Solid-Organ Transplantation
KEY CONCEPTS
A combination of two to four immunosuppressive drugs is used to target different levels of the immune cascade to prevent allograft rejection and allow lower doses of the individual agents to minimize their toxicity.
Calcineurin inhibitors (CNIs), such as cyclosporine and tacrolimus, which inhibit interleukin (IL)-2 and thus block T-cell activation are the backbone of immunosuppressive regimens. However, they are associated with significant adverse effects, namely, nephrotoxicity and neurotoxicity.
CNI-induced nephrotoxicity is one of the most common side effects observed in transplant recipients and is the leading cause of renal dysfunction in nonrenal transplant patients. Therapeutic drug monitoring is used in an attempt to optimize the use of CNIs.
Corticosteroids are a key component of immunosuppressive regimens because they block the initial steps in allograft rejection. However, the adverse effects associated with their long-term use have prompted the investigation of corticosteroid-free immunosuppressive protocols. Corticosteroids remain the cornerstone of the treatment of allograft rejection.
Antimetabolites agents such as azathioprine and mycophenolate inhibit T-cell proliferation by altering purine synthesis to prevent acute rejection. Bone marrow suppression is the most significant adverse effect associated with these agents.
The proliferation signal inhibitors (PSI) sirolimus and everolimus exert their activity by inhibiting the mammalian target of rapamycin (mTOR) receptor, which alters T-cell response to IL-2. The adverse effects associated with sirolimus include thrombocytopenia, anemia, and hyperlipidemia.
Antibody preparations that target specific receptors on T cells are classified as depleting or nondepleting. Most lymphocyte-depleting antibodies are associated with significant infusion-related reactions.
Long-term allograft and patient survival is limited by chronic rejection, cardiovascular disease, and long-term immunosuppressive complications such as malignancy.
INTRODUCTION
Solid-organ transplantation provides a lifesaving treatment for patients with end-stage cardiac, kidney, liver, lung, and intestinal disease. Over 300 U.S. hospitals offer transplant services, and pharmacists are often an integral part of the transplant team. The Centers for Medicare and Medicaid Services regulations require that transplant programs have a multidisciplinary team including individuals with experience in pharmacology. While the regulations do not specifically state that each center must have a pharmacist, a pharmacist would certainly provide the desired expertise in transplant pharmacotherapy that the regulations mandate.1
With the success of transplantation, an increasing number of transplant recipients are in our communities. By the end of 2009 there were nearly 200,000 people living with a solid-organ transplant in the United States, which has doubled over the previous decade.1 In 2011, 28,537 solid-organ transplants were performed. Kidney transplants remain the most common; 11,043 were from cadaveric donors and 5,770 from living donors. The next most frequently transplanted organ was the liver, with 6,095 from cadaveric donors and 247 from living donors. Heart and pancreas (or combined kidney–pancreas) transplants account for over 2,300 and almost 1,100 transplants, respectively; over 1,800 lung transplants were performed as well.1 While the demand for transplantation continues to grow, the number of cadaveric donors has remained relatively stable during the last decade. In 2012, more than 115,000 persons in the United States were waiting for a transplant (over 93,000 people were awaiting a kidney, 16,000 a liver, and 3,000 were on the list for a heart transplant). Median waiting time for a cadaveric kidney is more than 3.5 years. For liver transplantation the median time to transplant is about 1 year, whereas for heart transplantation it is approximately 6 months. For heart, liver, and lung transplantation clinical status is an important factor affecting waiting times, with the sickest patients receiving priority for available organs.1
To increase the number of organs available for transplantation, several strategies have been employed in the last several years. The use of living donors for renal transplantation represents over one third of all kidney transplants, more than any other organ. Living-donor transplantation is also becoming increasingly important for those with end-stage liver and lung disease. Efforts to expand the cadaveric donor pool have included relaxation of age restrictions, development of better preservation solutions, use of “extended-criteria” and non–heart-beating donors, and, in the case of liver transplants, the transplantation of one liver to more than one recipient or implantation of only a segment of a liver. Although very controversial, others advocate the creation of a regulated system for compensating individuals in a monetary fashion for the “donation” of a kidney.
Clinical Controversy…
Despite all these efforts, patients continue to die awaiting transplantation. In 2009, more than 7,000 people who were on transplantation waiting lists died. In all areas, efforts have been made to improve organ allocation by moving toward allocation based primarily on “medical necessity” versus time on the waiting list. Although dialysis can be used for an extended period of time to partially replace the function of the kidneys, such options are not readily available for most liver and heart transplantation candidates. Left ventricular assist devices are now used commonly as a bridge to transplantation for many heart transplantation candidates; however, hepatocyte transplantation and artificial liver support remain investigational alternatives or bridges to liver transplantation.2
Patient and graft survival rates following transplantation have improved significantly over the past 30 years as a result of advances in pharmacotherapy, surgical techniques, organ preservation, and the postoperative management of patients (Table 70-1). The half-life of transplanted kidneys has also continued to improve, but is lower for kidneys from deceased donors compared to living donors, 14.7 years and 26.6 years, respectively. Similarly, the half-life of the transplanted livers and hearts has improved to 10 years for livers from deceased donors and 14.9 years for hearts.1 In this chapter, the epidemiology of end-stage kidney, liver, and heart disease is briefly reviewed, the pathophysiology of organ rejection is reviewed, the pharmacotherapeutic options for individualized immunosuppressive regimens are critiqued, and the unique complications of these regimens along with the therapeutic challenges they present are discussed.
