http://evolve.elsevier.com/Edmunds/NP/
The dramatic decrease in infant mortality rates throughout the twentieth century has paralleled the development of improvements in the prevention, diagnosis, and treatment of pediatric problems; the introduction of better technology; and the increasingly effective use of pharmacologic products. Because children are subject to many of the same diseases as adults, they sometimes are treated with the same drugs and biologic products as adults. However, use of these drugs to treat children is rarely based on evidence-based medicine.
The preparation of valid and generalizable knowledge about drug use in children has been problematic at best. Few clinicians want to experiment with children, and few parents would knowingly allow their infants or small children to participate in research studies that would entail risk of any kind. As a result, many drugs are currently labeled with the comment “Safety and efficacy not established for children.” Many of the initial data about drug use in children were based on firsthand observations by clinicians who tried drugs even when no guidelines were available to assist them. What these clinicians quickly determined was that children are not just small adults who require smaller doses. Health care providers who do not learn that lesson are likely to create problems for both themselves and their patients (Kleigman et al, 2011).
What has been determined is that one third of drugs tested separately in children under the FDA Pediatric Exclusivity Program had different effects than they did in adults; some were more toxic, and others were completely ineffective (Li et al, 2007). Seventy-five percent of drugs used in children have never been tested in this population, yet they are used in children every day. Children are our therapeutic orphans (Li et al, 2007).
Pharmacokinetics of Drug Therapy in Infants and Children
Determining how to give medications to children involves having an especially good understanding of the various physiologic factors that may be affected by these drugs. The growing but immature child is a dynamic and changing entity whose unique characteristics must be understood if errors are to be avoided. The practitioner must remember that information must be mastered for each of the age groups that comprise the pediatric category: preterm infants (gestational age younger than 36 weeks), neonates (30 days old and younger), infants (1 to 12 months), toddlers (1 to 4 years), children (5 to 12 years), and adolescents (13 years and older). Fundamental to this understanding is knowledge of the unique pharmacokinetic variables that may be encountered in pediatric dosing for each of these groups. In recognizing the maturational and developmental differences among children of the same age, developmental differences are further compounded by the same qualities that affect therapeutic response in adults, such as disease, environment, and genetic traits. Consequently, when compared with adults, a wider range of responses to a particular drug treatment can be expected in a population of children; in fact, the same child’s response to a regimen can change rapidly during a single treatment course.
Experts generally agree that devising a therapeutic regimen is most difficult for the youngest patients, particularly neonates. Signs of efficacy or toxicity are difficult to recognize, and newborns are the least able to withstand adverse effects. Neonates often experience the greatest number of therapeutic errors (Kleigman et al, 2011).
Drug Absorption
Many expect drug absorption in infants and children to follow the same basic principles as in adults. However, three factors tend to be especially important in children. First, the physiologic status of the infant or child determines blood flow at the site of parenteral drug administration. Factors that may reduce blood flow to muscular or subcutaneous tissues include cardiovascular shock, vasoconstriction caused by sympathomimetic agents, and heart failure. Under these conditions, absorption of any medications injected into intramuscular or subcutaneous tissue would be reduced. In premature infants with little muscle mass, perfusion to these areas and the resulting absorption are extremely irregular. In larger children, more rapid absorption occurs from the deltoid muscle than from the vastus lateralis muscle, whereas medications are absorbed the slowest from the gluteal muscles (AAP, 1997). Peripheral vasomotor instability, thermal instability, and reduced muscular contractions in premature infants compared with other children and adults also influence drug absorption from intramuscular sites. Toxic drug concentrations may be provoked if perfusion to intramuscular or subcutaneous tissues suddenly increases, promoting greater absorption of medication and increasing the amount of drug that enters the circulation. Drugs that have narrow therapeutic margins, such as anticonvulsants, cardiac glycosides, or aminoglycoside antibiotics, are particularly good examples of drugs with which toxicity may be encountered when absorption is variable (Burns et al, 2013).
