Sterile Packaging
Thierry Wagner
Dan B. Floyd
Michael H. Scholla
Jane E. Severin
In 1668, Francesco Redi, an Italian physician and biologist, published a book entitled Experiments on the Generation of Insects challenging the theory of “spontaneous generation” of maggots from dead flesh or, in a more general way, of putrefying matter.1 Under the theory of spontaneous generation, the widely held belief at the time, microorganisms were formed without reproduction from parents. Redi prepared jars with raw meat, some he left open and others he covered with corks or with “very fine Naples veil” to avoid flies entering the jars to deposit their larvae. Maggots only appeared in uncovered jars. Redi’s experiments were a cornerstone of the scientific work that allowed Louis Pasteur, Joseph Lister, Robert Koch, and others to understand the true nature of microorganisms. But what was also remarkable, Redi conducted probably the first scientific experiments with containers covered with a porous lid and he worked with a control, in this case with uncovered jars, to prove his hypothesis, an innovative concept at the time. The veil used was a barrier to flies, and allowed air to enter, important to counter the arguments of those believing that air was required for life to develop. The veil was probably not a good microbial barrier, but by challenging the theory of spontaneous generation, the concept of protection for preservation became possible. It took however two additional centuries to finally root out this ancient theory.
In 1745-1748, John Needham, a Scottish clergyman and naturalist, showed that broth still shows microbial growth when conserved in a hermetically sealed container after being boiled, although the boiling should have destroyed all forms of life. Critics pointed out that he may have recontaminated the broth during transfer to the container.1 Another Italian, Lazzaro Spallanzani at the University of Reggio Emilia, repeated these experiments during 1765-1767 in sealed glass flasks using a special procedure to avoid recontamination. Spallanzani concluded that the elements at the source of putrefaction could be killed by sufficiently long boiling. He also proposed that these elements can move through air and the hermetic sealing protects from recontamination. He even showed that the liquid could not be preserved in flasks with an integrity issue. His opponents claimed that his hermetically sealed flasks did not allow air to enter, which was believed at the time to be an essential requirement (ER) for life.1 But it was Louis Pasteur who finally gave the mortal blow to the theory of spontaneous generation with his famous swan neck flask experiments during the period of 1859-1865 (see Figure 2.2, chapter 2). His flasks had a thin and long tub neck shaped like the neck of a swan. Air could enter, but particulates would not get into the flask as they settled somewhere in the S-shaped neck of the flask. Pasteur filled the flasks with a meat broth, sterilized them through boiling, and could demonstrate preservation of these liquids for weeks or months unless the bulb was tilted such that some liquid could reach the bottom of the S-shaped neck where particulates with microorganisms had settled. Microorganisms would also develop when the neck was broken off such that the surrounding air could enter freely into the flask. With his experiments, Pasteur demonstrated that a torturous path could be an effective barrier to microorganisms, a concept still in use today. He also showed the limitations of the swan neck tortuous path. When the flasks were violently shaken such that air would rush in, then it was possible of observe microbial growth. He even demonstrated that the air of Paris was more contaminated than mountain air at 850 m or even 2000 m above sea level (see Vallery-Radot1).
In the 19th century, Louis Pasteur, Robert Koch, Joseph Lister, and many others set the basis for moving into modern medicine with the increasing knowledge of microbiology. Sterilization at the point of use with carbolic acid and heat was the first approach without any preservation means. As packaging emerged to preserve the microbiological state, it was mainly based on metal or glass containers. During the 19th century, a lot of research focused on the preservation of foods.
