(1)
Faculty of Medicine of Montpellier, Montpellier, France
Abstract
The spine is a complex biomechanical structure made by articulated vertebras in fixed position (postures) and moved in different directions by muscles. The spinal stabilisation is automatically regulated by a spinal servomechanism with three inputs: muscle transducers for the spinal balance, skin on the axial line of the spinal processes for global control of position and movement and vestibular utricular macula for the horizontal stabilisation of the head (inverse pendulum). A first group of muscles goes from spine to superior and inferior limbs and a second group is fixed directly on the spine (spinal muscles), and their complex architecture is now better known. Each segment has its own functional feature. The cervical spine is in close relation with vision, justifying the cardan joint for the occipitocervical junction and the specific protection system of the vertebral artery, the artery of life. The dorsal spine is linked to the respiratory function. The lumbar spine is articulated with the broken pelvic ring and its deformable joints: two posterior sacroiliacs and one anterior pubic in order to reinforce mechanical resistance of it. Rachidian pains have to be well clinically identified, and a particular emphasis has to be put on the important role of the spinal ganglion.
2.1 Introduction
The spine is an axial rod made of overlapping articulated bone pieces mobilised by muscles. It supports the cephalic mass, is leaning on the pelvic base and gives attachment to the upper and lower limbs. It has several segments with specific rules requiring to be studied separately, even if the spine is a very interactive system finding its unity in the complex function of spinal stabilisation.
In mechanical terms, the spinal beam is subjected to large stresses in compression that corresponds to the problem of compressed linear vertical beams generating buckling. At a maximum level of compression and even measuring strain, the direction of the rupture becomes unpredictable. It depends essentially on the degree of the homogeneity of the material. To avoid buckling, the most efficient system is to anticipate the direction of the deformation until rupture by bending the beam which makes it predictable and allows to avoid it by using correctly positioned shrouds. This is the case of the spine which is formed by a succession of reversed curves, with bracing muscle placed in the concave part of the curves, except at the thoracic level due to mechanical transverse continuity given by the rib cage. The newborn, which has not yet walked (except some intrauterine trampling reassuring the mother), has not acquired the reverse spinal curves at birth. The chimpanzee that is not using a permanent bipedal posture has a spine with a single curvature.
2.2 Spinal Stabilisation [1–5]
This is the function that helps maintain spinal posture in three positions: flexion, extension, lateral left and right. It is dependent on the morphology of the vertebral triangle segmental support and especially the organisation of the muscles. This factor is predominant in all spine pathology and is not sufficiently taken into account, while modern imaging with new modalities (CT and MRI) allows for very precise anatomical images closer to the reality than conventional vector models. This function, for the same reason related to the ignorance of the pilot, is highly automated and integrated into what has to be called the spinal servomechanism. Like any servo, it has inputs that transmit imbalance information in order to induce stabilisation reactions by impulsions.
2.2.1 Spinal Servomechanism Inputs
They are three: the proprioceptive muscle sensors of the spinal balance, the cervico-thoracoabdominal skin and the gravity sensor utricular macula of the vestibule.
2.2.1.1 Spinal Balance
It is defined as a system of two active right/left pendulums formed by spinal muscles attached directly to the spine like the shrouds and capable of exerting a pulling force on the spinal axis. The myotatic reflex we have described from stretching the muscle spindle will be the key for the spinal balance. Apparently, it determines paradoxical reactions that Isch has defined a long time ago by electromyography. Bending the spine on the right creates a concave curvature, and it is found that the muscle activity is on the side of the convexity to the left in relation to the stretch of the muscle spindles from this side. This is the same phenomenon which can be observed by placing both thumbs on the lumbar paravertebral mass and asking to walk in place. The lifting of the lower limb creates a fusorial stretching on the opposite side with an automatic contralateral muscular contraction. It is clear that the overall contraction of the spinal muscles is managed by spinal cord but also by supraspinal levels including the cerebellum.
2.2.1.2 Axial Goniometric Skin Reference Line
As we explained above, the skin is the goniometer of all the body segments, which allows isolating within the spine an axial skin baseline attached to the spinous processes. This axial reference line contributes largely to the cutaneous perception of spine movements. It can be disrupted in axial postoperative scars. In addition, it is possible to define a supraspinal fibrous complex (Fig. 2.1) which links the vertebrae together and is made by five anatomical structures: the skin (epidermis and dermis), the flat tendon of latissimus dorsi muscle, the thoraco-lumbosacral aponeurosis around the spinal muscles, the lumbosacral tendon and the supraspinal ligament which is not the strongest structure.


