Gene
Protein
Other names
Common mutations
Associated features
GJB2
Connexin 26
DFNB1
35delG, M34T, 167delT, V37I, 235delC
Rarely skin conditions
SLC26A4 (PDS)
Pendrin
DFNB4
His723Arg, Thr416Pro, Leu236Pro
Mondini deformity, euthyroid goiter
MT-RNR1
12S rRNA
A1555G
Susceptibility to aminoglycosides
Standard evaluation for hearing loss includes a detailed medical history, physical examination, and audiometry. Imaging with MRI or CT scan may be useful for many individuals. With the discovery of dozens of genes for dominant and recessive hearing loss, genetic tests have become increasingly effective in identifying the specific cause of hereditary hearing loss. For all children with congenital hearing loss, genetic tests are now the first diagnostic modality recommended by otolaryngologists (Chan et al. 2011; Hart and Choo 2013; Shearer and Smith 2012).
18.3 Role of Free Radicals in the Most Prevalent Forms of Hereditary Hearing Loss
Free radicals play an important role in the pathogenesis of each of the three most common forms of hereditary hearing loss. Previously, the causal mechanisms behind hereditary hearing loss were largely unknown. The key to greater understanding of the pathogenesis of hereditary hearing loss has been the identification of the specific genes involved. The same genes can subsequently be examined in mouse models. Through these mouse models, the role for oxidative stress in hereditary hearing loss has been identified.
Still, free radicals should not be misconstrued as playing a role in the pathogenesis of all forms of hereditary hearing loss. For example, individuals with mutations in proteins forming the tectorial membrane typically have midfrequency hearing loss that is stable (McGuirt et al. 1999; Verhoeven et al. 1998). Mutations that decrease the exposure of the hair cells to sound may protect against noise-induced hearing loss (Legan et al. 2005).
18.3.1 GJB2 (Connexin 26)
Mutations in the gene encoding connexin 26, GJB2, are the most common cause of genetic hearing loss and account for over half of hereditary congenital deafness in many populations. The GJB2 mutation 35delG is the most common mutation in Europe, North America, South America, Northern Africa, and India (Mahdieh and Rabbani 2009). In Eastern Asia, the GJB2 mutation 235delC is the most common (Yan et al. 2003). 167delT is the most common mutation seen among the Ashkenazi Jews and in Palestinians (Sobe et al. 1999). Each of these mutations is common within the specified population and has a founder effect where a single mutation spread broadly throughout a population (Yan et al. 2003; Lerer et al. 2001; Ohtsuka et al. 2003; Van Laer et al. 2001). This lends support to a selection advantage for carriers of these mutations. Missense mutations in GJB2 are also very common, especially M34T, V37I, and L90P (Snoeckx et al. 2005). Hundreds of hearing loss mutations have been described in comprehensive databases and include both missense and nonsense mutations. Over 3 % of the hearing population in the United States is a carrier of a mutation in connexin 26 that causes deafness (Green et al. 1999).
The hearing loss associated with GJB2 mutations may vary from mild to profound (Snoeckx et al. 2005). The hearing loss is typically symmetric and worse in the higher frequencies (Green et al. 2003). GJB2 mutations are the most common etiology of profound congenital sensorineural hearing loss. However, many individuals have been identified who have passed newborn hearing screens, indicative of, at worst, mild hearing loss, who were subsequently found to be deaf (Green et al. 2000; Norris et al. 2006). The degree of hearing loss is worse for truncating and nonsense mutations than missense mutations (Snoeckx et al. 2005). In childhood and adulthood, the hearing loss is typically stable, but may be progressive (Kenna et al. 2010; Chan et al. 2010; Hochman et al. 2010). Neither improvement in hearing nor fluctuation has been noted with GJB2 mutations (Green et al. 2003).
The hearing loss associated with GJB2 mutations is typically nonsyndromic. However, several dominant mutations in GJB2 are associated with skin abnormalities (Lee and White 2009). The mutation causes no neurologic or cognitive dysfunction apart from direct consequences of its effect on the cochlea.
GJB2 mutations are known to interact with mutations in the gene GJB6, which encodes connexin 30 and is also found in the cochlea (Lerer et al. 2001). Additionally, there appears to be an interaction between mutations in MTRNR1 and GJB2 (Kokotas et al. 2010).