EPIDEMIOLOGY AND ETIOLOGY
The epidemiology and etiology associated with solid-organ transplant is specific to the type of organ transplant.
Kidney
Renal transplantation is the preferred long-term therapeutic option for most patients with end-stage renal disease (ESRD) because it provides patients with the greatest potential improvement in quality of life. Dialysis catheter-related infections, peritoneal dialysis-associated peritonitis, and scheduled dialysis treatments are avoided, and dietary restrictions are fewer. Patients who receive a renal transplant before the initiation of dialysis have markedly improved quality of life and prolonged life expectancy.3 The use of living-donor transplantation has made this increasingly possible. Although the analysis of quality of life is complex, patients generally report improved quality of life following transplantation as compared with patients on maintenance dialysis.4
Diabetes mellitus, hypertension, and glomerulonephritis are the three leading causes of ESRD leading to kidney transplantation and account for more than 70% of patients (see Chap. 29).5 Patients with medical conditions such as unstable cardiac disease or recently diagnosed malignancy, for whom the risk of surgery or chronic immunosuppression would be greater than the risks associated with chronic dialysis, are generally excluded from consideration for transplantation.
Liver
Noncholestatic cirrhosis (hepatitis C, alcoholic cirrhosis, hepatitis B, nonalcoholic steatohepatitis, and autoimmune hepatitis) is the primary cause of end-stage liver disease and more than 70% of liver transplant recipients have been diagnosed with one of these conditions.1 Livers are allocated based on a United Network for Organ Sharing-adapted, Model for End-stage Liver Disease (MELD) score.6 This score, based on serum creatinine concentration, total serum bilirubin concentration, international normalized ratio, and etiology of cirrhosis, has been demonstrated to be a useful tool to predict impending mortality.
The few absolute contraindications to liver transplantation are active alcohol or substance abuse. Although hepatitis B and C can recur in the transplanted liver, these are not absolute contraindications to liver transplantation.2,7
Heart
Cardiac transplant candidates are typically patients with end-stage heart failure who have New York Heart Association class III or IV symptoms despite maximal medical management and have an expected 1-year mortality risk of 50% or greater without a transplant.8 Idiopathic cardiomyopathy and ischemic heart disease account for heart failure in more than 90% of heart transplantation recipients.1 Other etiologies include valvular disease, retransplantation for graft atherosclerosis or dysfunction, and congenital heart disease. The role of heart transplantation as a therapeutic option for patients with heart failure is discussed in Chapter 4.
Absolute contraindications to orthotopic cardiac transplantation include the presence of an active infection (except in the case of an infected ventricular assist device, which is an indication for urgent transplantation) or the presence of other diseases (e.g., malignancy) that may limit survival and/or rehabilitation and severe, irreversible pulmonary hypertension.
PHYSIOLOGIC CONSEQUENCES OF TRANSPLANTATION
Transplantation is truly lifesaving for heart, liver, and lung transplantation recipients, whereas renal transplantation is associated with improved quality of life and survival when compared with dialysis.9 Most heart transplantation patients return to New York Heart Association functional class I following transplantation. Although not all return to work, 89.9% of patients consider themselves to have no activity limitations at 1-year follow-up.10 The specific physiologic consequences of kidney, liver, and heart transplantation are discussed below.
Kidney Transplantation
The glomerular filtration rate (GFR) of a successfully transplanted kidney may be near normal almost immediately after transplantation. In some patients, however, the concentration of standard biochemical indicators of renal function, such as serum creatinine and blood urea nitrogen, may remain elevated for several days. Standard formulas used to predict drug dosing rely on a stable serum creatinine and may be inaccurate immediately following transplantation (see eChap. 18 and Chap. 33).
Although the allograft is able to remove uremic toxins from the body, it may take several weeks for other physiologic complications of ESRD, such as anemia, calcium and phosphate imbalance, and altered lipid profiles, to resolve. The renal production of erythropoietin and 1-hydroxylation of vitamin D may return toward normal early in the postoperative period. Because the onset of physiologic effects may be delayed, continuation of the patient’s pretransplantation vitamin D, calcium supplementation, and/or phosphate binders may be warranted. The duration of therapy will depend on how rapidly kidney function improves. Patients should be monitored for hypophosphatemia and hypercalcemia for the first few days to weeks after kidney transplantation.
Primary nonfunction of a renal allograft or delayed graft function (DGF) is characterized by the need for dialysis in the first postop week or the failure of the serum creatinine to fall by 30% of the pretransplantation value. The incidence of DGF in cadaveric renal transplantation ranges from 8% to 50% and results in a slower return of the kidney’s excretory, metabolic, and synthetic functions. DGF is associated with prolonged hospital stays, higher costs, difficult management of immunosuppressive therapy, slower patient rehabilitation, and poor graft survival. Other early causes of renal dysfunction such as urethral obstruction or arterial or venous stenosis or thrombosis should be distinguished from DGF.
The primary cause of DGF is acute tubular necrosis (ATN). The incidence of ATN is higher when kidneys are harvested from donors who recently experienced a cardiac arrest, those who were hypotensive or on vasopressors, or older donors (age >55 years). While cyclosporine and tacrolimus have been implicated in the prolongation of ATN, a clear cause-and-effect relationship has not been established. Nonetheless, most clinicians will decrease calcineurin inhibitor (CNI) doses in patients with ATN. DGF predisposes patients to acute rejection, possibly as a consequence of decreased CNI levels and a resultant reduction in the level of immunosuppression.