Fig. 2.1
Axial section of L3. 1. Tendon of the latissimus dorsi; 2. Thoraco-lumbo-sacral aponeurosis; 3. Ilio-costal muscle; 4. Quadratus lumborum; 5. Kidney; 6. Psoas muscle; 7. Facet joint; 8. Long dorsal muscle; 9. Transverso-spinal muscles (multifidus); 10. Spinal process; 11. Supraspinal fibrous complex
2.2.1.3 The Gravity Utricular Transducer
The cephalic mass based on the spinal rod is an inverted pendulum which plays an important role in stabilising the spine. It is desirable to achieve a proper balance of the system that the head is placed in horizontal position. This cannot be given by the visual system, because we have two eyes, but we only see one image while two right and left sensors are needed for good horizontal head stabilisation. This justifies the existence in the vestibule of the inner ear of a gravity transducer, sensitive to vertical forces (Fig. 2.2). The utricular macula plays this role. It is formed by a thin bone lamella which is placed horizontally and covert by a mucous membrane reinforced with crystals. This changes the inertia of the system and creates what is called a flyweight in mechanics. It is not exactly the same system as fish or shellfish also have, a sensor formed by otolith, a calcareous concretion based on sensory cells, which allows the animal to know where the top and bottom are, allowing good navigation. An upside fish in water is nearing its biological end. In humans, it is called otolithic membrane to characterise the mucosa overlying bone lamella and sensory cells it contains. This horizontal stabilisation of the head intervenes significantly in the spinal stabilisation, and now we come to think that some adolescent idiopathic scoliosis, without apparent cause, may come from a functional deficiency of the utricular macula.


Fig. 2.2
Utricular macula on a sagittal section of temporal bone. 1. Posterior semi-circular canal; 2. Cerebellum; 3. Lateral sinus; 4. Tympanic membrane; 5. Tensor tympani muscle in its bony canal; 6. Geniculate ganglion (VII); 7. Utricular macula
2.2.2 Muscular Corrections
Each vertebra has medial tendon attachments on spinous processes and lateral on lamina and transverse processes, as well as at the thoracic level on the posterior angle of the ribs. An economic spinal posture corresponds to total muscle relaxation obtained by a back support that neutralises the effects of muscle spindles stretching, but has the disadvantage to distend the posterior facet joint capsule, which can generate pain. The upright posture involves minimum muscle contractions for incomplete straightening. Posture leaning forward, however, requires significant contractions quickly painful in untrained subjects.
2.2.2.1 First Group: Spino-appendicular Muscles
The first group of muscles is attached to the spinous processes and activates either the upper limb: trapezius, rhomboid, serratus anterior, and latissimusdorsi; or the lower limb with a spinal action: iliopsoas; or the lower limb only: maximus medius and minimus glutei, tensor fascia lata, rectus femoris, adductors, medial and lateral obturators (Fig. 2.3).


Fig. 2.3
The spine muscles. (a) Posterior view of the trunk: 1. Trapezius muscle; 2. Latissimus dorsi muscle; 3. Posterior and inferior serratus; 4. Serratus anterior; 5. Thoraco-lumbo-sacral aponeurosis; 6. Rhomboid muscle; 7. Scapula reclined outside. (b) Lumbar scanner of an axial section: 1. Psoas muscle; 2. Multifidus; 3. Long dorsal muscle; 4. Ilio-costal muscle. (c) Axial section of the abdomen: 1. Spinal muscles; 2. Psoas muscle; 3. Abdominal wall large muscles. (d) Spinal muscles: 1. Long dorsal muscle; 2. Lumbosacral tendon
2.2.2.2 Second Group: Spinal Muscles
The second group, the spinal muscles, has a specific action on the spine. Bonneau [9] in his thesis gave a comprehensive description. We must remember the following points (Fig. 2.4):


