11 Sterile Manufacturing Facilities
George Wiker
CONTENTS
Understanding the Principles of Sterile and Aseptic
Sterile Manufacturing Facilities
Primary Drug Product Processing
Process Design of Open versus Closed Systems
Processing in Barrier and Isolation Systems
Cleaning, Sanitization, and Sterilization
Primary and Secondary Containment
Design Considerations for Operation Interventions
Balance of Engineering with Procedural Solutions
Risk Created and Mitigated by Personnel
Risk Created and Mitigated by Equipment
Developing Project Drivers and Objectives
Project Concepts, Principles, and Considerations
Process Design and Architecture
Proper Material and Personnel Flows
Room Layout and Facility Configuration
Balancing Quality, Cost, and Schedule with Net Present Value
New Filling Techniques and Sterile Containers
Improved Environmental System Controls
Standardization and Harmonization
Risk-Based Approach and Risk Management
INTRODUCTION
The goal of this chapter is to provide a good design practice guideline for developing a sterile manufacturing facility. It focuses on the final formulation, filling, and finishing (initial packaging) of injectable products. Other products, such as inhalants, medical devices, and cell therapy, may be manufactured similarly to injectables, requiring a high level of integrity and protection. Thus, some of the principles and ideas presented here are applicable to those products. This chapter may help anyone who is developing a sterile manufacturing facility project to realize good design practices. It should be read in tandem with related agency guidelines and other guides, such as the International Society for Pharmaceutical Engineering (ISPE) Baseline Guide series.
Injectable products typically function by targeting specific regions or indications within the body. Such drugs, like vaccines and genetic therapies, are introduced directly into the bloodstream to be most effective and do not pass through the body’s natural defense mechanisms when ingested. The impact of a defective sterile manufacturing facility on injectable drug products can be catastrophic, so maintaining a very high level of control of product integrity and mitigation of risk is essential to human safety.
The term sterile manufacturing facility is used throughout this chapter as a description of a range of facilities that produce injectable products for humans. Chapters 10 and 18 have information regarding upstream (drug substance) processing and downstream (secondary) packaging of typical sterile manufacturing operations.
EXECUTIVE SUMMARY
By introducing a drug directly into the bloodstream, it reacts faster and with more intensity than other dosage forms. Therefore, the dosage must be free of any by-products or microorganisms that may adversely affect the body [1]. Also, many injectable products have limited stability, making the shelf life and storage conditions critical elements to the product’s effectiveness. Manufacturing and storage of these products are subject to regulatory compliance, and thus a high degree of effort is centered on current Good Manufacturing Practices (cGMPs).
The differences between sterile and aseptic processing are discussed, which is critical to understanding how these processing methods affect the design, operation, and testing of injectable products. The reader should be attentive to these processing methods.
Injectable dosage forms, or parenterals, comprise the largest portion of these manufactured products, and typically fall into one of two categories: large-volume parenterals and small-volume parenterals, which are composed of cytotoxic and noncytotoxic drug substance matrices. Other forms of injectable products include inhalants, cell therapy products, diagnostics, and compounded products. The facilities in which these products are processed are discussed in this chapter. The processing technologies have been influenced by developments in the processing of biologics and dairy products, where product sterility is essential. The design, construction, validation, and operation of these facilities have greatly contributed to the success of pharmaceutical sterile manufacturing facilities today.
In Western medicine, the use of injectable products first began in 1796 with Edward Jenner’s vaccination for smallpox (Figure 11.1) [2]. The use of injectable products expanded to include delivery of anesthetics, transfusions, and a variety of delicate drug matrices. The processing of these products expanded over the decades, and in 1987, the U.S. Food and Drug Administration (FDA) issued the “Sterile Drug Products Produced by Aseptic Processing” guideline. This guideline was issued under Title 21 of the U.S. Code of Federal Regulations (CFR), Part 10.90, and while it did not set legal requirements for aseptic processing, “it states the principles and practices of general applicability … acceptable to the Food and Drug Administration” [3]. With new and more delicate drug matrix developments comes a surge in the use of injectables as a method of effective drug delivery into the human body. Today, injectable products represent a significant portion of the total prescription drug delivery methods and are regulated by agencies all over the world. “Injectable dose formulation is the fastest growing segment, with a projected growth rate of 12.2% in 2012” [4]. “The global injectable drug delivery market [has] reached a value of around $22.5 billion by the end of 2012 and is forecast to grow to $43.3 billion by 2017. This estimates a compound annual growth rate of 14% between 2012 and 2017” [4].