The gene GJB2 (gap junction beta 2) is found on the long arm of chromosome 13 (13q11-q12). This gene encodes connexin 26 (Cx26), one of several connexins found within the ear. Connexins aggregate in groups of six around a central 2.3 nm pore to form a connexon. Connexons from adjoining cells can covalently bond to form a channel. In large aggregates these channels form plaques and gap junctions. These channels allow the transmission of small ions and signaling molecules. They are presumed to be important in the potassium pathway recirculation where potassium entering the hair cells during mechanotransduction is shuttled back to the stria vascularis. A similar pathway can enable shuttling of free radicals.
Mutations in GJB2 reduce the ability of supporting cells to shuttle potassium, free radicals, and other metabolic by-products involved in sound transduction (Martínez et al. 2009). Cell death is seen in the hair cells and supporting cells in both human temporal bones and mouse models. The mechanism for this apoptotic cell death appears to be increased production of free radicals and potassium-induced cytochrome c release. In addition, there may be a direct dependence of free radical formation on potassium channel function during the respiratory chain in mitochondrial function. Local extracellular accumulation of potassium due to the absence of connexin 26 could result in both increased glutamate concentrations and oxidative stress. Trauma-induced decreases in connexin 26 levels in the spiral ligament can be prevented by pretreatment with the antioxidant tempol or the nitric oxide synthase inhibitor N(ω)-nitro-l-arginine methyl ester (l-NAME) (Nagashima et al. 2009). Gap junction channels are under negative regulation by reactive oxygen species (Martínez et al. 2009). Overexpression of connexin 26 is known to have a protective effect against oxidant-induced death in a nonotologic epithelial cell line (Hutnik et al. 2008). Antioxidants may protect gap junction function from oxidative damage induced by ototoxic insults (Fukuda et al. 2000).
18.3.2 SLC26A4 (Pendred Syndrome and Isolated Enlarged Vestibular Aqueduct)
Mutations in the gene encoding SLC26A4 are the second most common causes of nonsyndromic hearing loss and the most common cause of syndromal hearing loss in many populations (Albert et al. 2006). The carrier rate for SLC26A4 mutations in the hearing population is lower than GJB2, and there is no evidence of a founder effect or selective advantage for mutations in this gene. Several mutations including H723R, T416P, and L236P have been seen across geographically diverse locations (Campbell et al. 2001). Interestingly, many individuals with only a single identified mutation in SLC26A4 have hearing loss (Albert et al. 2006).
The hearing loss associated with SLC26A4 mutations is typical of that associated with enlargement of the vestibular aqueduct, viz., progressive and fluctuating. Hearing loss is predominantly sensorineural with a conductive hearing loss in the lowest frequencies. Hearing loss may be asymmetric. Hearing may precipitously decline after minor head trauma or progress slowly over time (Honings et al. 2008). Progression to profound hearing loss during adolescence is common for individuals with mutations in SLC26A4.
Mutations in SLC26A4 are associated with inner ear abnormalities that can be visualized with computed tomography or magnetic imaging of the temporal bones (Campbell et al. 2001; Everett et al. 1997). The Mondini deformity is the most common abnormality and consists of enlargement of the vestibular aqueducts with incomplete partition of the cochlea. However, isolated vestibular aqueduct enlargement and even unilateral vestibular aqueduct enlargement are often seen in individuals with SLC26A4 mutations. Enlargement of the vestibular aqueduct is alternatively called large vestibular aqueduct, enlarged vestibular aqueduct, or dilated vestibular aqueduct. Precise diagnostic criteria remain in flux, but a vestibular aqueduct with a width that is greater than 1.0–1.5 mm is a relatively broadly accepted criterion (Boston et al. 2007; Vijayasekaran et al. 2007). In individuals with unilateral vestibular aqueduct enlargement, hearing loss may occur bilaterally or unilaterally. Unilateral hearing loss may occur on the side contralateral to the enlarged vestibular aqueduct.
Most hearing loss associated with SLC26A4 is nonsyndromic. However, mutations may occur with euthyroid goiter formation that begins in the late teens (Everett et al. 1997; Pryor et al. 2005). Hearing loss when associated with goiter is known as Pendred syndrome. Pendred syndrome is the most common cause of syndromal hearing loss. The likelihood of having or developing thyroid disease may be correlated with the genotype (Pryor et al. 2005; Campbell et al. 2001). Thyroid abnormalities can be identified prior to the development of goiter through a perchlorate discharge test which identifies a partial organification problem.
Mutations in SLC26A4 are known to interact with mutations in the potassium channel gene KCNJ10 to cause deafness (Yang et al. 2009). Many individuals, largely with enlarged vestibular aqueducts, have been identified with hearing loss and only a single mutation in SLC26A4 (Campbell et al. 2001; Pryor et al. 2005).