Second, for neonates, another factor in absorption is the changing status of GI function. In the first weeks of life, the intestine is highly permeable and premature babies may absorb substances that cannot penetrate the more mature intestine. The presence of amniotic fluid in the stomach ensures an alkaline pH during the days after birth. (Although neonates quickly develop an acidic gastric environment, they do not produce normal levels of gastric acid until somewhere between the ages of 3 and 7 years.) During the neonatal period, the absorption of orally administered members of the penicillin family is enhanced, as is the absorption of carbamazepine suspension, diazepam, and digoxin. Both increased production of gastric acid and slow or varying peristalsis through the GI tract create situations in which orally administered drugs may be partially or totally inactivated by the low pH of gastric contents. Acid-labile drugs, such as ampicillin, nafcillin, and penicillin, develop higher serum concentrations in the presence of higher gastric acid, whereas lower serum concentrations may be found with phenobarbital, phenytoin, and acetaminophen (Kleigman et al, 2011). Drug absorption may be delayed or increased to a greater extent than anticipated because of the absence of intestinal flora, reduced enzyme function, delays in gastric emptying, deficient transport mechanisms across neonatal intestinal membranes, or slow GI transit. The stomach begins to empty more quickly once the infant is 6 to 8 months old. At that time, GI transit may become faster and more unpredictable. Low concentrations of bile acids and lipase may decrease the absorption of lipid-soluble drugs. The activity of pancreatic enzymes is decreased in neonates and infants up to 4 months of age (Koren & Cohen, 2006). These factors are particularly important to remember when one is contemplating the administration of a sustained-release agent. If the drug passes through the GI tract rapidly, absorption will be insufficient and a subtherapeutic dose will be delivered. Rectal suppositories generally should be avoided for drug administration, primarily because children may not retain the dosage form long enough to receive the entire dose.
Because so little research has been conducted on oral vs. intravenous administration, differences in the bioavailability of drugs through different routes of administration in premature infants are poorly understood. If a drug is poorly absorbed from the GI tract of an adult, absorption may be much more efficient in a premature child because of the delayed emptying. The one common finding of most studies is that drug absorption varies widely; the younger the child, the more erratic the absorption may be (Burns et al, 2013).
Third, the skin of premature and newborn infants has a greater ability to absorb some chemicals because of increased hydration and increased permeability, resulting from underdevelopment of the stratum corneum in the epidermal barrier. The transdermal route may be used therapeutically in selected infants to reduce the unpredictability of some oral and intramuscularly administered medications, such as theophylline. However, commercially available transdermal dosage forms are not intended for pediatric patients and would deliver doses much higher than those needed for infants and children. Rubbing the drug into the skin, incorporating the drug in an oily vehicle, and using an occlusive dressing, sometimes by wrapping the site in plastic wrap, may increase cutaneous absorption of topical products (Edmunds, 2013). Children have the potential for increased absorption through the skin because their skin is thinner and more sensitive—thus allowing for greater penetration—particularly if the skin is damaged or an occlusive dressing (even a diaper) is applied over the medication. Factors that increase transdermal drug absorption also increase the risk to the infant of toxic effects following topical use of drugs, as well as such items as hexachlorophene soaps, boric acid, powders, or rubbing alcohol (Gilman, 1990). Both clinicians and parents should remember that small children can ingest a topical drug by sucking or licking it off an accessible patch of skin.
Drug Distribution
Drug distribution as a process is determined by two factors: (1) the physicochemical properties of the drug itself (i.e., the molecular weight, etc.), which do not vary, and (2) the physiologic factors specific for the patient, including total body water, extracellular water, protein binding, and pathologic conditions that modify physiologic function, all of which vary widely in different patient populations (McCance & Huether, 2009). The distribution volumes of drugs vary in the child as the body composition changes through growth and development. The neonate has a higher proportion of its body weight in the form of water than does the adult, who has 50% to 60% of body weight in water. Small premature neonates may have 85% of their body weight as water, with 40% of that amount as extracellular water, whereas the normal full-term neonate usually has only about 70% body weight as water. Because many drugs are distributed throughout the extracellular water space, the volume or size of the extracellular water compartment may be important in determining the concentration of drug at the receptor sites. This is an especially important consideration because many neonates diurese heavily in the first 24 to 48 hours of life, and many important drugs are water soluble (Koren & Cohen, 2006; McCance & Huether, 2009). Water-soluble drugs, such as the aminoglycoside antibiotic gentamicin, will be distributed more widely in the body, resulting in lower serum levels. To compensate, a higher dosage is necessary.
Another variable that affects drug distribution in premature infants is a reduced percentage of body fat. Premature infants may have 1% of body weight in fat compared with 15% in normal full-term infants (Yaffe & Aranda, 2010). Organs that ordinarily would accumulate high concentrations of lipid-soluble drugs in adults and older children may not accumulate as much in infants. Early on, when the proportion of body fat is small, lipid-soluble drugs such as vitamins A and E do not distribute as well, provoking higher drug concentrations in the serum. In such cases, the dosage may have to be reduced to avoid toxicity. In older children, the alterations in body fat associated with obesity and extreme emaciation obviously affect fat storage of drugs just as they do in adults.