“The increased focus on cytotoxics (toxic to cells to develop a therapy), lyophilized (freeze-dried) prefilled syringes, and reformulation of existing products is expected to drive the remarkable success of injectables. Lyophilization and manufacturing of sterile products, such as cytotoxics, are likely areas of growth potential, given the demand for oncology and high-potency drugs. Key growth drivers include increased pharmaceutical and biotechnological focus on complex disease areas, trends in disease control, growth in emerging markets, the pharmaceutical patent cliff (i.e., several blockbuster drugs losing patent protection), and reformulation of existing products. The major growth is expected to be cytotoxics as both cancer research and the development of new cancer therapies are driving growth in this area” [5].
FIGURE 11.1 A child receiving a smallpox vaccination. (Available at http://www.blatner.com/adam/consctransf/historyofmedicine/3-immunology/3-lecture.html.)
“The number of injectable drugs to be introduced in [the] long-term is expected to be significant [and] to outweigh the threat from advanced alternative drug delivery technologies. Additionally, increase[d] innovation in alternative technologies has [heightened] the focus of injectable market participants on issues, such [as] injector design, convenience, and painless modes of injectables” [6].
INJECTABLE PRODUCTS
Injectable products come in a variety of primary package forms, including ampules, vials, syringes, bottles, and bags (Figure 11.2). Injectable products, as defined by the characteristics of the drug matrices, are often rendered sterile via a number of qualified methods: suitable membrane filtration (i.e., 0.2 μm pore size or smaller to remove all microorganisms), ionizing radiation, dry or moist heat, and chemical sterilization.
Not every injectable product, due to the nature of the drug matrices, can be rendered sterile through the methods listed above, in which case the product must be processed aseptically. This often applies to vaccines, cell therapy processes, or drug matrices that are damaged or impacted by the above-mentioned sterilization methods.
UNDERSTANDING THE PRINCIPLES OF STERILE AND ASEPTIC
When discussing the processing of injectable products, it is very important to understand the definitions and differences of the terms being used; for example, the terms sterile and aseptic are often misused. Aseptic is an adjective that describes a condition where a substance or item is free of pathogenic microorganisms, as proven by appropriate sterility testing, showing a log reduction (see testing guidelines as defined by the agency with jurisdiction). Sterile is an adjective that describes a condition where a substance or item is free of all microorganisms, as proven by appropriate sterility testing (see testing requirements as defined by the agency with jurisdiction).
STERILE MANUFACTURING FACILITIES
In the spectrum of pharmaceutical manufacturing facilities, injectable product manufacturing facilities represent the most sophisticated and challenging to design, build, qualify, and operate, particularly when products manufactured in these facilities are rendered sterile and ready to inject directly into a human when complete. For this reason, very careful consideration is necessary when developing such a facility. Key measures that make sterile manufacturing facilities unique include highly controlled room environments (equal to Grade A or C Class A/ISO 5), unidirectional flow of materials and personnel, highly sophisticated and controlled sterile or aseptic filling systems, clean-in-place (CIP) and steam-in-place (SIP) systems, complex equipment and components, and intensive utilities and heating, ventilation, and air conditioning (HVAC) systems (Figure 11.3).
FIGURE 11.2 Packaging for injectable products.
FIGURE 11.3 Controlled room environments.