The gene SLC26A4 (solute carrier family 26 anion exchanger, member 4) is found on the long arm of chromosome 7 (7q31). This gene encodes pendrin. Pendrin is a transmembrane anion transporter that transports chloride, iodide, and bicarbonate (Scott et al. 1999). Pendrin is present in the kidneys, inner ear, and thyroid. Within the inner ear, the role of pendrin is thought to be due to chloride and bicarbonate exchange. In the thyroid gland, the effect of iodine transport disruption leads to goiter formation.
Mutations in SLC26A4 result in disruption of the inner ear. Initially, the anatomic entity of enlarged vestibular aqueduct was presumed to be the cause of hearing loss. Evidence against this being the case includes hearing loss in the side contralateral to the enlarged vestibular aqueduct and the failure of surgical intervention directed at the vestibular aqueduct. When the gene was identified as an anionic transporter, a metabolic basis for hearing loss was reconsidered. The creation of animal models and precise dissection of the metabolic derangements associated with SLC26A4 mutations has identified oxidative stress as a strong role player in the development of deafness (Hochman et al. 2010). Loss of the bicarbonate secretion leads to acidification of the endolymph and subsequent free radical stress-mediated loss of Kcnj10 in the stria vascularis which abolishes the production of the endocochlear potential (Singh and Wangemann 2008). For a detailed discussion of the stria vascularis and endocochlear potential, see Chap. 3 by Ohlemiller.
18.3.3 MTRNR1 (the Mitochondrial Gene Encoding 12S rRNA)
Mutations in the mitochondrial gene MTRNR1 are the most common cause of mitochondrial deafness (Hutchin and Cortopassi 2000). The gene was first identified based upon hereditary predisposition to deafness after therapeutic doses of streptomycin were given for antituberculosis therapy (Prezant et al. 1993). A single mutation, A1555G, is a leading cause of deafness in many populations worldwide. This mutation has recurred multiple times across ethnic backgrounds (Torroni et al. 1999). This mutation is a leading cause of deafness around the world and is estimated to account for a large portion of all hearing loss associated with aminoglycosides. In some countries, this mutation is very common, particularly in familial hearing loss of late onset (Estivill et al. 1998).
The hearing loss associated with MTRNR1 mutations varies from congenital profound to mild and is often not seen until late adulthood (Estivill et al. 1998). However, with even small doses of aminoglycosides, profound hearing loss may result (Estivill et al. 1998). For a detailed discussion of the role of the A1555G mutation in aminoglycoside-induced hearing loss, see Chap. 10 by Rybak and Brennar.
The hearing loss associated with MTRNR1 mutations is typically nonsyndromic, but has associations with hypertension or cardiomyopathy (Santorelli et al. 1999; Chen et al. 2012). The T1095C mutation in MTRNR1 may result in isolated hearing loss or deafness, neuropathy, and parkinsonism (Thyagarajan et al. 2000; Zhao et al. 2004).
MTRNR1 mutations have been shown to interact with TFB1M (the mitochondrial transcription factor B1) which is a modifier for the severity of hearing loss in patients with A1555G (Bykhovskaya et al. 2004). The gene MTO1, involved in tRNA hypermodification, has been shown to play a role in the development of hearing loss in individuals with A1555G mutation who have not been exposed to aminoglycosides (Li et al. 2002).
The gene MTRNR1 is found in mitochondrial (648–1,601 bp) rather than nuclear DNA. Outside of the nucleus of a cell where the paternally and maternally inherited DNA from the 23 pairs of chromosome subsides, several copies of DNA are present within the mitochondria. Mitochondrial DNA found within eukaryotes is different in many ways than nuclear DNA and is postulated to have an ancient bacterial origin (Fischel‐Ghodsian 1999). This DNA is inherited usually exclusively from one’s mother from the mitochondria of the egg. The copies of mitochondrial DNA within a cell may be entirely the same (a condition called homoplasmy) or may differ (a condition called heteroplasmy). A1555G is usually found in homoplasmy.
The gene MTRNR1 encodes the 12S rRNA. 12S rRNA is a component of the small mitochondrial ribosome subunit (28S).