François Nicolas Appert2 invented the process of food bottling and was awarded by Napoleon in 1810 who was keen to have a method to preserve the food for his armies. The effective and simple method was widely adopted in a short time span. Preservation was first done in glass bottles and later in tin cans invented by Peter Durand. The process of bottling was further improved by Dr Rudolf Rempel who filed a patent in 1892 to close and vent sterilization containers. His patent was the basis for Johann Carl Weck to create the company Weck GmbH, which still marketing their Weck preservation bottles today.3
François Nicolas Appert2 invented the process of food bottling and was awarded by Napoleon in 1810 who was keen to have a method to preserve the food for his armies. The effective and simple method was widely adopted in a short time span. Preservation was first done in glass bottles and later in tin cans invented by Peter Durand. The process of bottling was further improved by Dr Rudolf Rempel who filed a patent in 1892 to close and vent sterilization containers. His patent was the basis for Johann Carl Weck to create the company Weck GmbH, which still marketing their Weck preservation bottles today.3
The limitations of boiling for sterilization became quickly apparent. It took until 1879 for the first autoclave for medical purposes to be introduced by Charles Chamberland in Paris, although Denis Papin had already developed a precursor in 1679. Using this equipment, Pasteur demonstrated that steam sterilization was particularly effective to kill microorganisms. Steam sterilization presented a problem that any packaging had to be porous to allow the steam to reach the packaged devices. In 1890, Curt Schimmelbusch4 filed a patent for sterilization containers, the so-called Schimmelbusch drum (Figure 41.1), and he recommended the steam sterilization of wound dressings in his famous book published in 1892 with the support of Prof Dr E. von Bergmann. Schimmelbusch4 described the preservation of sterilized wound care products in sterilization drums as well as preservation of surgical catgut ligatures in special containers. The same year, Aesculap (in Germany) created the first rigid sterilization containers responding to the needs of military hospitals. Originally, they were equipped with valves or sliding vents replaced in the 1930s with reusable filters.
As medicine continued to make progress internationally, Fred Kilmer, pharmacist in New Brunswick, New Jersey, saw the potential of offering ready-made packaged sterile dressings, which he described in 1897 in an article entitled “Modern Surgical Dressings.”5 The sterile glass jars were filled aseptically under well-controlled conditions and become the key product for the success of Johnson & Johnson. At the beginning of the 20th century, sterile packaging was still very basic, for example, Charles E. Parker6 specified packaging in his catgut ligature and suture patent of June 3, 1902, as follows: “wrapping or container of paper or similar material which is permeable to sterilizing fluids, but will exclude dust or any solid carrier of infection.” Parker’s description included all the essentials, but engineered microbial barrier materials were not yet available. He included a drawing, which showed an object wrapped in plain paper. The development of the prefilled syringe, combining primary packaging and drug delivery mechanism is an equally fascinating story. Johann Sigismund Elsholtz was one of the first to investigate ways of intravenous (IV) injection in 1667. Literature7,8 lists several inventors for the syringe working independently in the middle of the 19th century, namely, Alexander Wood (1817-1884), a Scottish physician, and Charles Gabriel Pravaz (1791-1853), a French surgeon. Robert Koch came up with a sterilizable syringe in 1888 and Becton, Dickinson and Company introduced the disposable syringe.7 In 1939, Erhard9 of E.R. Squibb and Sons, filed a patent for a “hypodermic unit,” which led to the development of the famous “morphine syrette” adopted by the US army during World War II. The design was simple and battlefield compatible; it consisted of a toothpaste-like squeezable metal container with a seal that was pierced by a wire in the attached hypodermic needle before the medication could be administered into the patient. In first aid kits, the syrette was protected with a hard fireboard tube. This innovative device combined the delivery system, packaging, and protection for extreme situations with simplicity and great usability to allow for administration in adverse and difficult conditions.
In the 1940s, the US military commissioned Drs Charles A Phillips and Saul Kaye to develop an efficient biological decontamination process.10 Their work at Fort Detrick, Maryland, on ethylene oxide (EO) established the scientific foundations for the introduction of EO as a gaseous sterilant. The EO sterilization, also in addition to the use of radiation, enabled the introduction of sterile medical devices made of materials that were not compatible with the high temperatures of steam sterilization. More and more single-use disposable medical devices emerged, and with that the need for appropriate packaging. Fabrics and medical paper were the most used material initially, joined by film packaging and later by DuPontTM Tyvek® in 1972.
Sterile packaging over the many years of its history has developed into an extremely diverse sector covering medical devices, combination products, and pharmaceuticals, addressing professional health care users as well as patients in a variety of situations such as home health, health care facilities, and military applications. The complexity of sterile packaging has grown with the emergence of sophisticated devices, sensitive drug products, new sterilization modalities, and aseptic processing technologies as well as with the development of sophisticated clinical procedures. Packaging continues to evolve with the
introduction of new drug delivery and device technology, to enable productivity improvements, the adoption of new materials and manufacturing technologies, the inclusion of active elements to control atmosphere, and the integration of microchips for smart functions, and to address changing regulatory requirements. But the fundamental aspects, discovered by the pioneers, are still true today.
introduction of new drug delivery and device technology, to enable productivity improvements, the adoption of new materials and manufacturing technologies, the inclusion of active elements to control atmosphere, and the integration of microchips for smart functions, and to address changing regulatory requirements. But the fundamental aspects, discovered by the pioneers, are still true today.

