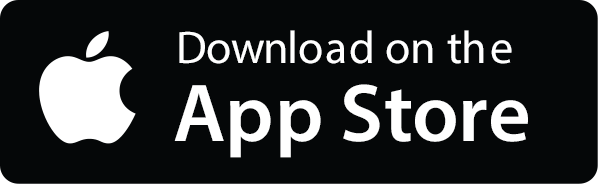
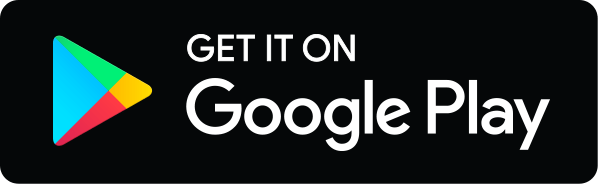