Generally, there are two types of processing operations within a sterile manufacturing facility: primary bulk processing of the drug substance, and the formulation, filling, and finishing of the drug product into its final dosage form. Testing of diagnostic kits, medical device assembling, cell therapy processes, and in-process product testing are examples of other types of operations carried out in a highly controlled sterile or aseptic manner.
Major sterile manufacturing operations include component preparation in ultrasonic sinks, autoclaves, and other wash and preparation equipment; compounding and formulation by mixing and blending several product components in either fixed or portable tanks or mixing systems; filling, which ranges from hand-fills under a hood to a fully automated high-speed container filling system; freeze drying (lyophilization) or removing water from a drug product or dose for greater stability and longer shelf life; inspection, ranging from a manual inspection by operators to a fully integrated multifunctional inspection system; and process utilities by direct-impact systems, which support manufacturing, including water-for-injection (WFI) and clean steam generators, as well as the supply of sterile air or gases and other product contact utility supply systems.
PROCESS TECHNOLOGIES
At the core of the sterile manufacturing operation, process technology drives the ability to safely, efficiently, and repeatedly produce sterile products. Early sterile manufacturing facilities centered on aseptic processing (often open processing) in a clean room environment with personnel in the critical work area. This approach created the risk of particulate and bioburden contamination. Thus, as sterile manufacturing technologies and practices developed, the drive to close the process and separate the critical manufacturing environment from personnel became a key driver in the development of the core process technology. Today, the most commonly prescribed approach is to close the manufacturing process and locate it behind a fully contained International Organization for Standardization (ISO) 5 isolator system in an ISO 7 or ISO 8 background clean room environment. In contrast, when the ISO 5 zone is open to the surrounding environment, the background environment is commonly designed to meet ISO 5 or ISO 6, thus increasing the complexity and management of materials and personnel.
DRUG PRODUCT PROCESSING
The principles and approaches to cleaning and sterilization are the centerpiece of technological development, evolving from manual operations recorded on paper (by hand) to fully automated cleaning and sterilization systems with compliant electronic recording devices. The primary drug substance is made from either a biological or chemical process, producing a bulk active pharmaceutical ingredient (API). These processes are discussed in detail in Chapter 10.
Table 11.1 outlines the major steps for the secondary processing of the drug product and shows typical room cleanliness classifications for European Commission (EC) guidelines.
Primary Drug Product Processing
Drug product compounding, also known as formulation, is the basic preparation of a drug product for final filling. It includes the final preparation of a product through the dilution, concentration, or other preparation of a mixture of approved pharmaceutical ingredients into a bulk quantity. This process can range from a simple, one-step dilution to a multistep process of homogenization. The batch is sampled, and when the process is complete, it is then quarantined, tested, and released for final filling and finishing.
TABLE 11.1
Room Function and Classifications
FIGURE 11.4 Vial filling operation.
Drug product filling in a sterile facility consists of the transfer of a bulk formulation (prepared in the same facility or elsewhere) into a dosage form for patient administration. Dosage form containers typically consist of bags, vials, and syringes, and the final product may be either in liquid form or lyophilized (freeze-dried) if required [4, 5]. Figure 11.4 is a simplified diagram showing a vial filling operation.
Processing Scales
When developing a facility program, the intended scale of manufacturing drives many decisions that impact design and operation. The scale of manufacturing determines the methods and approaches. In a developmental-scale facility, design solutions may call for manual operations and administrative procedural solutions rather than fixed, automatic, or complex engineering solutions. In a commercial-scale facility, the design leans toward automatic operations and engineered systems, including redundancy and robustness. There are four main scales of processing of products that are described below.
Developmental scale. This is a processing scale where the drug or drug matrix is developed from the bench scale to a measured quantity. Considerations of eventual scale-up to larger volumes are essential. Many processes are carried out manually, so having a firm grasp on standard operating procedures (SOPs) is prudent. Careful consideration of drug toxicity is also critical here, since the process may need to be highly contained to protect operators. In this case, creating a contained process that is also scalable is essential.