Clinical Controversy…
Liver Transplantation
The physiologic consequences of liver transplantation are complex, involving changes in both metabolic and synthetic function. Postoperatively, the liver transplant recipient will likely have many fluid, electrolyte, and nutritional abnormalities. Biliary tract dysfunction may alter the absorption of fats and fat-soluble drugs.11 Poor absorption of the lipid-soluble drug cyclosporine improves after successful liver transplantation and reestablishment of bile flow. Vitamin E deficiency and its neurologic complications in liver failure patients are reversed after successful liver transplantation. In stable adult liver transplant patients, the concentrations of retinol and tocopherol are similar to those seen in normal healthy subjects, indicating recovery of transplanted liver production and excretion of bile salts needed for fat-soluble vitamin absorption. Table 70-2 summarizes the effects of liver transplantation on metabolism and renal elimination that are seen in the immediate postoperative period. Most of these changes resolve as liver function normalizes.
TABLE 70-2 Perioperative Changes in Drug Disposition and Elimination Following Liver Transplantation
The newly transplanted liver fails to function in 10% to 15% of recipients as the result of several different mechanisms. Early graft failure can result from preexisting disease in the donor, and even coagulation defects have been acquired through donor organs. The technical complexity of the operation can produce flaws in revascularization that also lead to graft nonfunction. Surgical complications include portal vein or hepatic artery thrombosis and bile duct leaks. Ischemic injury can also result in early graft dysfunction. While hyperacute rejection in liver transplantation rarely occurs, graft failure in the first two postoperative weeks may indicate antibody-mediated graft destruction.
Heart Transplantation
The orthotopically transplanted heart is denervated and no longer responds to physiologic stimuli and pharmacologic agents in a normal manner (Table 70-3).9 In situations requiring an increased heart rate such as exercise or hypotension, the denervated heart is unable to acutely increase heart rate but instead relies on increasing the stroke volume. Later in the course of exercise or hypotension, heart rate increases in response to circulating catecholamines. While the maximum exercise capacity of heart transplant recipients is below normal, most patients are able to resume normal lifestyles and reasonably vigorous activity levels. Partial reinnervation may occur over time, thereby facilitating more normal physiologic and pharmacologic responses and better exercise capacity.10
TABLE 70-3 Altered Responses to Cardiac Drugs in the Denervated Transplanted Heart
A number of autoregulatory, anatomic, and physiologic responses present in the normal heart are interrupted or blunted for the first 6 weeks after transplantation. The donor sinus node function may be impaired by preservation injury, direct surgical trauma at excision, the presence of long-acting antiarrhythmics (e.g., amiodarone) taken prior to transplant by the recipient, and a lack of “conditioning” responsiveness to catecholamines.10 Consequently, the transplanted heart generally requires chronotropic support with either milrinone or pacing in the perioperative period to maintain a heart rate of 90 to 110 beats per minute and satisfactory hemodynamics (i.e., blood pressure, urine output, and tissue perfusion). Approximately 10% to 20% of transplant patients will have persistent chronotropic incompetence requiring either short courses of medications, such as terbutaline or theophylline, or permanent cardiac pacing.
Right ventricular function is frequently impaired, presumably as a result of preservation injury and elevated pulmonary vascular resistance. A “restrictive” hemodynamic pattern may be present initially but usually improves in 6 weeks following transplantation. Donor–recipient size mismatch may contribute to early posttransplantation hemodynamic abnormalities characterized by higher right and left ventricular end-diastolic pressures. Supraventricular arrhythmias are usually transient and may result from over vigorous use of catecholamines or milrinone. If this type of arrhythmia occurs after the perioperative period, the astute clinician should consider the possibility of acute rejection.
Myocardial depression frequently occurs and generally requires inotropic support with agents such as dobutamine, milrinone, and epinephrine. On occasion, intra- or postoperative administration of vasodilators, including nitric oxide, and inotropic agents may be necessary to treat right-sided failure in the transplant patient; milrinone and isoproterenol are preferred in this setting.
Persistent abnormalities of diastolic function are often noted in the transplanted heart such that intracardiac pressures increase in an exaggerated fashion in response to exercise and/or volume infusion.10 These abnormalities are due in part to denervation, but also to acute rejection or to the scarring secondary to previously treated rejection episodes, hypertension, or cardiac allograft vasculopathy.
Hypertension may occur following surgery secondary to the effect of elevated catecholamine levels and systemic vascular resistance as the residual effects of end-stage heart failure on the healthy heart. Systolic blood pressure should be maintained at 110 to 120 mm Hg to enhance cardiac function. In the acute posttransplantation period, IV nitroprusside or nitroglycerin may be needed, whereas oral angiotensin-converting enzyme inhibitors (ACEIs) and/or amlodipine are commonly used once the patient can ingest oral medications.
PATHOPHYSIOLOGY OF REJECTION
Rejection of the transplanted tissue can take place at any time following surgery and is classified clinically as hyperacute rejection, acute cellular rejection (ACR), humoral rejection, and chronic rejection.
General Concepts
Rejection of any transplanted organ is primarily mediated by activation of alloreactive T cells and antigen-presenting cells such as B lymphocytes, macrophages, and dendritic cells. Acute allograft rejection is caused primarily by the infiltration of T cells into the allograft, which triggers inflammatory and cytotoxic effects on the graft. Complex interactions between the allograft and cellular cytokines, cell-to-cell interactions, CD4+ and CD8+ T cells, and B cells ultimately lead to chronic rejection and graft loss if adequate immunosuppression is not maintained.12
The sequence of events that underlies graft rejection is recognition, via major histocompatibility complex (MHC) class I and II antigens, of the donor’s histocompatibility differences by the recipient’s immune system, recruitment of activated lymphocytes, initiation of immune effector mechanisms, and finally graft destruction. The specifics of this immune cascade of organ rejection are discussed in eChapter 20. The complex nature of cytokine interactions makes it very difficult to design drugs with exclusive actions (Fig. 70-1).