Fig. 2.4
Dissection of spine muscles. (a) Posterior view: 1. Latissimus dorsi; 2. Posterior and inferior serratus minor; 3. Transmuscular cutaneous nerves; 4. Lumbosacral tendon; 5. Ilio costal muscle; 6. Long dorsal muscle. (b) Sagittal section of lumbar spine: 1. Skin, aponeurosis and lumbosacral tendon; 2. Deep oblique muscular fibres. (c) Sagittal section of sacral spine: 1. Lumbosacral tendon; 2. Coccyx; 3. Rectum 4. Sigmoid colon. (d) Lateral view of lumbar spine after reclining the aponeurosis: 1. Thoraco-lumbo-sacral aponeurosis; 2. Iliac crest; 3. Posterior and inferior serratus minor. 4. Iliocostal muscle; 5. Lumbosacral tendon. (e) Lateral view: 1. Reclined lumbosacral tendon; 2. Deep muscular fibres of long dorsal; 3. Transverso-spinal muscle (multifidus); 4. Skin gliding plan. 5. Spinous processes
there is on the back under the skin a fibro-tendinous plan consisting, from the surface to the depth, of three formations: first the flat tendon of the latissimus dorsi from T6, second the thoraco-lumbosacral aponeurosis surrounding spinal muscles and encompassing within its deep surface the two mechanical tensors—superior and inferior serratus minor—and third the extremely resistant lumbosacral tendon forming a real posterior fibrous wall. This tendon is strongly attached to the lumbar spinous processes and the banks of the iliosacral groove till the coccyx. It represents the tendon for laterally iliocostal muscle and medially long dorsal muscle that fit with multiple chevrons on posterior angles of the ribs and transverse processes. Note that on the deep surface of the lumbosacral tendon are fixed a very thick oblique muscle fibres package that provide much of the force required to erect spine [10];
below the fibrous plan, spinal muscles [11, 12] are arranged in three columns:
lateral, with the long dorsal muscle which has lumbar, thoracic cervical (neck transverse T1–T5/C3–C6) and cephalic portions (head transverse T3–C3/mastoid process);
intermediate with the iliocostal muscle having lumbar, thoracic and cervical portions (back angle T4–T8/transverse processes C4–C7);
medial with the transverso-spinal muscles called multifidus which is formed by 23 beams overlapped in the craniocaudal direction like tiles on a roof. Each beam has four different parts: the short lamellar (crossing one vertebral segment), long lamellar (two segments), short spiny (three segments) and long spiny (four to six segments).
It must be put in place after the muscles with a special place: the yellow ligament (Fig. 2.5). It is responsible for a resilient damping of the spine movement of flexion. So called because of its yellow colour due to a great number of dense elastin fibres it contains. It is the only true elastic ligament of the body. It is attached to the superior and inferior border of the laminae and gives a viscoelastic amortising return in the spine bending movement. It is present at all spinal levels and its morphology on the images of the spine is clearly related to the state of the disc [13, 14]. It appears relaxed and expanded when the disc collapse (pseudo-hypertrophy). It can also reverse its normal curvature by protruding into the spinal canal and causing an acquired narrow canal (soft stenosis) which can create neurological disorders that may require surgical recalibration of the canal by a minimally invasive approach. At the lumbar level, it is clearly visible on an axial section of the spine that it is shaped like a V covering posterior facet joint processes allowing to “smooth” the dorsal part of the foramen. Like all fibrous structures, it tends to involute with age, resulting in a decrease of elastic fibres and fibroblast proliferation, creating an image of true hypertrophy (Fig. 2.6).



Fig. 2.5
Cervical yellow ligament. (a) Oblique sagittal section: 1. Vertebral body; 2. Spinal process; 3. Superior border of lamina; 4. Dura mater; 5. Spinal cord. (b) Sagittal section: 1. Cervical roots; 2. Posterior opening of the vertebral body vein covert by the posterior common vertebral ligament; 3. Yellow ligament