Clinical scale. A clinical scale concerns the manufacture of product for integrity and patient testing. Processing is still manual and controlled through procedures. Engineering systems and controls, however, are employed, especially for critical steps and data collection. Some automatic features may also be included as the process develops through clinical trials, along with tighter controls in practice. The scalability of the process is further developed, so that when the product reaches agency approval, the process capacity is scalable to meet market launch demand. As the product progresses through clinical trials, the process typically moves toward a more uniform, consistent, and repeatable operation.
Launch scale. Once a regulatory body has approved a product for commercial use, larger quantities are needed to satisfy product launch into the marketplace. Launch-scale quantities are often made from the clinical-scale facility. Typically, as a product moves through phase 3 clinical trials, sourcing decisions are made to either build a dedicated facility or contract with another company for large-scale manufacturing capacity. Some companies have operations set up specifically for new products being introduced into the marketplace, while a full commercial-scale facility is prepared for operation.
Commercial scale. Commercial scale is a full-scale process operation designed to meet marketplace demands for one or more products. The process operations are well defined and developed, with automatic or engineered methods of processing employed. The facilities are large and expensive, providing high reliability with risk managed through complex engineered solutions and administrative procedures.
Process Equipment
Sterile manufacturing operations are rigorously scrutinized for integrity and consistency to maintain patient safety. Accordingly, process equipment supporting or controlling sterile operations is designed to meet strict regulatory guidelines and design requirements. Major design considerations in process equipment include operability and ergonomics; cleanability of the system; ability to sterilize the system; drainability; smooth, hard, and crevice-free finishes of all product contact surfaces; fully controllable, consistent, and repeatable functions (manual or automatic); closed versus open process systems; and the ability to control the manufacturing environment to a prescribed level.
Materials of construction for sterile manufacturing equipment typically comprise 316L-grade stainless steel, designed for cleanability, strength, durability, and especially sterilizability. Stainless steel contact surfaces often meet very high standards, consistent with interior surface finishes as defined in Part SF, “Stainless Steel and Higher Alloy Interior Surface Finishes” [6].
Process Design of Open versus Closed Systems
Issues related to closed versus open systems significantly affect the development, size, cost, and operation of a sterile manufacturing project and, as such, become a top priority in the design of a process. In comparison, while closed process systems require greater design and operational integrity to function consistently in a controlled manner, open systems often require more real estate in a facility and add complexity of access or egress into a critical environment, so a comparative understanding of each approach is very important in the development and operation of a facility.
An open process system is a system that is exposed to the background environment in a processing facility. Such examples include final filling into dosage forms, loose connections in a process system, testing of samples, and open transfer of product within a clean room or biosafety cabinet (BSC). An open system used to process an injectable product that cannot be maintained in a closed state is typically located within a Grade A or ISO 5 environment. This approach requires rooms and functions to support this critical operation. For example, a closed process system, occupying 500 ft2, may grow to as much as 2,000 ft2 to accommodate support and background features for an open system.
A closed system is commonly defined as a process that has no normal potential exposure to the surrounding environment. This system may comprise multiple- or single-unit operations. A closed system can be opened initially for cleaning or product or parts changeover, but it is then intrinsically closed and SIP before use in a process operation. When a system can be operated and maintained in a closed state, it has been proposed that the background environment may be significantly downgraded. In most cases, though, the background is maintained to a determined level regardless of a closed process state due to conservative design practices (i.e., engineering solutions over procedural solutions) and conservative risk management considerations.
The design should reflect the number and frequency of connections made to the process system, as well as the method of connection, before declaring a system closed or open. The design should consider that the system may be required to be closed to contain a process because of operator exposure limits to certain drugs and drug matrices. When a level of segregation is required, barrier or isolation systems should be considered along with personnel protection (see Chapter 14).