The folding of this gene, specifically the stem-loop structure, is altered in the A1555G mutation such that there is a great similarity between the human 12S rRNA and the corresponding ribosomal RNA (16S rRNA) found in bacteria that are sensitive to aminoglycosides (Perletti et al. 2008; Prezant et al. 1993). This altered folding pattern allows aminoglycosides to bind to the human-altered mitochondrial ribosome in a way similar to the way that it binds to the bacterial ribosome. The binding of the aminoglycoside to the human ribosomal RNA results in destruction of the mitochondria.
Mitochondrial defects preferentially adversely affect the inner ear in comparison to other tissues. The effectiveness of free radical scavengers, both endogenous (like glutathione levels) and exogenous, in preventing aminoglycoside hearing loss further points to the role of free radicals in the development of this type of hearing loss. At the cellular level, aminoglycosides cause damage through excessive formation of reactive oxidative species (Talaska et al. 2006). These in turn trigger cell death pathways through caspases or cathepsins.
18.4 Interventions for Hereditary Hearing Loss
For individuals with free radical-associated hearing loss that is associated with genetic mutations, there are two specific medical goals: to optimize performance and to maintain or improve hearing. Ideally, we will do this with as little risk and as few side effects as possible. Through understanding of the specific causes of deafness, diagnostic and prognostic information is gained. This can specifically lead to individualized therapeutic programs (Table 18.2).
Table 18.2
Treatment of hereditary hearing loss
Behavioral |
Avoidance of specific insults in genetically susceptible populations |
Devices |
Amplification |
Middle ear surgery |
Cochlear implantation |
Medications |
Steroids |
Antioxidants |
Genetic interventions |
Nonspecific (i.e., increased endogenous antioxidant production) |
Specific correction of the underlying defect |
18.4.1 Behavioral Interventions
It is well known that some individuals are particularly sensitive to otologic insults of many types. The genetic basis for some of this susceptibility is beginning to be identified. For example, greater susceptibility to noise-induced hearing loss requires the analysis of large numbers of individuals (Konings et al. 2009; Sliwinska-Kowalska and Pawelczyk 2013). If the susceptible individuals can be identified, a conscientious decision can be made to avoid exposure.
The clearest implementation of this has been the avoidance of aminoglycosides in individuals that carry the MTRNR1 mutation A1555G. Screening for this mutation in individuals prior to the administration of aminoglycosides could lead to the prevention of deafness in many individuals (Rahman et al. 2012; Bitner-Glindzicz et al. 2009). A genetic screening program is being initiated at several centers around the world.
For individuals with SLC26A4 mutations, head trauma can precipitously result in hearing loss (Gopen et al. 2011; Honings et al. 2008). Avoidance of sports and other activities that can result in head trauma is recommended.
Diet modification and supplementation is an intriguing potential intervention for individuals with susceptibility to free radical-induced hearing loss. Diets rich in antioxidants have been associated with improved hearing, as discussed in detail in Chap. 6 by Spankovich. Micronutrient supplementation with fluoride has markedly decreased hearing loss associated with otosclerosis, an often hereditary form of hearing loss of uncertain etiology (Chen et al. 2002; Vartiainen and Vartiainen 1997). Supplementation with biotin has improved another (Straussberg et al. 2000). Therapeutic micronutrients can potentially be identified based upon the genomic profile of an individual, e.g. individuals with mutations in genes associated with otosclerosis may benefit from fluoride supplementation.
18.4.2 Devices
Hearing aids may be useful for many individuals. Generally, hearing aids are much better than cochlear implants for individuals with less than severe hearing loss. Hearing aids are broadly used by individuals with mutations in GJB2, SLC26A4, and MTRNR1, as well as many other types of genetic hearing loss. For some types of genetic hearing loss (such as those associated with otosclerosis), stapedectomy or other middle ear surgical implants may be of benefit (Chen et al. 2002).
When hearing aids are inadequate, cochlear implantations may be useful. The results of cochlear implantation may vary based upon the genetic etiology of deafness. Specifically, children with GJB2 mutations obtain exceptional results after cochlear implantation (Green et al. 2002). Adults with SLC26A4 mutations have excellent results after cochlear implantation (Hochman et al. 2010). Individuals with MTRNR1 mutations do well after cochlear implantation (Sinnathuray et al. 2003). Not all individuals with genetic mutations will perform well after cochlear implantation: CHD7 mutations are a known predictor of poor performance after cochlear implantation (Lanson et al. 2007).