Fig. 2.6
Lumbar yellow ligament. (a) Axial section of L1: 1. Vertebral body L1; 2. Spinal cord; 3. Facet joint with pseudomeniscal synovial fold; 4. Yellow ligament. 5. Fat pad; 6. Vertebral foramen. (b) Coronal section of the posterior vertebral arch: 1. Lamina; 2. Facet joint; 3. Fat pad; 4. Yellow ligament; 5. Intertransverse muscle
The enormous complexity of these muscles responds to the complex problem of stabilising the multi-segmented spinal rod and managing its movements. The human pilot of the machine does not feel this complexity unless its muscles become painful, sometimes creating an attempted involuntary automatic active spine immobilisation called lumbago.
Finally, to conclude this section on spinal stabilisation, some comments have to be given about pathomechanics. It seems not appropriate to talk about instability and a fortiori about microinstability to characterise abnormal stabilisation of one or more vertebral segments. In fact, in the official terminology of mechanics, the definition of an unstable structure is a structure that cannot be stabilized. That obviously is not the case of pathological spine. This is actually an abnormal stabilisation with hypo- or hypermobility that we must call a distability. This change in terminology will surely take a lot of time, but hopefully at least the readers of this book will agree to adopt this new terminology more consistently with the rigour of mechanics.
2.3 Cervical Spine and the Eye/Head Servomechanism
The cervical spine is the “head holder” and its mobility is directly related to that of the cephalic mass. A simple functional analysis helps to understand that the head movements are mainly related to the visual exploration of space in two main directions: vertical and horizontal. A blind person who lost this visual exploration is recognised by its cephalic immobility. This implies movements in flexion/extension and horizontal rotation of the cervical spine [15–18].
2.3.1 The Visual Programme
To understand the functional nature of these movements, it is essential to analyse the visual program. Vision allows perceiving shape, colour and movement of objects in all directions. A further refinement, the third dimension (X, Y, Z), can be collected by stereoscopy (3D vision). This is possible, thanks to the existence of a gap between the two eyes placed, in humans, in a frontal position. The major technical problem is the fusion of the two images pixel by pixel. That can be done by the optic chiasm, which crosses at 50 % the retinal fibres and sends to the area of primary visual cortical projection of each occipital lobe two—left and right—half images. But the perfect fusion can only be made with a high resolution point placed in the retina of the eye on the optical axis. The macula gives 80 % of the vision and represents an extraordinary concentration of visual cones (135,000 mm2). Peripheral vision is in fact less accurate.
At birth, it may happen that the two eyeballs are not perfectly centred, making impossible to merge the two retinal images for stereoscopy. Therefore, an amblyopia is detected in children, with a normal eye appearance but with a reduced acuity on one side. This is due to a macular active inhibition of this eye by cortico-retinal fibres described by Mawas. In other words, this means that the constructor has not only organised the complex establishment of vision, but he also predicted the failure. The treatment consists in blocking the good eye in order to disinhibit the other and then trying a realignment of the two visual axes by orthoptics or surgery, with rather mixed results.
In comparative anatomy, punctiform macula exists only in animals with stereoscopy: owls, raptors, cats, etc.; the others are using a panoramic vision with a macula in band as for ruminants which have low binocular vision field and two different retinal images which cannot be merged to give stereoscopy. Only the chameleon combines both types of vision: first panoramic when he looks at his prey using completely separate both eye vision with small pupillary opening for precision and second stereoscopic by converging the two eyes and merging the two images, in order to guarantee the success of its quick tongue lick on its prey. It has a nice punctiform macula (Fig. 2.7).


Fig. 2.7
The chameleon. (a) Independent movable eyes (panoramic vision). (b) Convergence of the two eyes to catch a pray with tongue projection (stereoscopic vision)
In humans, the two eyeballs are moving along two axes: vertical and horizontal, but beyond 30 degrees of horizontal rotation, stereoscopy is impossible because of the image of the nose and optical parallax problems. We can deal with this problem by finding the solution of moving at the same time the head and the eyes, which is the eye/head servomechanism. But it is obviously necessary to coordinate and adjust the two movements, which means for the brain to know accurately the speed and direction of the movements of the head.
This explains why the vestibule, which has a three-dimensional angular accelerometer with three semicircular canals positioned orthogonally in the three planes of space and is able to measure very precisely direction and speed of the mobile head, was placed within the skull base. It could indeed be placed in the body since it can also quantify body movements in 3D, as, for example, when walking without visual control at night.
In addition, the vestibule and the cochlea have two different functions (stato-dynamic equilibration of the head and hearing) but are linked topographically in the same organ, the ear. The reason for that could be presumably found in their common technical problem: the precise osmotic regulation of their two perilymphatic and endolymphatic liquids. In fact it seems more intelligent and economical to put them together. Vertigo in Meniere’s disease is the pathological illustration of this successful joint programme.
This also explains the important role of the 3D space reference of the vestibule that requires to be fixed very early in a dense bone area of the skull base. This is why semicircular canals and attached cochlea are the first organs with full growth at birth (Fig. 2.8e). It remains the problem of left-right symmetry in the adult semicircular canals. Caix from Limoges showed a low percentage of subjects with symmetrical canals and that could be a factor influencing susceptibility to vertigo.