FIGURE 70-1 Stages of CD4 T-cell activation and cytokine production with identification of the sites of action of different immunosuppressive agents. Antigen major histocompatibility complex (MHC) II molecule complexes are responsible for initiating the activation of CD4 T cells. These MHC–peptide complexes are recognized by the T-cell recognition complex (TCR). A costimulatory signal initiates signal transduction with activation of second messengers, one of which is calcineurin. Calcineurin removes phosphates from the nuclear factors (NFAT-P) allowing them to enter the nucleus. These nuclear factors specifically bind to an interleukin (IL)-2 promoter gene facilitating IL-2 gene transcription. Interaction of IL-2 with the IL-2 receptor (IL-2R) on the cell membrane surface induces cell proliferation and production of cytokines specific to the T cell. (APC, antigen-presenting cells; MMF, mycophenolate mofetil.) (Reprinted from Mueller XM. Drug immunosuppressive therapy for adult heart transplantation. Part I. Immune response to allograft and mechanism of action of immunosuppressants. Ann Thorac Surg 2004;77:354–362, Copyright © 2004, with permission from Elsevier.)
Efforts are made to allocate well-matched kidneys, according to human leukocyte antigens (HLA)-A, -B, and -DR, to minimize rejection and enhance survival. However, the benefit of having no recipient donor mismatches may be negated by excessive cold ischemia time (>36 hours) and donor age older than 60 years. HLA tissue matching is not performed routinely before transplantation for livers and hearts because organ availability is more limited and the optimal cold ischemia time is shorter. However, if the potential recipient’s blood is reactive against a panel of random donor blood samples (i.e., panel reactive antibody [PRA] >10% to 20%), a negative T-cell crossmatch is required prior to transplantation. Transplanted organs must be matched for ABO blood group compatibility with the recipient as ABO mismatching will result. Liver transplantation may be carried out in emergency situations across ABO blood groups, but survival is lower.
Hyperacute Rejection
Hyperacute rejection may be evident within minutes of the transplantation procedure when preformed donor-specific antibodies are present in the recipient at the time of the transplant. Hyperacute rejection can also be induced by immunoglobulin G antibodies that bind to antigens on the vascular endothelium, such as class I MHC, ABO, and vascular endothelial cell antigens. Tissue damage can be mediated through antibody-dependent, cell-mediated cytotoxicity, or through activation of the complement cascade. The ischemic damage to the microvasculature rapidly results in tissue necrosis.
Hyperacute rejection has become uncommon in kidney and heart transplants. A positive crossmatch presents a serious risk for graft failure even if hyperacute rejection does not occur. A negative lymphocytotoxicity crossmatch does not entirely rule out the possibility of hyperacute rejection because non-MHC antigens on the vascular endothelium can serve as targets of donor-specific antibodies. Early graft dysfunction is treated with supportive care and retransplantation if possible. The reason for the rarity of hyperacute rejection in liver transplantation is not fully understood, but the local release of cytokines may alter the immunologic reaction in the liver.
Acute Cellular Rejection
Acute rejection is most common in the first few months following transplantation but can occur at any time during the life of the allograft. ACR is mediated by alloreactive T-lymphocytes that appear in the circulation and infiltrate the allograft through the vascular endothelium. After the graft is infiltrated by lymphocytes, the cytotoxic cells specifically target and kill the functioning cells in the allograft. At the same time, local release of lymphokines attracts and stimulates macrophages to produce tissue damage through a delayed hypersensitivity-like mechanism. These immunologic and inflammatory events lead to nonspecific signs and symptoms including pain and tenderness over the graft site, fever, and lethargy.
Kidney
Acute rejection, which may affect up to 20% of patients during the first 6 months following transplantation, is evidenced by an abrupt rise in serum creatinine concentration of ≥30% over baseline. A specific histologic diagnosis can be obtained via biopsy of the allograft and is often used to guide therapy for rejection. A biopsy specimen with a diffuse lymphocytic infiltrate is consistent with ACR. After the diagnosis of rejection has been confirmed, the potential risks and benefits of specific antirejection therapies must be evaluated. Hypertension often worsens during an episode of rejection, and edema and weight gain are common as a result of sodium and fluid retention. Symptomatic azotemia may also develop in severe cases.
Liver
The liver is more likely to promote immunologic tolerance than the other vascularized organs. Approximately 18% of liver transplantation recipients will experience a rejection episode in the first posttransplant year. The clinical signs of ACR include leukocytosis and a change in the color or quantity of bile for those who still have an external drainage tube in place. A serum bilirubin 50% over baseline or increases in hepatic transaminases to values more than three times the upper limit of normal are sensitive markers of rejection. Although a liver biopsy provides definitive evidence of the diagnosis of rejection, a prompt response to antirejection medication has also proven useful as a means to differentiate rejection from other causes of hepatic dysfunction.
Heart
Approximately 16% of heart transplantation recipients will experience at least one episode of acute rejection during the first year.13 Because rejection of the cardiac allograft is not necessarily accompanied by overt clinical signs or symptoms and because the incidence of acute rejection is highest during the first year posttransplant, endomyocardial biopsies are often performed at regularly scheduled intervals following transplantation.14 A typical biopsy schedule would be weekly for the first postoperative month, biweekly for the next 2 months, and monthly to bimonthly through the remainder of the first posttransplant year. Nonspecific symptoms, including low-grade fever, malaise, mild reduction in exercise capacity, heart failure, or atrial arrhythmias may also be evident and if present are reflective of a more severe rejection episode.