Processing in Barrier and Isolation Systems
Barrier and isolation systems represent the most common approach in the design and operation of sterile facilities. A restricted access barrier (RAB) isolation system is a barrier system consisting of a set of glass doors set into a stainless steel frame that surrounds the critical filling environment. Due to the nature of this system, interventions during processing, as well as the loading and unloading of material, must be clearly understood, since these aspects often require the background environment to be highly controlled to mitigate risk caused by the opening of the RABs into the room (Figure 11.5).
An isolation system consists of a stainless steel and glass enclosure system, with glove ports, creating a totally sealed system for the critical environment. These technologies are very different, so a comprehensive understanding of the impact of either technology selection is a key part to following good design practices (Figure 11.6).
Benefits to these technologies include protection of product, containment of potent and cytotoxic compounds, protection of personnel, and the potential ability to reduce the environmental classification level of the background environment.
While an isolation system may cost more than a RAB system, the positive effects of both reduced background environmental requirements and operational benefits often exceed the additional initial cost as measured by life cycle costs. Some process systems and unit operations may require a variety of interventions during an operational run; thus, understanding the benefits, limits, and risks of barrier and isolation systems is important during early conceptual design and development. Integration of a barrier or isolation system with process equipment often requires the process equipment to be fabricated and then sent to a vendor specialist to locate the process component in the barrier or isolation system. Consideration of schedule and cost should be made when considering process equipment vendor options. Further dialogue on barrier, containment, and isolation systems can be found in Chapter 14.
FIGURE 11.5 An isolation system. (Courtesy of Bosch Technologies, Palo Alto, CA.)
FIGURE 11.6 Barrier and isolation systems. (Courtesy of Cook Pharmica, Bloomington, IN.)
CLEANING, SANITIZATION, AND STERILIZATION
Any equipment, materials, and systems that offer product contact surfaces in a sterile manufacturing facility must be free of all viable microbial organisms on or in inanimate surfaces [7]. To achieve this, any contact surfaces must be thoroughly cleaned and sterilized. Consistent and thorough preparation of product contact surfaces represents a great challenge, so intensive design efforts are required to achieve a fully qualified operation. To mitigate risk or achieve an economic advantage, a company may opt to purchase rather than produce certain sterilized raw materials and disposable products.
Cleaning Process Equipment
While nonproduct contact equipment, such as tables, racks, carts, and so forth, is cleaned at intervals, product contact equipment, such as tanks, pumps, and piping, must be cleaned routinely (between different product runs or batches). Product contact equipment also consists of fixed and portable equipment. Fixed equipment is disassembled with some components removed from the room for cleaning-out-of-place (COP) in a purpose-built room. This also includes the removal of portable equipment for cleaning, typically in the same location. Remaining components are CIP by flushing the system with a series of solutions and rinses while the system is closed. Control of cleaning fluids is managed by both the process equipment and CIP system (typically a skid) control units. The COP typically consists of further disassembly of equipment, where it is cleaned along with an ultrasonic-type or detergent-flushing cleaning cycle (semiautomatic or automatic). A final rinse and drying step completes the cleaning process. Cleaned equipment is then reassembled (if required) and may be placed into a container or bag for protection and sterilization. As the equipment moves through the cleaning process, the surrounding environment increases in cleanliness to correspond with the state of the equipment being cleaned. This typically means that the rooms will be designed to meet a Grade D or C environment, with local Grade B or A areas as required.
Sanitization
Sanitization, in comparison to sterilization, is the “process of substantially reducing or destroying a number of microbial organisms to a relatively safe level.” Sanitization “generally requires a 99.9% or greater reduction of a test organism.” The “test organism should be agreed upon with the inspecting agency” before completion of the design and validation of the process [8]. In general, most agencies, designers, quality assurance (QA) personnel, and operators prefer that a product is rendered as being sterile as close to the end of a process cycle (i.e., bioburden is controlled throughout the process) as possible. Ideally, this is realized through terminal sterilization, in which filled containers of product pass through a prescribed process, consisting of heat sterilizing the product at a fixed range of parameters (Figure 11.7).