In addition to fat, selected drugs are stored to a smaller extent in other tissues. For example, the tetracyclines chelate with calcium and so have a high affinity for the rapidly growing teeth and bones of infants and young children (Kleigman et al, 2011).
Drugs are not distributed uniformly throughout the body. For example, drug distribution to the central nervous system is small, and movement through the brain’s rich blood supply is unique. Drugs must move from the bloodstream across the blood-brain barrier. Because certain drugs, such as highly lipid-soluble drugs (e.g., tetracycline, diazepam), readily penetrate the central nervous system, there is no absolute barrier. However, the blood-brain barrier is encountered when drugs that are moving from the blood across the capillary endothelium are restricted by the close approximation of the glial cells with the capillary endothelium. Also, drug access to the cerebrospinal fluid is limited by the epithelium of the choroid plexus (Kleigman et al, 2011). In premature infants, incomplete glial development enhances permeability of the blood-brain barrier and permits drugs and bilirubin to enter the central nervous system more readily. Poorly lipid-soluble drugs such as gentamicin and penicillin may then cross the blood-brain barrier when administered in large doses, when administered via rapid intravenous infusion, or when administered to neonates with renal failure. The permeability of the blood-brain barrier in infants and children of all ages is increased in meningitis, brain tumors, and cranial trauma. In these conditions, drugs that do not ordinarily enter the central nervous system may do so without difficulty (Yaffe & Aranda, 2010).
Some drugs are reversibly bound to plasma proteins (usually albumin), fat, bone, or other tissues. Diazepam, digoxin, furosemide, and warfarin are examples of drugs that are highly bound to plasma albumin. When bound, these tissue-bound drugs are not free to gain access to receptor sites and thus are pharmacologically inactive. Some tissue-bound drugs are released when plasma concentrations fall; thus, these tissues are said to act as drug storage depots (Katzung, Masters, & Trevor, 2009; McCance & Huether, 2009).
Plasma protein binding in neonates is comparatively low because of decreased plasma protein concentration, lower binding capacity of protein, decreased affinity of proteins for drug binding, and competition for certain binding sites by endogenous compounds. Because of this, the concentration of free drug in plasma is increased and exerts powerful pharmacologic effects that can result in greater drug effect or toxicity. Acidosis, cold stress, and hypoglycemia in the neonate may produce free fatty acids that act to displace drugs from plasma albumin-binding sites (Brunton, Chabner, & Knollman, 2010). However, because drugs bound to plasma proteins cannot be eliminated by the kidneys, an increase in free drug concentration may also increase its clearance (Katzung, Masters, & Trevor, 2009).
In the neonate, bilirubin, maternal hormones, and other endogenous substances also occupy available plasma protein-binding sites. Sulfonamides, furosemide, large doses of vitamin K, and sodium benzoate given to the mother during labor or delivery, or passed to the baby through breastfeeding, may compete with serum bilirubin for binding to albumin. If these drugs are given to a neonate with jaundice, they can displace bilirubin from albumin. Because of the greater permeability of the neonatal blood-brain barrier, substantial amounts of bilirubin then could enter the brain and cause kernicterus. Additionally, as the serum bilirubin rises for physiologic reasons or because of a blood group incompatibility, bilirubin displacement of a drug from albumin can substantially raise the free drug concentration. This might occur without altering the total drug concentration and would result in a greater therapeutic effect or toxicity at normal concentrations (Koren & Cohen, 2006).
The decreased capacity for plasma protein binding of drugs can increase their apparent volumes of distribution. The clinical implications of this action are that to obtain a therapeutic serum concentration of many drugs, premature infants will require a larger loading dose than that required for older children and adults (Brunton, Chabner, & Knollman, 2010).
Finally, certain drugs distributed in the breast milk of breastfeeding mothers may pose problems for infants. The American Academy of Pediatrics (AAP) last updated its warning statement in 2002 to list drugs that should be avoided by the mother during pregnancy and breastfeeding if at all possible and the effects of drugs on infants. Drugs that are absolutely contraindicated during breastfeeding include bromocriptine, cimetidine, clemastine, cyclophosphamide, ergotamine, gold salts, methimazole, phenindione, and thiouracil (AAP, 2002).
Drug Metabolism
The biotransformation of drugs into usable substances in the body involves chemical reactions that convert a drug to an inactive or a less active compound. These metabolic reactions usually produce metabolites that are less lipid soluble and more readily eliminated than are highly lipid-soluble compounds. Not all metabolites are pharmacologically inactive. Active metabolites are excreted unchanged or undergo additional metabolic reactions. Poorly absorbed lipid-soluble drugs, such as the penicillins, are not metabolized but are excreted unchanged (Brunton, Chabner, & Knollman, 2010).