Antibody-Mediated Rejection
Antibody-mediated rejection (AMR), sometimes referred to as vascular or humoral rejection, is characterized by the presence of antibodies directed against HLA antigens present on the donor vascular endothelium. The antibodies activate complement, which creates a membrane attack complex that directly damages the organ tissue and further attracts inflammatory cells to the allograft. The resultant damage is histologically distinct from cellular rejection that involves microvascular injury, often to the peritubular capillaries.15 Definitive diagnosis of AMR is based on the presence of three criteria: presence of donor-specific antibodies, immunofluorescence staining of C4d deposits in the peritubular capillaries, and evidence of allograft dysfunction.16 Circulating immune complexes often precede humoral rejection. This form of rejection is less common than cellular rejection and generally occurs in the first 3 months after transplantation. It is associated with an increased fatality rate and appears to be more common when antilymphocyte antibodies are used for rejection prophylaxis. An increased risk of humoral rejection is associated with female gender, elevated PRA, cytomegalovirus (CMV) seropositivity, a positive crossmatch, and prior sensitization to OKT3 (muromonab-CD3).17 Strategies to reverse humoral rejection include plasmapheresis, often in combination with IV immunoglobulin, high-dose IV corticosteroids, antithymocyte globulin (ATG), cyclophosphamide, rituximab, and mycophenolate mofetil.
Chronic Rejection
Chronic rejection is a major cause of graft loss. It presents as a slow and indolent form of ACR, in which the involvement of the humoral immune system and antibodies against the vascular endothelium appear to play a role. Persistent perivascular and interstitial inflammation is a common finding in kidney, liver, and heart transplantation. As a result of the complex interaction of multiple drugs and diseases over time, it is difficult to delineate the true nature of chronic rejection. Unlike acute rejection, chronic rejection is not reversible with any immunosuppressive agents currently available.
Kidney
Advances in the management of acute rejection during the last decade have increased the duration of functioning grafts from living and cadaveric donors by more than 70%.18 Chronic allograft nephropathy remains the most common cause of graft loss in the late posttransplantation period (>1 year). The syndrome is characterized in histological terms as interstitial fibrosis and tubular atrophy (IFTA) of unknown etiology. Structural changes are seen in as many as 50% of kidney transplantation patients within a year after transplantation and may present as early as 3 months.19 Hypertension, proteinuria, and a progressive decline in renal function represent the classic clinical triad of chronic allograft nephropathy. Factors that contribute to the development of chronic allograft nephropathy include CNI nephrotoxicity, polyomavirus infection, hypertension, donor-related factors including ischemia time and undetected kidney disease in the donor kidney, and recurrence of the primary kidney disease in the recipient.
Liver
Approximately 3% to 5% of transplant livers are affected by chronic rejection, which is characterized by an obliterative arteriopathy and the gradual loss of bile ducts, often referred to as the vanishing bile duct syndrome. Initially patients experience an asymptomatic rise in the alkaline phosphatase and α-glutamyl transpeptidase. As levels of bilirubin increase, patients become jaundiced and may experience itching.
Heart
Cardiac allograft vasculopathy, characterized by accelerated intimal thickening or development of atherosclerotic plaques, is the leading cause of graft failure and death in heart transplant recipients.20 Endothelial injury, caused by both cell-mediated and humoral responses, is the first step in the process. Vasculopathy is restricted to the transplanted allograft. Routine surveillance with coronary angiography, intravascular ultrasound, or other procedures can aid in the diagnosis of vasculopathy. Evidence of cardiac allograft vasculopathy can be seen in as many as 14% of patients within 1 year of transplantation and in as many as 50% of patients within 5 years.20 While chronic rejection of the kidney or liver allograft is generally not amenable to treatment, 3-hydroxy-3-methylglutaryl-coenzyme A (HMGCoA) reductase inhibitors and ACEIs have been used to decrease the incidence of vasculopathy in the heart allograft.20 More recently, sirolimus and everolimus have been shown to reduce the incidence and slow progression of cardiac allograft vasculopathy.20 Percutaneous transluminal coronary angioplasty and coronary artery bypass grafting have been used in severe cases of vasculopathy; these procedures, however, are limited by significantly increased mortality compared with the general population.20
TREATMENT
Immunosuppresion can be achieved with a variety of agents and the accepted regimens for most solid organs are usually comprised of two or more agents.
Desired Outcomes
Immediately following surgery, the primary goal of therapy is to prevent hyperacute and acute rejection. The high doses of immunosuppressants required to achieve this goal, if maintained long term, may result in serious complications such as nephrotoxicity, infection, thrombocytopenia, and drug-induced diabetes. Therefore, rapid dosage reductions are frequently used to minimize these effects. Transplant immunosuppression must be balanced to optimize both graft and patient survival.
General Approach to Treatment
A multidrug approach is rational from an immunomechanistic viewpoint because the many agents have overlapping and potentially synergistic mechanisms of action. Furthermore, the use of a multidrug immunosuppression regimen may allow the use of lower doses of individual agents, thus reducing the severity of dose-related adverse effects (Fig. 70-2). The protocols and individual drug regimens tend to be medical center specific. Although induction therapy may not be uniformly used, in almost every setting, patients receive IV methylprednisolone intraoperatively. Patients may also receive a descending dose of methylprednisolone over the first 5 to 7 postoperative days before beginning oral prednisone. Protocols generally combine a drug from two or three of the following classes: calcineurin inhibitors (CNIs), antimetabolites or proliferation signal inhibitors (PSIs), and corticosteroids.