Fig. 2.8
Axial sections of the cardan joint. (a) Section of the atlanto-axoidal joint: 1. Anterior arch of atlas; 2. Odontoid process of C2; 3. Transverse ligament of C1 (transverse part of the cruciform atlantis ligament). (b) Superior view of atlas/axis: 1. Superior articular surface of atlas; 2. Odontoid process of C2; 3. Transverse foramen of C1; 4. Posterior arch of C1. (c) Axial section at the foramen magnum: 1. Alar ligaments; 2. Longitudinal fascicles of the cruciform ligament; 3. Posterior inferior cerebellar artery (PICA); 4. Cerebellar tonsils; 5. Roots of C1 cervical nerve; 6. Vertebral artery. (d) Axial section of the C1/C2 junction: 1. Vertebral artery; 2. Odontoid process of C2; 3. Inferior oblique muscle. (e) Dissection of a skull base of new born: 1. Lateral semicircular canal; 2. Cochlea; 3. Three semicircular canals (size of the adult). (f) Inferior axial section of the cardan: 1. Atlanto-axoidal joint; 2. Horizontal loop of the vertebral artery behind the lateral mass of C1
Finally, the eye/head servomechanism has to coordinate movements of two different anatomical systems. It needs a common guide, which is the image perceived by visual pathways. It is interesting to note that with the artificial vision of robotic systems, it is the same problem and the same solution: give to images the crucial role of guiding servomechanisms.
This focuses on the very rich interactions between vision and vestibule illustrated by many reflexes that have been described. Eye is helping vestibule, which is a liquid sensor with a remanence (excitation maintained after stopping motion) by readjusting it (reset). Classical dancers, although they are not physiologists, know that to perform a “pirouette” perfectly (vertical rotation of the body) they must take within the environment a fixation point at each rotation (or the light from the balcony or the shiny bald head of the conductor…), explaining the separate head and body rotation.
The waltz, which has still escaped the trend of zoological modern dances, theoretically requires the same type of rotation system. This excludes talking to the partner by staring into each other’s eyes with otherwise the risk of ending in so inglorious manner in a typical vestibular vertigo accompanied by nystagmus. That is characterised by saccadic eye movements induced by the vestibule. On a train trip, watching the traveller sitting in front of you that sees the passing scenery allows to observe a form of physiological nystagmus with a quick recall of the eyeballs not perceived by the subject. Therefore, when looking at scrolling objects, it is not possible for the eyes to follow them by going out of their orbital cavities. Aircraft pilots who do aerobatics also know that it is important to always keep the head within the direction of the velocity vector in order to avoid dizziness which may have catastrophic consequences. Nystagmography and vestibulometry are techniques that have made significant progresses, bringing to clinicians valuable data used also in posturology as we shall see later.
2.3.2 The Craniocervical Junction
The strong functional connection between visual exploration and cervical spine dynamics fully justify at the junction of the head and cervical spine a joint system with two degrees of freedom corresponding to the two axes (vertical and horizontal) of visual exploration [16, 18]. This system is a cardan joint, from the name of the philosopher and scientist Gerolamo Cardano who invented the method of fixing the compass on the boat. Indeed it has a vertical pivot on which is placed the needle indicating north. Therefore, the compass must always remain horizontal despite oscillations in roll and pitch of the boat. It is therefore necessary to increase the inertia of the compass by using oil and fix it to the boat by a system with two perpendicular axes of rotation. A combination of both axes may also result in a third DF with an instantaneous axis of rotation. This is exactly what you can see on the craniocervical cardan. It is needed in the future not to talk anymore for the craniocervical junction about a hinge which is a one degree of freedom joint (Figs. 2.8, 2.9 and 2.10).



Fig. 2.9
Drawings of the occipito-cervical cardan. (a) Posterior view of a coronal section: 1. Clivus; 2. Transsected membrana tectoria; 3. Cruciform ligament with the superior longitudinal band fixing the transverse ligament to the occipital and to C2 by the inferior longitudinal band; 4. Articular surface of the dens of C2 and transverse ligament of C1; 5. Alar ligament; 6. Articular capsule of the atlanto-occipital joint; 7. Membrana tectoria. (b) Sagittal section: 1. Clivus; 2. Membrana tectoria in continuity with the posterior longitudinal ligament; 3. Anterior atlanto-occipital membrane in continuity with the anterior longitudinal ligament; 4. Cruciform ligament; 5. Posterior atlantoaxial membrane; 6. Anterior longitudinal ligament; 7. Yellow ligament; 8. Posterior longitudinal ligament