In general, drug metabolism in infants is substantially slower than that in older children and adults. The newborn’s kidneys are immature, and because most drug metabolism takes place in the liver, the fact that the P450-dependent mixed-function oxidases and the conjugating enzymes of infants are only 50% to 70% of adult values is an important consideration in the treatment of children (Katzung, Masters, & Trevor, 2009). Different enzymes are present in varying amounts, but the capacity to increase production of all enzymes continues until the third or fourth year of life. For example, the glucuronidation pathway is undeveloped in infants, but the sulfation pathway is relatively well developed and may compensate for deficits in the other pathway in the presence of some drugs (DiPiro et al, 2011; Rane, 2010).
Given neonates’ decreased ability to metabolize drugs, they may be at increased risk for adverse effects because of slow clearance rates and prolonged half-lives, particularly in drugs given over long periods. One of the earliest cases in which serious adverse effects were observed in neonates following administration of a drug that had not been adequately studied in pediatric patients was the development of “gray baby syndrome” after treatment with the antibiotic chloramphenicol. After 23 deaths in neonates, it was determined that the immature livers of these infants were unable to clear chloramphenicol from the body, allowing toxic doses of the drug to accumulate (DiPiro et al, 2011). These facts make it essential for the clinician to consider the maturation of the infant when evaluating whether to administer drugs metabolized in the liver. Conversely, some drugs that a mother might take would induce the fetal hepatic enzymes to mature early. This would result in faster metabolism of certain drugs, with less therapeutic effect and lower plasma drug concentrations. One of the drugs that might cause this action is phenobarbital (Koren & Cohen, 2006). By the first birthday, the liver’s metabolic capabilities are not only mature but more vigorous than those of an adult, probably because the child’s liver has a greater volume per kilogram of body weight. Although this phenomenon is probably most apparent between the ages of 2 and 6 years, it continues until approximately 10 to 12 years of age so that certain drugs may need to be given in higher dosages or more often. An example of this is theophylline. A child between 1 and 9 years of age with asthma might require markedly higher doses of theophylline on a weight basis compared with an adult (Brunton, Chabner, & Knollman, 2010). Dosing errors because of this have led to decreased use of this drug.
Although most drug metabolic reactions occur in the liver, the GI tract, kidney, and plasma may also biotransform some drugs. The extent of use of these alternate forms of metabolism is usually identified in pharmacokinetic tables, and these forms account for only a small percentage of drug metabolism.
Excretion
As with metabolism, the growth and maturity of the child’s organs have a significant effect on the elimination of drugs. Difficulty in excreting drugs that results from incomplete development of the fetal renal excretion system, including reduction in glomerular filtration, tubular secretion, and tubular reabsorption, is gradually resolved with increased gestational age but may still be markedly decreased at birth and may only slowly develop to capacity over the first year of life (Kleigman et al, 2011).
For the first few days of life, the glomerular filtration rate (GFR) of a neonate may be at only 30% to 40% of the adult rate. This rate may be even lower in premature babies. As the child grows and matures, the clearance rate improves, even during the first week of life. By the end of the third week of life, GFR is usually 50% to 60% of the adult value and may reach the adult value by 6 months (Brunton, Chabner, & Knollman, 2010).
This developmental process has implications for drug clearance, particularly for common drugs such as penicillin, aminoglycosides, and digoxin, for which rates may fall to 17% to 34% of the adult clearance rate. If a child is ill enough to require these drugs, its GFR may not improve as predicted during the first weeks and months of life. This will necessitate adjustments in dosage and dosing schedules. The child will require more vigilant monitoring, and appropriate dosages should be calculated based on plasma drug concentrations determined at intervals throughout the course of therapy (Koren & Cohen, 2006).
Changes in urinary pH may also affect the rate at which a drug is excreted. Ammonium chloride, ascorbic acid, and other drugs that acidify the urine increase the rate at which pseudoephedrine, meperidine, quinidine, and other weak bases are excreted. Sodium bicarbonate and other drugs that alkalinize the urine increase the rate at which phenobarbital, aspirin, nitrofurantoin, and other weak acids are excreted. Although increasing the rate at which a drug is excreted shortens its effect, decreasing urinary excretion increases the drug’s duration of action (Yaffe & Aranda, 2010).