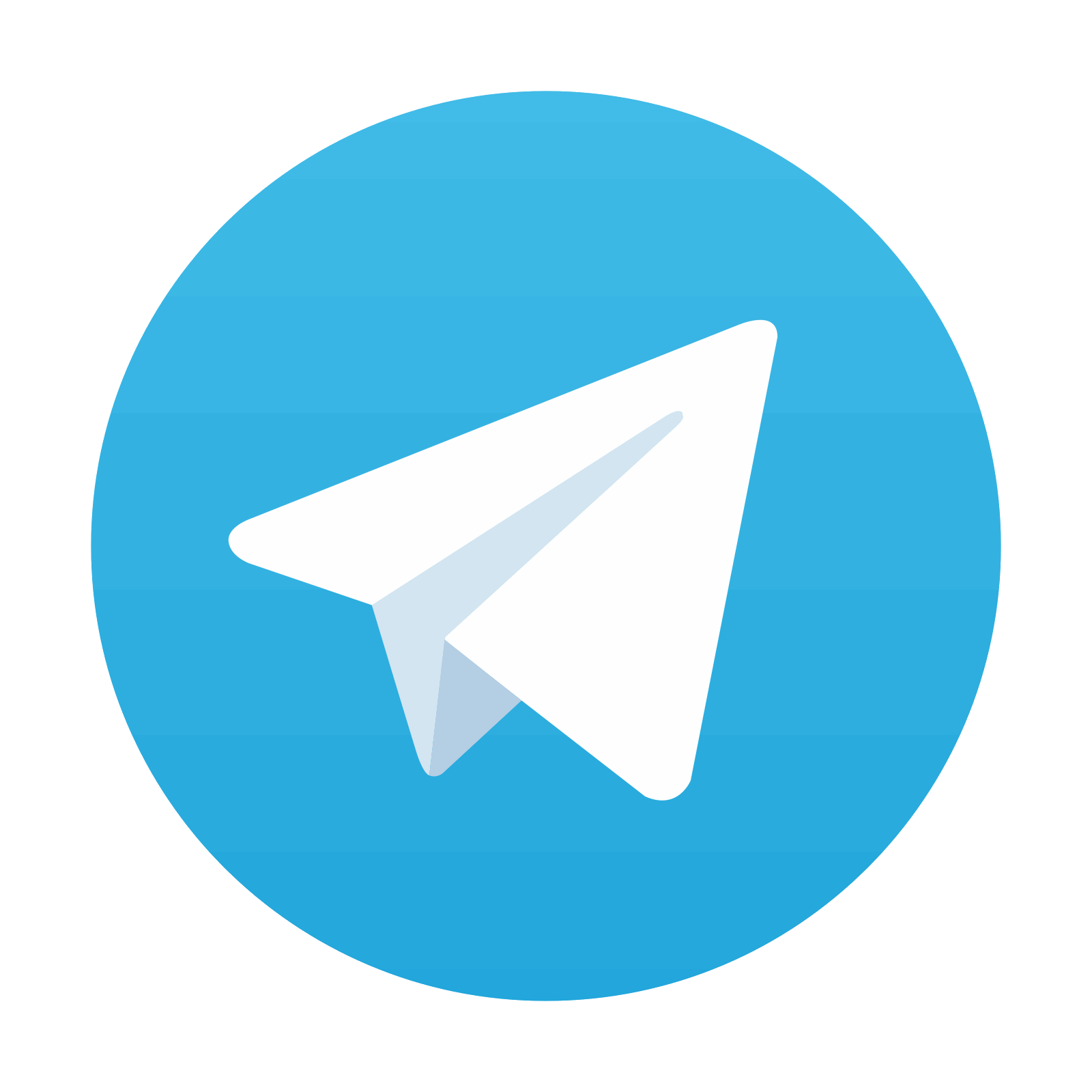
Stay updated, free articles. Join our Telegram channel

Full access? Get Clinical Tree
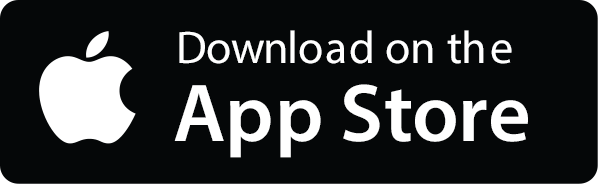
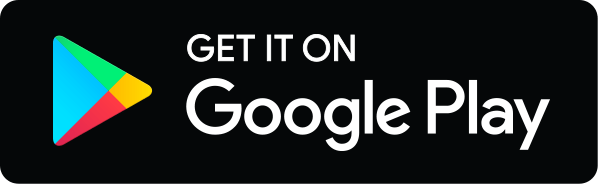