FIGURE 70-2 General approach to solid-organ transplant immunosuppression. (BUN, blood urea nitrogen; CNI, calcineurin inhibitor; CSA, cyclosporine; IL2RA, interleukin-2 receptor antagonist; LFTs, liver function tests; MPA, mycophenolic acid; OKT3, muromonab-CD3; RATG, rabbit antithymocyte immunoglobulin; Scr, serum creatinine; SRL, sirolimus; TAC, tacrolimus.)
If rejection is suspected, a biopsy can be done for definitive diagnosis or the patient may be empirically treated for rejection. Empiric treatment generally involves administration of high-dose corticosteroids, usually 500 to 1,000 mg of methylprednisolone IV for one to three doses. If signs and symptoms of rejection are resolved with empiric therapy, the maintenance immunosuppressive regimen is generally modified to provide a greater level of overall immunosuppression. If rejection is confirmed by biopsy, treatment may be based on the severity of rejection with polyclonal and monoclonal antibodies being reserved for moderate-to-severe rejections or those that have not responded to a course of corticosteroids.
Induction Therapy
Induction therapy provides a high level of immunosuppression, at the time of transplantation, with or without the immediate introduction of cyclosporine or tacrolimus (see Fig. 70-2). Two perioperative immunosuppressive strategies have been predominantly utilized to achieve this goal: (a) the provision of a highly intense immunosuppression, often on the basis of patient-specific risk factors such as age and race, or (b) the use of antibody therapy to provide enough immunosuppression to delay the initiation of therapy with the nephrotoxic CNIs. The rationale for delayed CNI administration varies slightly depending on the type of transplant. In renal transplantation, the newly transplanted kidney is very susceptible to nephrotoxic injury, whereas in liver and heart transplantation, the idea is to protect patients with preexisting renal insufficiency from further insults during the perioperative period. Additionally, CNI dosage adjustment to maintain target concentrations may be difficult in the perioperative period secondary to fluctuation of GI motility and enteral intake.
Acute Rejection
The primary goal of acute rejection therapy is to minimize the intensity of the immune response and prevent irreversible injury to the allograft. The available options include (a) increasing the doses of current immunosuppressive drugs, (b) “pulse” corticosteroids with subsequent dose taper, (c) addition of another immunosuppressant indefinitely, or (d) short-term treatment with a polyclonal or monoclonal antibody. The treatment of acute rejection almost always begins with “pulse” corticosteroid therapy for several days (oral or IV). However, African American kidney transplant recipients may not respond as well to corticosteroids; thus ATG may be preferable for this patient population.21
Cytolytic agents are often reserved for those with corticosteroid-resistant rejection, signs of hemodynamic compromise (heart), or more severe rejections. Other innovative forms of therapy for persistent or intractable rejection have been investigated, including mycophenolate mofetil, tacrolimus, low-dose methotrexate, sirolimus, total lymphoid irradiation, and plasmapheresis and IV immunoglobulin. Prophylactic agents such as valganciclovir, nystatin, trimethoprim–sulfamethoxazole, H2-receptor antagonists or proton-pump inhibitors, and/or antacids may be added to minimize adverse effects associated with these intensive immunosuppression regimens.
Maintenance Therapy
The goal of maintenance immunosuppression is to prevent acute and chronic rejection while minimizing drug-related toxicity. As patients progress through the posttransplant course, the risk of acute rejection decreases, thus allowing the clinician to gradually reduce the doses of immunosuppressants or in some cases totally withdraw them over a period of 6 to 12 months. Transplant organ and type (cadaveric vs. living-donor), the degree of HLA mismatch, time after transplantation, posttransplantation complications (including the number of acute rejections), previous immunosuppressive adverse reactions, compliance, and financial considerations are among the patient-specific factors considered in individualizing maintenance immunosuppression. CNIs are generally a central component in most maintenance regimens, although CNI-free immunosuppression remains a future goal because of the significant nephrotoxicity associated with these agents. Ideally, immunosuppression should be optimized to prevent acute rejection episodes, minimize the occurrence of chronic rejection, and prevent long-term toxicities.
Calcineurin Inhibitors
Cyclosporine and tacrolimus are the two CNIs currently used for most solid-organ transplant recipients. With the exception of heart transplant recipients (69%), more than 80% of transplant recipients receive tacrolimus as part of their immunosuppressive regimen.1
Pharmacology/Mechanism of Action
CNIs block T-cell proliferation by inhibiting the production of IL-2 and other cytokines by T cells (see Fig. 70-1). Cyclosporine and tacrolimus bind to unique cytoplasmic immunophilins cyclophilin and FK-binding protein-12 (FKBP12), respectively. The drug–immunophilin complex inhibits the action of calcineurin, an enzyme that activates the nuclear factor of activated T cells, which is, in turn, responsible for the transcription of several key cytokines necessary for T-cell activity, including IL-2. IL-2 is a potent growth factor for T cells and ultimately is responsible for activation and clonal expansion.
Pharmacokinetics
The CNIs are highly lipophilic compounds, with variable but generally low bioavailability of approximately 30% (range, 5% to 60%). Unlike tacrolimus, cyclosporine depends on bile for intestinal absorption, which lends to more interpatient and intrapatient variability. Liver recipients with a T-tube for diversion of bile may thus experience incomplete and erratic absorption of cyclosporine.