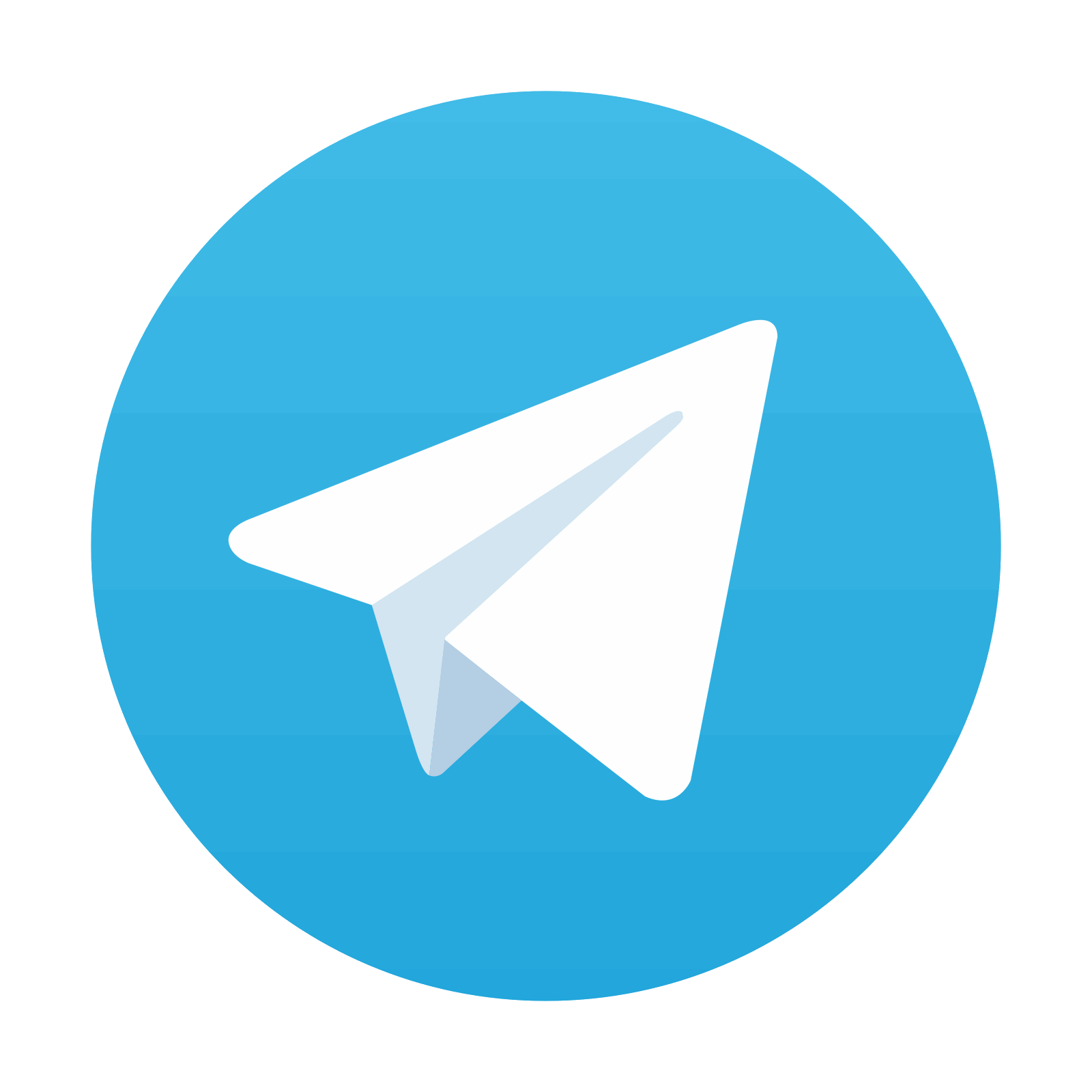
Stay updated, free articles. Join our Telegram channel

Full access? Get Clinical Tree
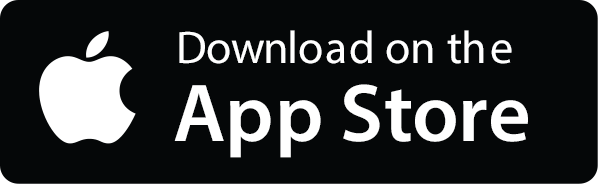
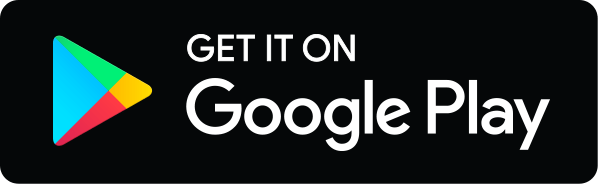