There are a broad range of sterile packaging types to package devices, combination products, or drugs. Before reviewing these in detail, it is worthwhile considering the intended use, typical functions, and key requirements for sterile packaging.
Sterilization Compatibility
The preferred and the lowest risk method to provide a sterile health care product is to package first and then sterilize the contents in the package (referred to as terminal sterilization). For drugs or devices that cannot be sterilized, aseptic processing is another option (see chapter 58). But even in this case, the various components including the packaging should be sterilized before filling the drug or device packaging operation. Packaging needs to allow for and must be compatible with the selected sterilization process(es). As an example, gaseous sterilization modalities require porous packaging for penetration of the gaseous sterilizing agent and evacuation of any residues. Packaging materials need to be able to withstand the specific sterilization process being applied in a way that the properties of the material stay within required limits and there are no chemical or other reactions that could lead to detrimental impacts on the product (safety and efficacy) or limited stability and performance of the packaging over the shelf life.
Package Integrity, Microbial, and Other Barrier Functionalities
The ability to exclude microbial contamination from the packaged product is a key feature of sterile packaging for the maintenance of sterility after sterilization or aseptic processing until the point of use. For preservation of sterile contents, packaging needs to maintain integrity over the shelf life and through the challenges of transportation and handling. In many cases, packaging must also shield the product from environmental impacts such as from oxygen or humidity while maintaining a set atmosphere inside. Porous materials for gaseous sterilization modalities should allow air to enter and exit while retaining particulates and airborne microorganisms. Air will pass typically through the porous structure of the sheet in a meandering pattern around fibers or filaments, representing a tortuous path with given microbial barrier properties, a concept originally demonstrated as effective by Pasteur. Porous materials are also used to allow packaging to adapt to changes in atmospheric pressure, important for large packages, or if transportation includes airfreight and significant changes in altitude.
The packaging, that is in direct contact with the product, is identified as primary packaging in pharmaceutical applications, whereas the medical device industry has introduced the terminology of sterile barrier system to identify the minimum package that minimizes the risk of ingress of microorganisms and allows aseptic presentation of the sterile contents at the point of use.11 The sterile barrier system is considered an essential accessory to a sterile device.
Protection of the Product and Its Sterile Barrier System
Packaging must protect the health care product from physical hazards, like shocks, vibration, compression of transport, distribution, and storage as well as from any harmful environmental elements like ultraviolet (UV) light, electromagnetic fields, temperature, or chemical products. To cope with these adverse conditions, packaging systems are often composed of various protective packaging layers, or secondary and tertiary packaging, including means for transportation like pallets or containers. If packaging alone cannot guarantee the protection, special conditions must be maintained like a temperature-controlled supply chain (cool or cold chains).
Biocompatibility Aspects and Interactions With the Health Care Product
Packaging can be in direct contact with a health care product, or during use, it may even come into contact with the patient. Biocompatibility, toxicity aspects, chemical compatibilities, potential leachables, and the risks of adverse impacts on the health care product from such interactions need to be understood. Leachables are chemical entities from materials in direct or even in indirect contact with the product that migrate to the product and as such contaminate it. Leachables could come from plastics and their antioxidants; from residual catalyst respective impurities; or from glass, metals, or any packaging materials. Further sources include printing inks used for labeling, label adhesives, paperboard, and cardboard boxes used for protection and from products used to treat pallets (eg, preservatives; see chapter 69). Leachables can lead
to effectiveness alterations of a drug product, inactivate active ingredients, or react with ingredients to form new chemical compounds that have further undesirable secondary effects. Packaging constructs could also bind components of a drug product to change it. Packaging is considered compatible with a health care product if its safety and intended use is not compromised and in case of a drug product if its efficacy is within required limits.12,13,14,15
to effectiveness alterations of a drug product, inactivate active ingredients, or react with ingredients to form new chemical compounds that have further undesirable secondary effects. Packaging constructs could also bind components of a drug product to change it. Packaging is considered compatible with a health care product if its safety and intended use is not compromised and in case of a drug product if its efficacy is within required limits.12,13,14,15
Packaging Usability
At the point of product use, packaging will be opened, removed, or the product will be dispensed, administered, or used. There are several functions and requirements for product usability that can impact packaging:
Identification of the product
Reading the information on the label, cautions, use-bydate information, required storage conditions, etc
Opening the package
Aseptically removing the sterile health care product or administering the drug
Aseptically handling components in an aseptic filling process
All aspects in the list earlier may have an impact on patient safety. Labels help with identification not only of the product but also design features like transparent web materials or windows in cardboard box allow for visual identification of the product. Label readability is important to support health care personnel that must deal with an increasing complexity under often stressful conditions. The use of symbols is preferred to increase label readability and to eliminate the need for multiple languages of internationally distributed products.