Because of the significant variability in absorption of cyclosporine, peak concentrations are reached within 2 to 6 hours of oral administration. To overcome the pharmacokinetic problems of cyclosporine, a microemulsion formulation was developed. Both forms are available commercially in the United States and are referred to as “cyclosporine, USP” and “cyclosporine, USP [MODIFIED].” The two formulations are not bioequivalent and should not be used interchangeably. The microemulsion formulation is self-emulsifying and forms a microemulsion spontaneously with aqueous fluids in the GI tract, making it less dependent on bile for absorption. The result is a significantly greater rate and extent of absorption and decreased intraindividual variability in pharmacokinetic parameters. Bioavailability is enhanced owing to better dispersion and absorption and does not require bile excretion. The relative bioavailability of the microemulsion formulation is 60%. Peak concentrations are generally reached within 1.5 to 2 hours after oral administration. Tacrolimus, on the other hand, has a more predictable absorption pattern, reaching peak concentrations within 1 to 3 hours.
Following oral absorption, both cyclosporine and tacrolimus are highly protein bound. Ninety percent of cyclosporine is bound to lipoproteins in the blood. In contrast, 99% of tacrolimus is bound primarily to albumin and α1-acid glycoprotein. Cyclosporine is distributed widely into tissue and body fluids, resulting in a large and variable volume of distribution, ranging from 3 to 5 L/kg. Because of the high concentration of FKBP12 that is found in red blood cells, tacrolimus is distributed primarily in the vasculature, with a volume of distribution of 0.8 to 1.9 L/kg. Both drugs are extensively metabolized by the cytochrome P450 3A4 (CYP3A4) system in both the gut and the liver, which accounts for both the poor bioavailability and numerous drug interactions (see eChap. 6).
Efficacy
The introduction of the CNIs significantly improved the outcomes of solid-organ transplantation in terms of patient and graft survival, with 1-year graft survival improving from 75% to 87% for cadaveric grafts.18 Both cyclosporine and tacrolimus are currently approved for prophylaxis of organ rejection in kidney, liver, and heart transplantations. When compared with the standard formulation, the microemulsion formulation of cyclosporine has demonstrated equivalent or superior efficacy in kidney, liver, and heart transplantation recipients. Studies comparing tacrolimus with either formulation of cyclosporine as primary immunosuppression demonstrate equivalent efficacy between the two agents in all transplantation situations.
Monotherapy with CNIs has been described.22 The avoidance of long-term corticosteroids is the primary advantage of CNI monotherapy, whereas the primary disadvantage is the higher incidence of rejection. As a result, CNIs are rarely used as monotherapy.
Adverse Effects
Table 70-4 summarizes the adverse effects of CNIs, cyclosporine and tacrolimus, and other immunosuppressants. The nephrotoxic potential of both drugs is equal and is often related to the dose and duration of exposure. Neurotoxicity typically manifests as tremors, headache, and peripheral neuropathy; occasionally, however, seizures have been observed. Tacrolimus may be associated with an increased occurrence of neurologic complications compared with cyclosporine.
TABLE 70-4 Comparison of Common Adverse Effects of Maintenance Immunosuppressants
Cyclosporine appears to have a greater propensity to cause or worsen hypertension and hyperlipidemia compared with tacrolimus.23–26 On the other hand, hyperglycemia is more common with tacrolimus than with cyclosporine but is often reversible when doses of tacrolimus and/or corticosteroids are reduced.24 Cyclosporine is associated with cosmetic effects, such as hirsutism and gingival hyperplasia, which may be managed by converting from cyclosporine to tacrolimus or by proper hygiene in patients who cannot be switched to tacrolimus. Tacrolimus, in contrast, has been reported to cause alopecia, which is usually self-limiting and reversible.
Calcineurin Inhibitor Nephrotoxicity Two types of nephrotoxicity can occur with CNIs. Acute nephrotoxicity is frequently seen early and is dose-dependent and reversible, but chronic nephropathy is more common. Clinical manifestations of CNI nephrotoxicity include elevated serum creatinine and blood urea nitrogen levels, hyperkalemia, hyperuricemia, mild proteinuria, and a decreased fractional excretion of sodium. CNI nephrotoxicity is recognized as the leading cause of renal dysfunction following nonrenal solid-organ transplant.
The predominant mechanism for CNI nephrotoxicity is renal vasoconstriction, primarily of the afferent arteriole, resulting in increased renal vascular resistance, decreased renal blood flow by up to 40%, reduced GFR by up to 30%, and increased proximal tubular sodium reabsorption with a reduction in urinary sodium and potassium excretion. A number of other mechanisms have been implicated, including changes in the renin–angiotensin–aldosterone system, prostaglandin synthesis, nitrous oxide production, sympathetic nervous system activation, and calcium handling.27
Measures to reduce CNI nephrotoxicity include delaying administration immediately postoperatively in patients at high risk for nephrotoxicity (using alternative induction protocols including an IL-2 receptor antagonist or antilymphocyte globulin), monitoring CNI trough blood levels and reducing the CNI dosage if the vasoconstrictive effects are problematic, and avoiding other nephrotoxins (e.g., aminoglycosides, amphotericin B, and nonsteroidal antiinflammatory agents) when possible. Currently, no proven therapies consistently prevent or reverse the nephrotoxic effects of CNIs.