Equipment Sterilization
In the development of a sterile facility and operation, understanding the meaning of a term and the effect of declaring it in a cGMP environment is extremely important since once that term is declared, it must be maintained. This is similar to the previously discussed difference between the terms aseptic and sterile. One such declaration is the need to declare a system either sterilizable or sanitizable.
Once a process room and equipment have been cleaned, product contact components and other critical items are sterilized. To claim that a system or unit operation is sterilized or sterilizable, one must prove that the system can consistently and repeatedly “destroy all forms of viable microbial organisms on or in inanimate surfaces.” Sterilization is usually required for the following reasons: to prevent contamination, to protect product, to protect the patient, and to ensure that only a certain product is present [8]. Critical design factors for sterilization are the material compatibility with the sterilization method, the design elements of time, the environmental conditions, the temperature, and the chemical contact [9].
FIGURE 11.7 Aseptic process cycle.
Equipment SIP is typically achieved through the introduction of pure steam in a closed system for a prescribed interval of time and temperature. Equipment and components that cannot be sterilized in this manner must be removed from the system and installed in an ISO 5 environment before a process operation. These typically consist of silicon or plastic materials used for disposable tubing, containers, or other components; they can be chemically sterilized, irradiated, or purchased already sterilized.
Once a level of sterility has been achieved in an injectable operation, that level must be maintained from that point forward to maintain that product’s declared integrity. Thus, having a clear understanding of why, when, and how a product in a process is to be sterilized is very important.
Monitoring Sterility
Once a product is rendered sterilized, that level of purity must be maintained and tested to ensure product integrity, so a clear understanding should be had early in the development of a project as to the method of monitoring batch integrity.
The QA team will verify that a process room has been cleaned through testing before commencement of a process operation, through the sampling of surface areas in a room, as well as the measurement of microbial organisms and particles. This is typically performed in accordance with the environmental tables of classifications (i.e., tables D and E). In a sterile manufacturing facility, process utilities, such as water, steam, air, and other gases, are rendered as pure or sterile so that the product integrity is not compromised during processing.
Primary and Secondary Containment
Every process operation in place today essentially has a primary and a secondary level of containment. The most common type of primary containment is a process equipment or system (ideally closed), and the process room and surrounding environment are the secondary level of containment. Examples are given in Table 11.2.
When targeting an efficient cost structure for a project, a review of the background environment (the secondary containment) should be done first, since often this costs more than the process equipment (the primary system), while offering no more manufacturing capacity. For example, in a typical sterile manufacturing facility, the process equipment consumes 10%–35% of the total cost of the project, compared to 30%–60% for the background environment and other costs. Thus, any reduction in the background environment is significant to the overall cost of the project, while not affecting process capacity. Space planning and management continue to be critical aspects of understanding the best approach to designing an efficient sterile manufacturing facility.
Often, the secondary containment element (i.e., the room or local protection) is required to perform at a certain level, since the primary containment system may require an opening or break in the system during a process run, or the product may have a containment or exposure limit requirement. In this case, the secondary containment element becomes an important line of protection to maintain product integrity, protect operators, and contain a product within a certain boundary.