The packaging design must allow for appropriate aseptic technique to remove the sterile device to minimize the risk of cross-contamination and infection. Aseptic presentation after opening is ideally performed by two people, one to open the packaging and the other to remove the product from the package without contact with unsterile areas (Figure 41.2).
Aseptic presentation is defined as “transfer of the sterile contents from their sterile barrier system using conditions and procedures that minimize the risk of microbial contamination.”13 Often, health care acts are only performed by a single health care practitioner and packaging designs need to account for these specific situations. Regulations and standards put increasing focus on usability evaluation of the device, including its packaging, to minimize the risk of adverse events with the patient and to produce evidence that the design allows for easy handling and is safe.
Packaging Sustainability
Finally, empty packaging must be discarded properly, avoiding harm to the environment and to users, like unintended sticks from needles, and increasingly prepared for recycling and fed into the appropriate channels. Many jurisdictions have set ambitious goals to improve recycling rates moving to a more circular economy and packaging designs will have to adapt to optimize reuse and recycling possibilities.14

Sterile Packaging Types
There are numerous materials, styles, and formats used for medical packaging. To meet the needs of more and more complex products especially for combination products, there are new combinations of package styles and materials emerging every year. Combination products in these cases refer to a combination of a drug and device; a biological product and a device; a drug and biological product; or a drug, device, and biological product. These can pose a new challenge in the design of packaging and impact on sterilization requirements. Table 41.1 illustrates typical package formats for some common product types and characteristics.
Common Materials—Medical Device Packaging
Medical device packaging must primarily allow its contents to be sterilized and it must then maintain sterility until the time of use. There are exceptions to this, for example, a manufacturer may market a nonsterile device, such as many types of adhesive bandages. Packaging types include bags, overwraps, pouches, trays, fin-seal wrappers, form-fill-seal containers, and clamshells. The packages are made of a variety of materials; porous, nonporous, rigid,
flexible, high barrier, etc. Figure 41.3 shows the typical packaging used for sterile contact lenses.
flexible, high barrier, etc. Figure 41.3 shows the typical packaging used for sterile contact lenses.
TABLE 41.1 Types of sterile medical packaging formats | |||||||||||||||||||||||||||||||||||||||||||||||||||||||||||||||||||||||||||||||||||||||||||
---|---|---|---|---|---|---|---|---|---|---|---|---|---|---|---|---|---|---|---|---|---|---|---|---|---|---|---|---|---|---|---|---|---|---|---|---|---|---|---|---|---|---|---|---|---|---|---|---|---|---|---|---|---|---|---|---|---|---|---|---|---|---|---|---|---|---|---|---|---|---|---|---|---|---|---|---|---|---|---|---|---|---|---|---|---|---|---|---|---|---|---|
|
Medical Grade Paper
Medical grade paper is used primarily for two-dimensional flexible pouches or lidstocks sealed to flexible, rigid, or semirigid trays. It is selected typically because it is porous, which is necessary for sterilization by EO or steam. Porosity levels of paper structures vary widely. They are made of fibers that can generate particles when the package is opened, which can compromise sterility or lead to other complications following surgical use. Fiber generation, as an example, can be minimized by reinforcing the paper with a polymer. The reinforcement is intended to improve strength and to provide a clean peel, which is critical for medical device packages. Such paper treatments can influence material porosity levels compared to the original substrate, but the levels remain acceptable for use for a medical device package. Paper does require, in most cases, the addition of heat seal coating to one side of the material for sealing to a film web or tray.15
DuPontTM Tyvek®
A DuPont brand, DuPontTM Tyvek® is marketed as an alternative to medical grade paper. It is made of virgin high-density polyethylene (HDPE). The unique manufacturing process results in long, spun filaments, randomly laid and bonded into sheet form by heat and pressure. DuPontTM Tyvek® is unique because it provides a superior microbial barrier due to a tortuous path (Figure 41.4) created in its manufacturing process and it is also very breathable. A more breathable or porous material can reduce cycle times for sterilization, which can result in improved operational efficiencies. Unlike paper, the clean peeling characteristic is not dependent on additional treatments.