In patients who have received a kidney transplant, it is often difficult to differentiate CNI nephrotoxicity from renal allograft rejection. Because the clinical features of acute renal allograft rejection and CNI nephrotoxicity may overlap considerably, a renal biopsy is necessary to differentiate the two (Table 70-5). However, differentiating between chronic renal allograft rejection and CNI nephrotoxicity may be more difficult because, in addition to clinical signs and symptoms, biopsy findings may also be similar.
TABLE 70-5 Differential Diagnosis of Acute Rejection and Cyclosporine or Tacrolimus Nephrotoxicity
Drug–Drug and Drug–Food Interactions
Drug interactions occur frequently with the CNIs because they are substrates for CYP3A4 and P-glycoprotein.28,29 The most commonly administered drugs that are known to significantly alter cyclosporine and tacrolimus levels are highlighted in Table 70-6. Inhibitors of CYP3A4, such as diltiazem or erythromycin, can increase drug concentrations up to 82%, whereas drugs that induce CYP3A4 activity, such as phenytoin or rifampin, can decrease drug concentrations by 50%.43 Some have taken advantage of these interactions by routinely prescribing CYP3A4 inhibitors to reduce the dosage and cost of CNI therapy while maintaining the same therapeutic concentrations. This strategy seems more beneficial with cyclosporine than with tacrolimus.29–31 While in vitro data suggest that drugs that increase the pH of the GI tract, such as magnesium-, aluminum-, or calcium-containing antacids, sodium bicarbonate, and magnesium oxide, can cause a pH-mediated degradation of tacrolimus by physically adsorbing tacrolimus in the GI tract, this has not been borne out in clinical studies.32 Some clinicians suggest separating such compounds from tacrolimus administration by at least 2 hours to avoid any potential interaction.
TABLE 70-6 Effect of Concomitant Drug Administration on Cyclosporine, Tacrolimus, Sirolimus, and Everolimus
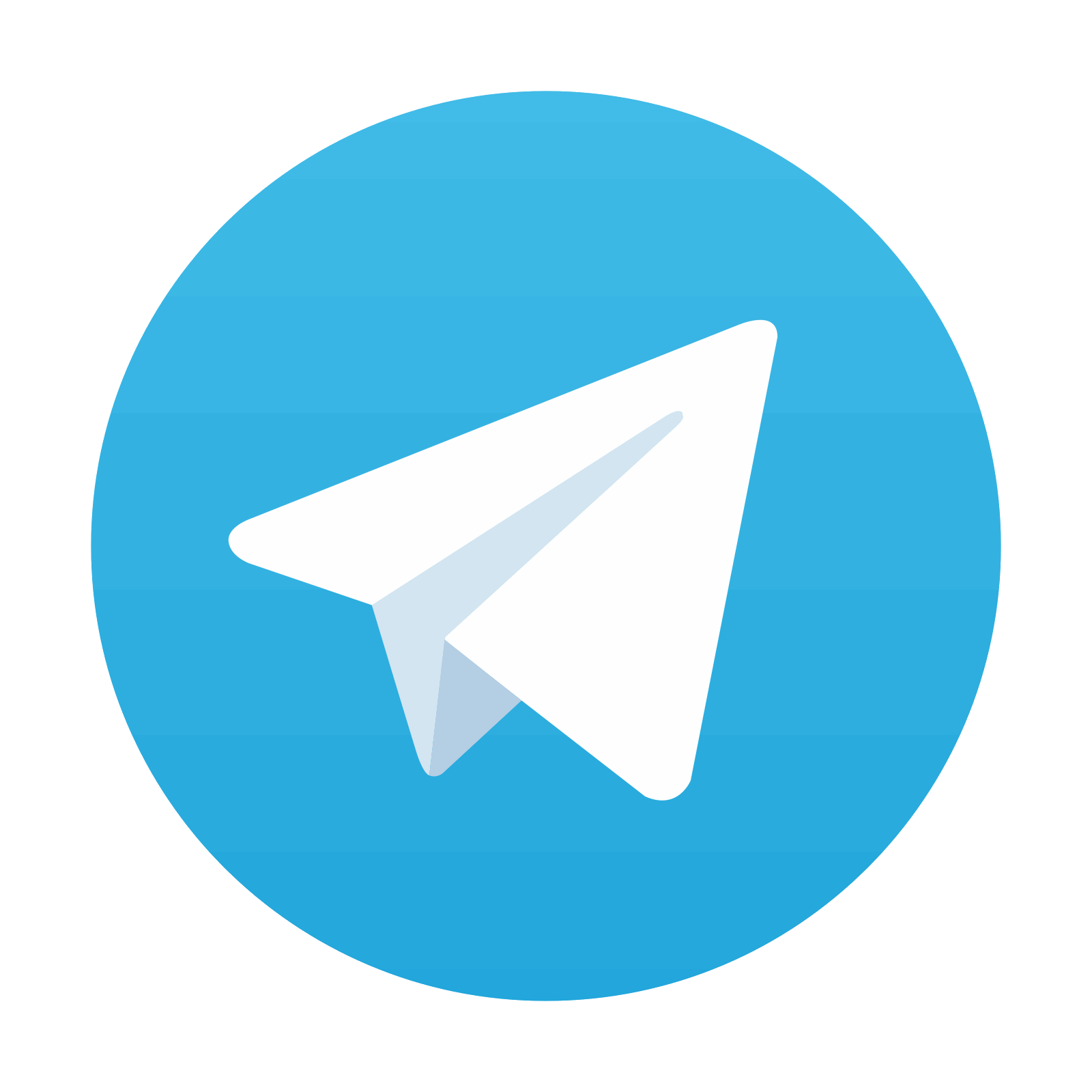