TABLE 11.2
Primary and Secondary Containment
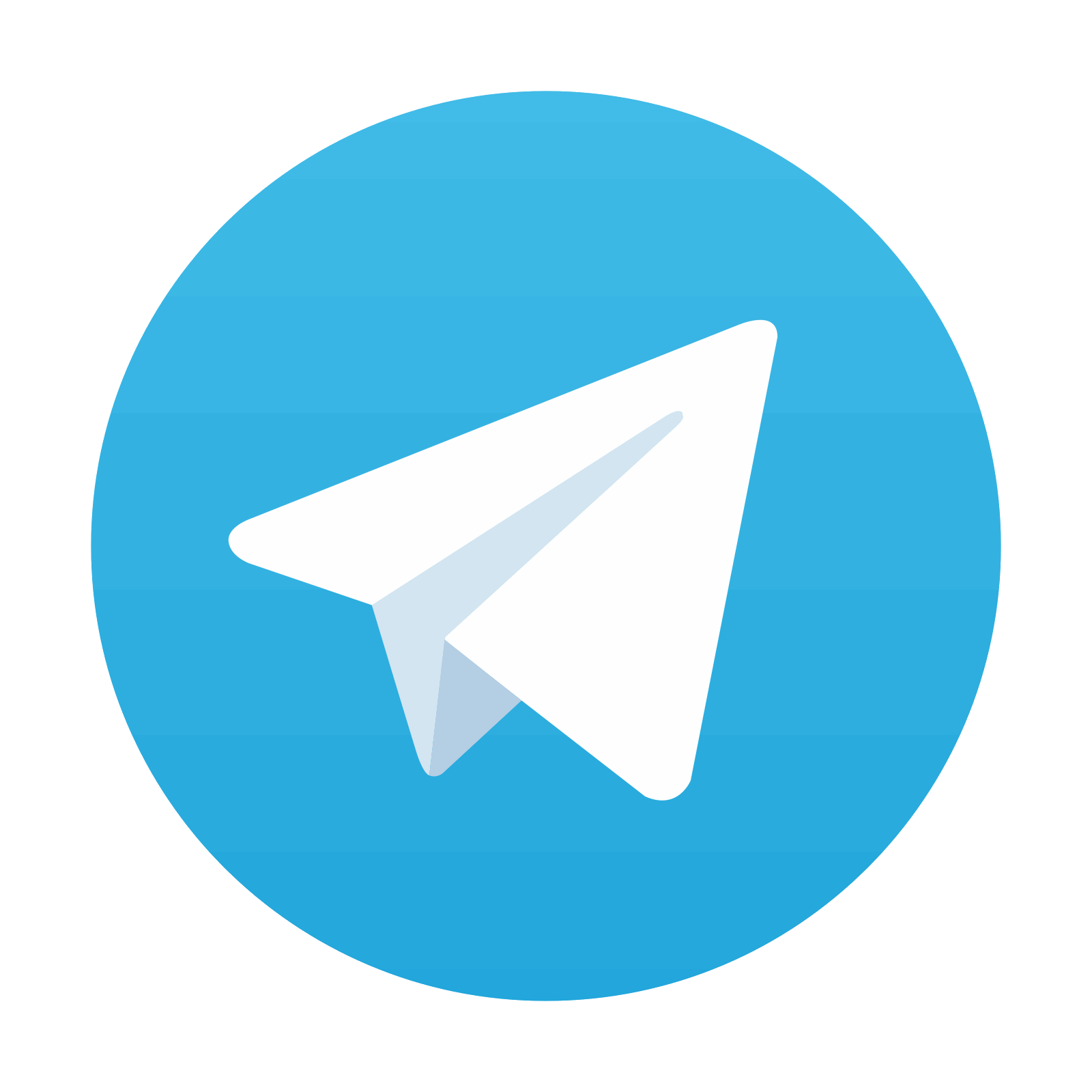
Stay updated, free articles. Join our Telegram channel

Full access? Get Clinical Tree
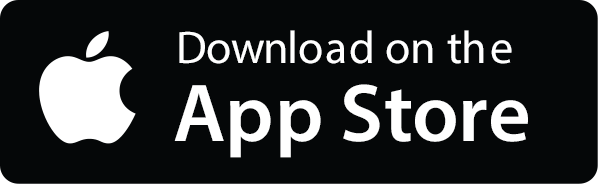
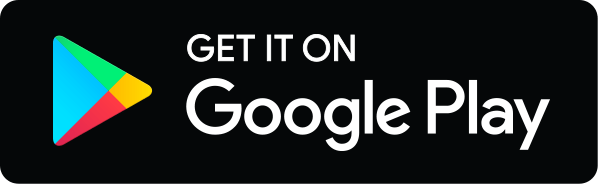