DuPontTM Tyvek® can be sealed to flexible webs and trays with or without the addition of a heat seal coating. DuPontTM Tyvek® also has superior physical strength and more resistance to tearing and puncturing.16
DuPontTM Tyvek® can be sealed to flexible webs and trays with or without the addition of a heat seal coating. DuPontTM Tyvek® also has superior physical strength and more resistance to tearing and puncturing.16
Aluminum
Aluminum can be in a foil form or vacuum deposited on films known as metallization. Aluminum is an excellent barrier against light, oxygen, and moisture. These properties are typically critical for the packaging of many types of combination products. When aluminum is the barrier layer in a lamination, it requires a heat-seal layer for sealing because aluminum does not seal. Also, aluminum is typically sandwiched between protective layers to eliminate any possible chemical reactivity and to minimize flex cracking. It is important to consider material thickness because aluminum is susceptible to the formation of pinholes. Pinholes are inherent due to the manufacturing process and are more prevalent in thinner materials.15
Plastic
Commonly used plastics for medical device packaging include polyethylene (low-density polyethylene [LDPE], HDPE), polystyrene (PS), high-impact PS, polypropylene (PP), polyvinyl chloride, polyvinylidene chloride (PVDC), polyethylene terephthalate glycol-modified (PETG), and polyester in addition to blends of materials. Plastics are used in flexible, semirigid, and rigid structures, as monolayers, laminations, or coextrusions of more than one material.
Plastics offer numerous characteristics and properties, such as a broad range of opacity, barrier properties, forming properties, sealability, strength, elongation, etc. It is important to thoroughly understand product requirements prior to selecting a plastic material for medical device packaging. Plastic films are compatible with sterilization methods that don’t require porosity (eg, electron beam and irradiation). Sterilization methods that require the ingress and egress of a gas, such as EO, do require material porosity. DuPontTM Tyvek® or medical grade paper are typically used in these cases. The porous material can be one or both sides of a flexible pouch, a lid for a semirigid or rigid tray, or a smaller area can be included as a strip or patch on a film pouch or lid. The adequacy of the reduced porous area for sterilization efficacy must be proven. See Figure 41.5, a nonporous foil pouch with a strip of porous material to allow for gas sterilization.17
Flexible Packaging Laminating
Laminating is the process in which two or more flexible packaging webs are joined together using a bonding agent. These webs are composed of films, papers, or
aluminum foils.15 To bond the webs, an adhesive is applied to the less absorbent substrate web, which is then pressed against the second web. This results in a two-layer laminate. Depending on required properties, a laminated structure can consist of several layers. Flexible packaging laminates provide mechanical product protection with excellent strength and tear resistance. They can provide excellent barrier properties to external elements such as light, moisture, and gases while also protecting the loss of product qualities such as freshness, aroma, etc. The laminate structure also provides a sealable layer to produce the finished package.17
aluminum foils.15 To bond the webs, an adhesive is applied to the less absorbent substrate web, which is then pressed against the second web. This results in a two-layer laminate. Depending on required properties, a laminated structure can consist of several layers. Flexible packaging laminates provide mechanical product protection with excellent strength and tear resistance. They can provide excellent barrier properties to external elements such as light, moisture, and gases while also protecting the loss of product qualities such as freshness, aroma, etc. The laminate structure also provides a sealable layer to produce the finished package.17
Coextruded Material
Coextrusion is the process of pressing two or more materials through the same extrusion die to produce a single material structure. When multiple plastics are combined, the result can yield properties different from those of a single material. Very thin layers of resin that cannot be made into a film alone can be layered through coextrusion. Several characteristics can be combined in a single film such as heat resistance, heat sealing capabilities, rigidity, flexibility, cold resistance, and easy-peel capabilities.17
Adhesives and Coatings
A medical device package must have a continuous, uninterrupted seal of adequate strength to maintain the integrity of the package. Most medical device packaging heat seals are designed to be peelable. A permanent seal, not intended to be opened for product use, is applied less frequently, for example, the bottom (base) seal of a flexible pouch. The challenge for heat seal designs is that the seal strength must be acceptable for the user to easily open yet strong enough to maintain package integrity through distribution. The user concerns include both the strength of the seal as well as the seal, when opened, providing a clean peel. A clean peel means that upon opening the seal does not generate particulates or fiber fragments that might compromise patient safety.