Fig. 2.10
Muscles of the cranio-cervical cardan. (a) Posterior view of the left side: 1. Small posterior rectus; 2. Superior oblique muscle; 3. Great posterior rectus; 4. Inferior oblique; 5. Great occipital nerve (Arnold); 6. Spinal process of C2; 7. Semispinalis cervicis muscle. (b) Coronal section: 1. Splenius capitis; 2. SCM; 3. Levator scapulae; 4. Semispinalis cervicis; 5. Spinal process of C2; 6. Inferior oblique muscle; 7. Great posterior rectus. (c) Scanner in coronal section (the “butterfly of the neck”): 1. Great posterior rectus; 2. Spinal process of C2; 3. Semispinalis cervicis
2.3.2.1 Firstly, a Transverse Axis
Placed between the occipital condyles and the upper concave portion of the lateral masses of the atlas; it allows flexion/extension of the head in the order of 40° and corresponds to the vertical component of the visual exploration.
2.3.2.2 Secondly, a Vertical Axis
Placed between the odontoid process of the axis and the posterior part of the anterior arch of the atlas; it allows horizontal rotation of the head for about 30° corresponding to the horizontal component of the visual exploration. This micrometric system is operated by short posterior muscles: small and great recti muscles, superior and inferior oblique muscles.
For a larger amplitude of horizontal head rotation, a special muscle couple associates the sternocleidomastoid (SCM) on the one side and the splenius capitis on the other side (typical heterolateral synergy) (Fig. 2.11). These two powerful muscles cannot obviously be fixed horizontally in front of the head, which would give them a more high rotating efficiency. They therefore have an oblique direction and are fixed the more laterally possible on the skull and the more medially possible to their bottom: thoracic inlet (clavicle and sternum) to the SCM and spinous processes for the splenius. This gives them a maximum torque, but imposes a spinal compression component that can explain even for small heads the frequency of cervical arthrosis (Fig. 2.12).



Fig. 2.11
Axial sections of cervical spine. (a) C2 axial section: 1. Sterno-cleido-mastoid muscle (SCM); 2. Splenius cervicis; 3. Splenius capitis; 4. Semispinalis muscle; 5. Oblique inferior muscle; 6. Rhinopharynx; 7. Hyoglossal muscle; 8. Submandibular gland; 9. Digastric muscle; 10. Parotid gland. (b) C3 axial section: 1. Anterior belly of digastric muscle; 2. Submandibular gland; 3. Carotid arteries and internal jugular vein; 4. Longus capitis muscle; 5. SCM muscle. (c) C4 axial section: 1. Thyroid cartilage; 2. Interarytenoid pad; 3. Piriformis sinus; 4. Constrictors of pharynx; 5. Carotid artery and internal jugular vein; 6. SCM; 7. Splenius capitis; 8. Trapezius muscle. (d) C4/C5 axial section: 1. Thyrohyoid and sternohyoid muscles; 2. Vocal cord and ligament; 3. Muscular process of arytenoid cartilage; 4. Anterior scalene muscle; 5. Median and posterior scalene muscles; 6. Levator scapulae. (e). C7 axial section: 1. Infrahyoid muscles; 2. SCM; 3. External jugular vein; 4. Internal jugular vein; 5. Left common carotid artery; 6. Thyroid gland; 7. Trachea; 8. Oesophagus; 9. Anterior scalene muscle; 10. Brachial plexus; 11. Middle scalene muscle; 12. Posterior scalene muscle; 13. Levator scapulae