The processing variables critical for heat sealing are pressure (the force that a tool applies to the materials to make a seal), temperature of both the top and bottom tools, and dwell time or machine speed, which is the amount of time the tool is in contact with the materials to be sealed. It is important that these inputs be balanced to provide the optimum seal and manufacturing efficiency.17,18
Inks
Packaging labeling, graphics design, and print are not typically used as a marketing tool as in the consumer industries. There are regulatory requirements concerning ink migration, direct contact, solvents used, etc, that must be met to use an ink for a medical device package. The label content and package printing are primarily for product identification, storage instructions, instructions for use, expiration dating, and lot numbering. Another feature ink can provide is the ability to visually detect if an event occurred with color changing inks. Two common examples are inks that change colors after sterilization providing a visual indicator the package has been sterilized, or temperature extreme indicator inks that indicate any unusual conditions (eg, climate extremes) the product may have been exposed to during distribution.15,18
Common Materials—Pharmaceutical Packaging
Glass
The glass used in packaging pharmaceuticals falls into four categories, depending on the chemical constitution of the glass and its ability to resist deterioration. Type I, II, and III glasses are intended for parenteral products, and type IV or NP (non parenteral) is intended for other products. Each type is tested for its resistance to water attack. The degree of attack is determined by the amount of alkali released from the glass under specified test conditions. Obviously, leaching of alkali from the glass into a pharmaceutical solution or preparation could lead to negative effects such as affecting the pH and subsequently the stability of the product.
There are four types of glass:
Type I (neutral or borosilicate glass)
Type II (treated soda lime glass)
Type III (soda lime glass)
Type IV or NP (general purpose soda lime glass)
Type I glass is the least reactive using higher ingredients and processing cost. It is used for more sensitive pharmaceutical products such as parenteral or blood products. Most ampules and vials are made of type I glass. Type II glass has high chemical resistance but less than type I; therefore, ingredient and processing costs are also less. Many products can be packaged in type II glass; some exceptions include blood products and liquid pharmaceuticals with a pH less than 7.
Examples of the types of products packaged with glass are
Type I—ampules or vials containing liquid injectables of any pH, blood and related products, and aerosol product
Type II—injectables of pH <7
Type III—nonliquid injectables
Type IV or NP—dry powders for parenteral use (to be reconstituted before use); bottles and jars for tablets, capsules, oral solids, and other solids for reconstitution; oral liquids (solutions, suspensions, emulsions); nasal and eardrops; and droppers
Metals
Metal containers are used for nonparenteral pharmaceutical products. Metals can be strong, opaque, typically not impacted by temperature extremes, shatterproof, and impermeable to moisture, gases, odors, light, or bacteria. It is the ideal packaging material for pressurized containers (Figure 41.6). Examples include tubes, blisters, cans, aerosol, and gas cylinders. Aluminum is a common choice for both primary and secondary packaging for pharmaceutical products. It is relatively light, yet very strong. It provides a barrier to light and chemicals and is usually impermeable. Thin aluminum is used when a flexible foil is a component of a laminated packaging material such as collapsible tubes for semisolid preparations.15,19
Elastomers (Rubber)
Container closures (or seals) are a critical component for container closure systems. The closure, which is typically crimped in place, on the container with a metal ring, and the vial form the primary package for many types of pharmaceutical products, both wet and dry (Figure 41.7). The closures are commonly referred to as “stoppers” or “bungs” and are typically made from elastomeric materials or also referred to as rubber. An elastomeric material is a material that resumes its original shape when a deforming force is removed, also referred to viscoelasticity. For example, following the withdrawal with a sterile needle, from a vial, of a dose of the product for administration, an elastomeric closure can reclose after the needle is removed. This characteristic prevents contamination of the contents from the outside and from the closure itself. Less elastomeric closure materials can result in “coring.” Coring is when a piece of the stopper is pushed or breaks free due to the needle insertion and can result in the contamination of the vial contents. Low permeability to moisture, vapors, and gases; chemical resistance; and resistance to aging conditions are other important characteristics of a closure. Rubber, an elastomeric material, is an excellent material used to form closures such as stoppers or bungs for vials or gaskets in aerosol cans. Natural rubbers are common for multiple-use closures for injectable products. They do not stand up well to multiple autoclave cycles. The most critical aspect of using components made of natural rubber, including closures, is the possible release of allergenic latex proteins into the container contents or to the user from handling the product. It has compelled manufacturers to seek for less problematic materials that offer the same positive characteristics of natural rubber. Isoprene rubber products provide the flexibility and strength of natural rubber without any of its disadvantages, such as odor, fragmentation coring, and allergic reactions. Some examples of the material usage are for glass or plastic infusion bottles, IV bags, vials, syringe plungers, and insulin pens. Synthetic materials are produced under controlled conditions and are free of the impurities and lot-to-lot variations that are inherent in natural materials, including natural rubber. These materials are often compatible with EO, γ radiation, and steam sterilization. Other common synthetic rubbers currently used for pharmaceutical packaging are butyl, bromobutyl, chlorobutyl, silicon, neoprene, and nitrile. There are many advantages and disadvantages to consider for all elastomeric materials. It is important to thoroughly understand the requirements for the product before selecting a closure material.15,20,21,22
Plastic
Many pharmaceuticals are packaged in plastic materials. Some examples include plastic bags for IV fluids, plastic ointment tubes, plastic film-protected suppositories, plastic tablet, and capsule bottles. There are a vast number of different plastic materials and blends with a range of molecular weights, each with different properties and characteristics. Plastic materials are generally strong, lightweight, reasonably inert, and chemical resistant and can be designed from various polymers for select applications. At certain stages of the manufacturing process, many plastics can be
formed, cast, molded, or polymerized into a designed shape or form. There are two classes of plastics: thermoplastics and thermosets. The primary physical difference is that thermoplastics can be remelted back into a liquid, where thermoset plastics always remain in a permanent solid state.