Fig. 2.12
Different types of cervical arthrosis. (a) Arthrosis of the vertebral body. (b) Arthrosis of the vertebral body and the right posterior articular process. (c) Arthrosis of both posterior articular processes
An interesting technical detail can be noted: the splenius cervicis, fixed on the transverse processes of C1 and C2, shrinks at the same time as the head rotation couple. It significantly reduces the difference of torque forces between the powerful head rotation couple and the weak horizontal rotation of the atlas by the inferior oblique muscle. Therefore, it is a protection for the cardan junction in case of sudden head movements.
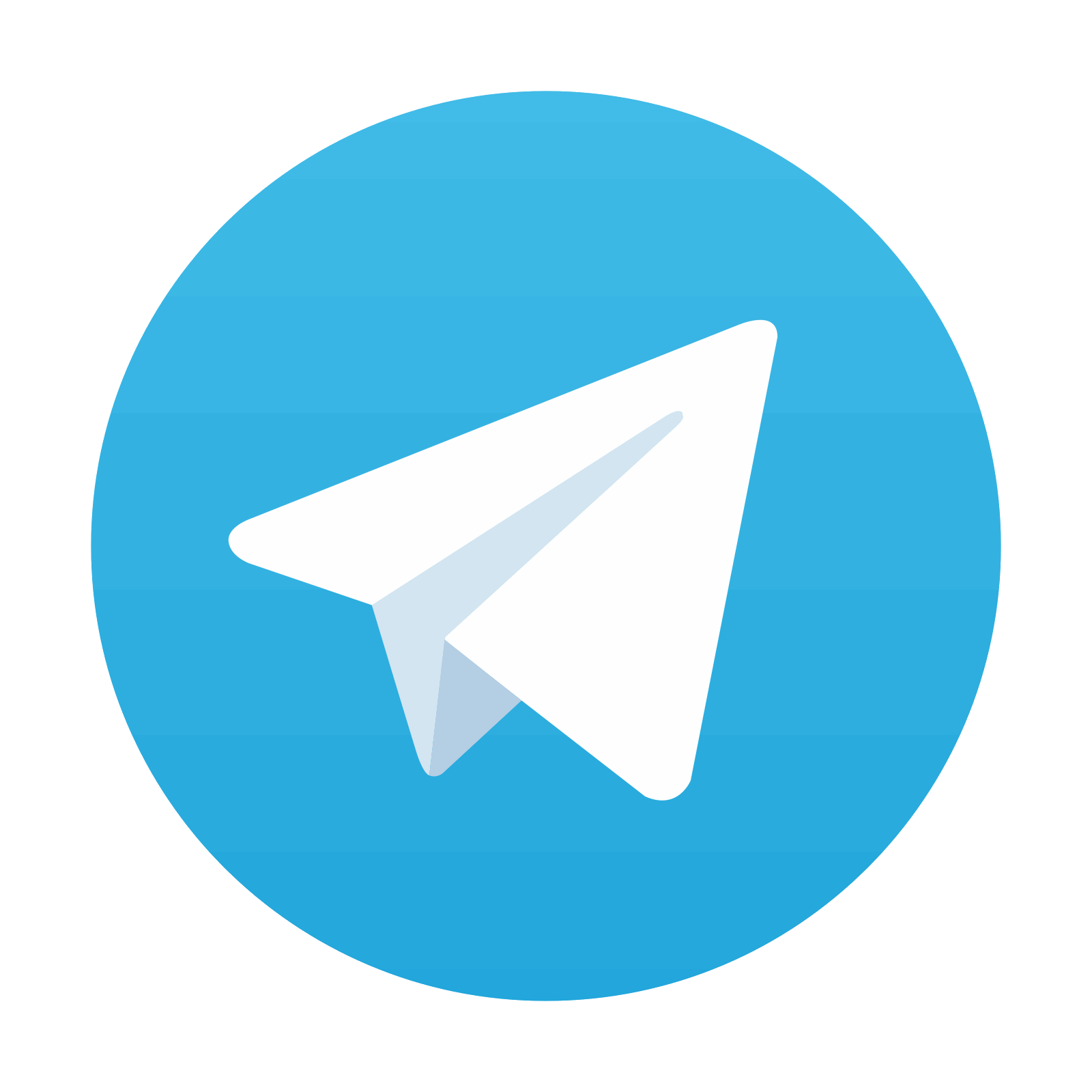
Stay updated, free articles. Join our Telegram channel

Full access? Get Clinical Tree
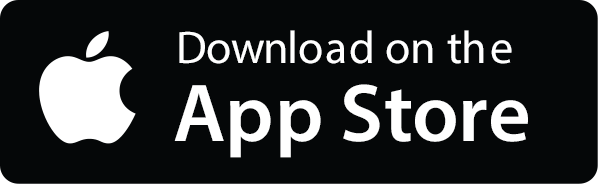
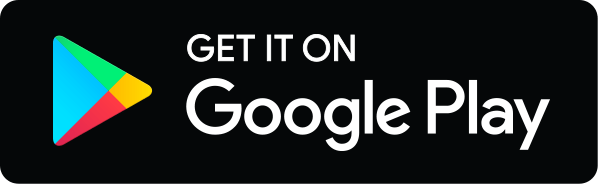