formed, cast, molded, or polymerized into a designed shape or form. There are two classes of plastics: thermoplastics and thermosets. The primary physical difference is that thermoplastics can be remelted back into a liquid, where thermoset plastics always remain in a permanent solid state.
Thermoset plastics contain polymers that cross-link together during the curing process to form an irreversible chemical bond. The cross-linking process eliminates the risk of the product remelting when heat is applied, making thermosets ideal for high-heat applications such as electronics and appliances. Thermoset plastics significantly improve the material’s mechanical properties, providing enhanced chemical resistance, heat resistance, and structural integrity. Thermoset plastics are often used for sealed products due to their resistance to deformation. Thermoplastics pellets soften when heated and become more fluid as additional heat is applied. The curing process is completely reversible as no chemical bonding takes place. This characteristic allows thermoplastics to be remolded and recycled without negatively affecting the material’s physical properties. There are multiple thermoplastic resins that offer various performance benefits, but most materials commonly offer high strength, shrink resistance, and easy bendability. Depending on the resin types, they can be used for low-stress applications such as plastic bags or higher stress mechanical parts. Some properties of LDPE include ease of processing, moderate barrier to moisture, strength/toughness, flexibility, and ease of sealing. A common application is squeeze bottles used for nasal and eye drops. The HDPE is less permeable to gases and more resistant to oils, chemicals, and solvents. Some properties include stiffness, strength/toughness, and resistance to chemicals. It is widely used in bottles for solid dosage forms. The PP has good resistance to cracking when flexed and good resistance to heat sterilization methods. It is a colorless, odorless thermoplastic material with excellent tensile properties even at high temperature and excellent resistance to strong acids and alkalis.
The PS provides versatility, insulation, and clarity. The PS is used for jars for ointments and creams with low water content. Styrofoam, made from PS, is used for insulation and a cushion material for product protection. The PVC provides versatility, ease of blending, strength/toughness, resistance to grease/oil, resistance to chemicals, and clarity. It is commonly used as a rigid packaging material and main component of intravenous (IV) solution bags. The addition of elastomers to the resin can improve impact resistance. The PVDC provides excellent barrier properties against moisture, water vapor, UV light, aroma, inorganic acids, alkalis, salt solutions, soluble acids, hydrocarbons, detergent base materials, emulsifying agents, and wetting agents. Material coating weight can be customized depending on the barrier requirements of the product. The PVDC is very transparent, which can improve the aesthetics of the product. The PETG has outstanding thermoforming characteristics for applications that require deep draws with precise molding details. It has strong structural integrity and is typically not brittle. The PETG is a common material for thermoformed rigid trays for medical devices that require tray design elements to protect the product. All plastics can contain other substances, such as residues from the polymerization process, plasticizers, stabilizers, antioxidants, pigments, and lubricants.15,17,18,19
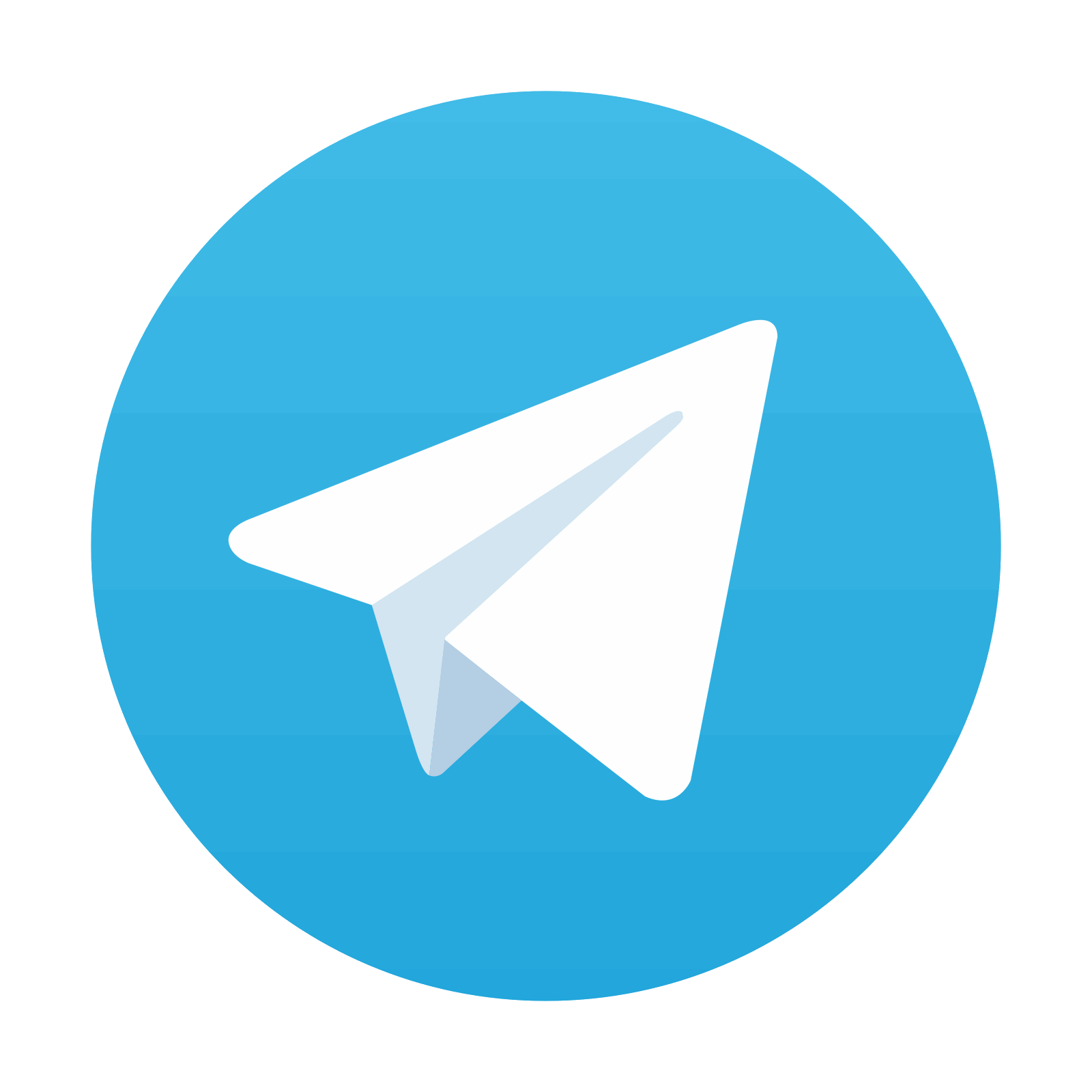
Stay updated, free articles. Join our Telegram channel

Full access? Get Clinical Tree
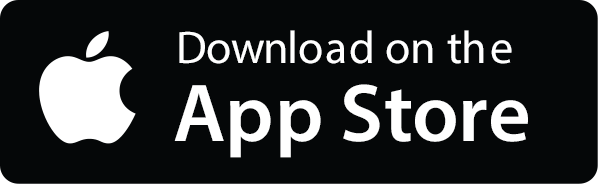
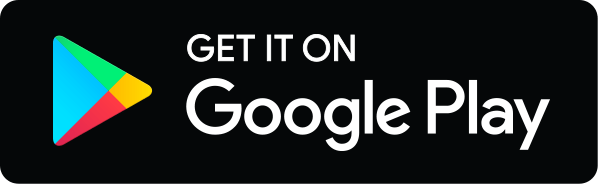
